Central Neural Mechanisms that Interrelate Sensory and Affective Dimensions of Pain
Abstract
Clinical and experimental studies show serial interactions between the intensity of pain sensation, pain unpleasantness, and secondary emotions associated with reflection and future implications (i.e., suffering). These pain dimensions and their interactions relate to ascending spinal pathways and a central network of brain structures that process nociceptive information both in series and in parallel. Spinal pathways to the amygdala, hypothalamus, reticular formation, medial thalamic nuclei, and limbic cortical structures provide direct inputs to brain areas involved in arousal, bodily regulation, and hence, affect. Another major input to these same structures is from spinal pathways to somatosensory thalamic [ventroposterior lateral (VPL) and ventroposterior medial (VPM)] and cortical areas (S1 , S2 , and the posterior parietal cortex) and from these areas to cortical limbic structures (insular cortex, anterior cingulate cortex). This indirect cortico-limbic pathway integrates pain-perceptive (nociceptive) input with information about overall status of the body and self to provide cognitive mediation of pain affect. Both direct and cortico-limbic pathways converge on the same anterior cingulate cortical and subcortical structures whose function may be to establish emotional valence and response priorities. This entire brain network is under dynamic top-down (neural effects from higher-to-lower levels) modulation by brain mechanisms that are associated with anticipation, expectation, and other cognitive factors.
INTRODUCTION
Pain contains both sensory and affective (behavioral) dimensions and is often accompanied by desires to terminate, reduce, or escape its presence (1, 2) . Pain is unpleasant and contains emotional feelings because of its unique sensory qualities and because the meaning of these sensory qualities are shaped by contextual factors and by a person’s ongoing anticipations and attitudes. These contextual and cognitive factors are partly the result of the fact that pain often occurs within a situation that is threatening, such as during physical trauma or disease. Part of the affective dimension of pain is the moment-by-moment unpleasantness of pain, which consists of emotional feelings that pertain to the present or short-term future, such as annoyance, fear, or distress. Pain-unpleasantness is often, though not always, closely linked to both the intensity and unique qualities of the painful sensation. Another component of pain affect, “secondary pain affect”, includes emotional feelings directed toward long-term implications of having pain (e.g., “suffering”). This review analyzes the evidence for underlying neural mechanisms of sensory, unpleasantness, and secondary affective dimensions of pain. These mechanisms include multiple ascending spinal pathways to the brain and both serial and parallel processing in the brain. This afferent circuitry is itself controlled by top-down processing.
Psychological Interrelationships Between Sensory and Affective dImensions of Pain
Multiple factors contribute to pain unpleasantness, and several sensory attributes of pain can dispose the individual to unpleasant emotional feelings. The foremost among these is that sensations of pain are often more intense than other types of somatic sensations. In addition, psychophysical and clinical studies of pain have demonstrated that pain presents characteristics of slow adaptation (i.e., a slow or negligible decline in pain intensity during a long-duration stimulus) temporal summation (increased pain intensity with repeated stimuli whose magnitudes remains constant), spatial spread of sensation at suprathreshold levels, spatial summation, and unique sensory qualities, as implied by words such as “stinging”, “burning”, and “aching” (1, 2) . Indeed, qualities of sensations evoked by tissue-damaging stimuli or stimuli that would produce tissue damage if maintained (nociceptive stimuli), dispose us to perceive pain as invasive and intrusive for both the body and consciousness (2) . Both neural and psychological processes related to sensory qualities of pain are important causal links in the production of pain-related emotional disturbance. The persistence of these sensory qualities over time enhances unpleasantness.
Nociceptive, exteroceptive (e.g., sight and sound) and interoceptive sensory processes (e.g., startle, increased autonomic responses) may provide parallel contributions to pain affect (2) . Consistent with a neurological view of emotion mechanisms (3) , pain unpleasantness reflects the contribution of several sources, including pain sensory qualities, arousal, visceral, and somatomotor responses. Thus, these psychophysiological sources of input, in combination with an appraisal of the context in which they occur, give rise to both perceptions and feelings of what is happening to the body and self, often but not necessarily accompanied by specific thoughts. This “felt meaning” derives largely from the experience of the body and constitutes the immediate unpleasantness of pain. For Damasio, “the essence of feeling an emotion is the experience of such changes in juxtaposition to the mental images that initiated the cycle” (3) . In the case of pain, the mental image is that of a physically intrusive and qualitatively unique somatic perception.
Psychophysical studies have revealed that pain sensation and pain-unpleasantness are two distinct dimensions of pain that demonstrate reliably different relationships to nociceptive stimulus intensity and are separately influenced by various psychological factors. Psychophysical relationships of both pain sensation intensity and pain-unpleasantness ratings to heat stimuli (45–50 °C, for five seconds) are positively accelerating power functions (with exponents of about 3.0 and 3.5, respectively) (2, 4) . [Power functions are stimulus-response functions in which equal ratios of stimulus intensity give rise to equal ratios of response magnitude. The most simple example is a precisely linear function where the power function exponent is 1.0—for example, Y = aX1.0 . Exponents can be higher than 1.0, as in the case of both pain intensity and pain unpleasantness.] However, pain unpleasantness ratings are less than those of pain sensation intensity ratings in response to 45–50 °C temperatures. Thus, the ratio of pain-unpleasantness to pain sensory ratings is less than 1.0, and a larger power function exponent is obtained for unpleasantness as compared to pain sensation intensity (4) . Lower ratings of unpleasantness in comparison to sensation intensity are likely the result of assurances of safety or the brevity of the five-second stimuli. Ratios of unpleasantness ratings to sensory ratings of brief experimental pain stimuli (five seconds of applied heat, electric shock) are systematically less than 1.0, whereas those of long-duration pain stimuli (ischemia, cold) are 1.0 or greater (5) . Thus, systematic differences in ratios of affective ratings to sensory ratings of nociceptive stimulus intensity occur as a predicted consequence of simple factors, such as stimulus duration and the presence or absence of assurances. These differences provide evidence for separate dimensions of pain.
Two related experiments provide further support for the uniqueness of the two pain dimensions—pain sensation and pain-unpleasantness—and help establish the direction of causation between them (6) . For both, the left hands of subjects were immersed in a moderately painful warm-hot water bath. Hypnotic suggestions were alternately given for enhancing and then decreasing only pain unpleasantness in the first experiment, and for enhancing and then decreasing pain-sensation intensity in the second. Only reported pain-unpleasantness ratings changed in the first experiment, whereas both pain intensity and unpleasantness ratings changed in parallel in the second. These results establish the direction of causation: pain sensation occurs in series with, and is a cause of, pain unpleasantness and not vice versa (Figure 1⇓). These results are consistent with studies showing that some psychological factors selectively influence pain-unpleasantness, whereas other factors alter pain-unpleasantness in response to changes in pain sensation (2, 4) .
Interactions between pain sensation, pain unpleasantness, and secondary pain-affect. Interactions are illustrated by arrows. Neural structures likely to have a role in these dimensions are shown. Dashed arrows indicate nociceptive or endogenous physiological factors that influence pain sensation and unpleasantness. ACC, anterior cingulate cortex; PCC, posterior cingulate cortex; IC, insular cortex; HYP, hypothalamus; S1 and S2 , first and second somatosensory cortical areas; PPC, posterior parietal complex; SMA, supplementary motor area; AMYG, amygdala; PFC, prefrontal cortex; RF, reticular formation.
Unlike pain unpleasantness, secondary pain-affect is based on more elaborate reflection related to what one remembers or imagines, and includes perceived interference with one’s life, difficulties of enduring pain over time, and implications for the future (2, 4) . Pain is often experienced not only as a threat to the present state of one’s body, comfort, or activity, but also to one’s future well-being and life in general. The perceived implications that present distress holds for future well-being and functioning support the link between pain unpleasantness and secondary-pain affect. Studies of pain patients show the distinction between immediate pain-unpleasantness and secondary pain-affect, and their sequential interactions. Thus, personality traits involving neuroses exert selective enhancement of secondary pain-affect (7) , whereas age appears to selectively decrease the secondary stage of pain-related affect (8) . The sequential nature of the sensory intensity-unpleasantness-secondary affect relationship is supported by multivariate [Linear Structural Relations (LISREL)] path analyses of ratings of these pain dimensions by chronic-pain patients (9) . The remainder of this review focuses on the functional neuroanatomy and physiology of ascending spinal pathways and brain circuitry that further explain the psychological distinctions between these dimensions of pain and their interrelationships.
Pain-related Pathways and Brain Regions
The “lateral” spinothalamic tract
One principal ascending spinal pathway for pain is the “lateral” spinothalamic tract (Figure 2⇓). It receives synaptic input from primary nociceptive afferent neurons and its neurons of origin are from both lamina I and deep layers V-VI of the dorsal horn (10, 11) . Its main projection is to the ventroposterior lateral (VPL) nucleus of the thalamus and, from there, to the primary and secondary somatosensory cerebral cortical areas (S1 and S2 ), that are critical for sensory processing of pain. Thus, this entire pathway can be termed STT-VPL-S1-2 (spinothalamic tract to ventroposteriolateral nucleus of the thalamus to primary and secondary somatosensory cerebral cortical areas). Two general points can be made about this pathway: 1) its neurons of origin within the dorsal horn contain predominantly wide dynamic range (WDR) neurons and some nociceptive-specific (NS) neurons and these two types of neurons are functionally significant for processing the different dimensions and stages of pain and, 2) this “lateral” STT–VPL–S1-2 pathway is critical for sensory processing of pain as well as for pain affect, a point that will be supported by neurological and physiological evidence discussed below.
Ascending pathways, subcortical structures, and cerebral cortical structures involved in processing pain. PAG, periaqueductal grey; PB, parabrachial nucleus of the dorsolateral pons; Vmpo, ventromedial part of the posterior nuclear complex; MDvc, ventrocaudal part of the medial dorsal nucleus;VPL, ventroposterior lateral nucleus; ACC, anterior cingulate cortex; PCC, posterior cingulate cortex; HY, hypothalamus; S1 and S2 , first and second somatosensory cortical areas; PPC, posterior parietal complex; SMA, supplementary motor area; AMYG, amygdala; PF, prefrontal cortex.
Two types of neurons in STT–VPL–S1-2
It is incontrovertible that nociceptive neurons within this pathway are comprised of wide dynamic range (WDR) neurons that respond differentially over a broad range of stimulus intensity, extending from very gentle to distinctly painful levels of stimulation as well as nociceptive-specific (NS) neurons that respond nearly exclusively to nociceptive stimuli. Both types of neurons receive synaptic input from primary nociceptive afferent neurons innervating cutaneous, visceral, muscular, or other tissues, and originate within the dorsal horn of the spinal cord and medulla (in the case of the trigeminal system) (Figure 2⇑). There is considerable evidence that the large majority of neurons of STT–VPL–S1-2 are WDR neurons. The large majority (>75%) of spinothalamic and trigeminothalamic tract neurons originating from the dorsal horn and projecting to VPL and VPM are WDR (11–15) . Studies of VPL–VPM nociceptive neurons that are likely to directly receive input from this ascending pathway similarly reflect a strong preponderance of WDR neurons (16–20) . To take just two examples, Kenshalo et al. found that 89% of VPL nociceptive neurons in monkeys were WDR and that the remaining neurons were NS (19) . More recently, Lee et al. found that 87% of human VP neurons that were clearly nociceptive were WDR and the remaining ones were likely NS neurons (20) . Finally, nociceptive neurons from the somatosensory cortex (S1 ) that are likely to receive input from nociceptive neurons in VPL–VPM are predominantly WDR (21, 22) . Thus, a conservative interpretation of both anatomical and neurophysiological studies of neurons within the STT-VPL-S1-2 pathway is that they are predominantly (>75%) WDR. The functional meaning of the fact that most nociceptive neurons within STT–VPL–S1-2 are WDR is based on the capacity of these neurons to precisely encode the intensity of a nociceptive stimulus. Thus, similar to the psychophysical performance of both human and monkeys, WDR neurons within STT–VPL–S1-2 can respond differentially to a 0.2 to 0.3 °C change in stimulus intensity within a range of painful 45–51 °C skin temperatures (16, 21, 22) . NS neurons have much less of a capacity to encode these small differences (16, 21, 22) . Moreover, the stimulus-response functions of WDR neurons to this range of skin temperatures are precise positively accelerating power functions, ones that strongly resemble power functions that are obtained from human subjects who rated these same temperature stimuli (2, 12–14) . Based on the capacity of WDR to precisely encode stimulus features of nociceptive stimuli, the parallels between WDR neuron responses and human ratings of nociceptive stimuli, and the strong predominance of WDR neurons in STT–VPL–S1-2 , it is clear that both WDR neurons and STT–VPL–S1-2 are critical for the sensory dimension of pain (2, 11–22) .
Other ascending pathways
Several other ascending spinal pathways are important for pain (Figure 2⇑). An interesting difference between some of these pathways and STT–VPL–S1-2 is that unlike the latter, the former often reflect a preponderance of NS neurons. For example, Bernard and colleagues have characterized two novel pathways for pain, termed the spino-parabrachio-amygdaloid and the spino-parabrachio-hypothalamic pathway (23, 24) . The most remarkable and consistent feature of nociceptive neurons within these two pathways is that they consist exclusively of neurons responsive to only nociceptive stimulation—that is, NS neurons, and that this pattern is nearly the reverse of STT–VPL–S1-2 . Consistent with this unique feature is the finding that spinal cord neurons of origin of these pathways are exclusively within layer I of the dorsal horn—a region dominated by NS neurons (15, 23, 24) . Neurons within these pathways appear to encode nociceptive stimulus intensity with some degree of precision, though somewhat less than that of WDR neurons. These two pathways offer a striking comparison with STT–VPL–S1-2 because of their very different proportions of WDR and NS neurons and because their central targets appear functionally very different. Whereas STT–VPL–S1 is likely to be heavily involved in sensory-perceptual aspects of pain, the two pathways to the amygdala and hypothalamus are more likely to be heavily involved in autonomic processes and behaviors related to fear and defense. The central nucleus of the amygdala has been strongly implicated in fear, emotional memory and behavior, and autonomic and somatomotor responses to threatening stimuli (23, 24) . Various hypothalamic nuclei have also been implicated in these functions. Based largely on their central targets, these pathways are very likely to strongly participate in the affective dimension of pain, particularly the initial affect associated with acute pain. There also is a spinohypothalamic pathway that contains neurons like that of STT–VPL–S1-2 and in fact many STT neurons project to both thalamus and hypothalamus (25) .
To a similar extent, spinothalamic tract pathways to more ventral and medial thalamic nuclei, such as VMpo (ventromedial portion of posterior nuclear complex) and MDvc (ventrocaudal portion of the mediodorsal nucleus) appear to have much greater proportions of NS neurons (26) . A study of nociceptive neurons within these thalamic nuclei found that, unlike neurons in VPM, they are highly modifiable by attentional and motivational state (16) . Based on these differences in response characteristics, VMpo and MDvc neurons may be somewhat more directly involved in affective motivational components of pain, whereas neurons within STT–VPL–S1 -2 participate in pain affect as a result of their role in sensory features of pain.
Brain pathways and regions interrelating sensory and affective dimensions of pain
The foregoing descriptions suggest the existence of several functionally different ascending pathways for pain: 1) a STT–VPL–S1-2 pathway containing mostly WDR neurons important for encoding the capacity to recognize the sensory intensity and sensory qualitative features of pain and, 2) other pathways containing mostly NS neurons important for several aspects of pain, including immediate affective-motivational responses, autonomic and somatomotor activation, and possibly pain sensation. These different pathways are functionally diverse and they are interpreted as contributing to both pain sensory features and pain-related emotions in different ways. These pathways include both series and parallel circuitry (Figure 2⇑).
The serial interconnections are established by the fact that the STT–VPL–S1-2 pathway is anatomically interconnected with a ventrally directed cortico-limbic somatosensory pathway that integrates somatosensory input with other sensory modalities such as vision and audition and with learning and memory (27) . This pathway proceeds from S1 –S2 to posterior parietal cortical areas and to the insular cortex (IC), and from IC to amygdala, the perirhinal cortex, and hippocampus (27) . This cortico-limbic pathway is critical for pain affect because damage to large parts of insular cortex has been found among patients with pain asymbolia (28, 29) . Patients with this condition do not display behavior indicative of threat or intrusion in response to painful stimuli despite their capacity to still appreciate the sensory qualities of painful stimuli. A recent study by Greenspan et al. showed that patients harboring only anterior insular cortical lesions had entirely normal thermal and mechanical pain thresholds yet had longer tolerance times when tested with painful ice-water (30) . Presumably this latter test reflected the affective dimension of pain to a greater extent than sensory discrimination. Similar results have been found for non-human primates. Focal damage to S-2/7b (secondary somatosensory and adjacent infraparietal cortical areas) in the monkey results in an absence of escape responses to painful temperatures despite preservation of the ability to detect the offset of noxious thermal stimuli (31) . An area that receives input from areas S-2/7b is insular cortex (32) , the same area that is associated with pain asymbolia in humans. This serial corticolimbic somatosensory pathway ultimately converges on the same limbic and subcortical structures that may be more directly accessed by other ascending spinal pathways. The latter reflect parallel processing. This dual convergence may be related to a mechanism whereby multiple neural sources, including somatosensory cortices S1 and S2 , contribute to pain affect. Therefore, this dual convergence reflects both parallel and serial processing.
Electrophysiological recordings of neurons of infraparietal cortical area S-2/7b neurons in monkeys help explain how this mechanism may be involved in pain affect (33, 34) . Neurons in this region responded to nociceptive stimuli (44–47 °C) and yet were enhanced by antecedent or concurrent visual stimuli (33) . However, this enhancement only occurred if the target location or direction of motion within the visual receptive field was spatially aligned with the cutaneous receptive field. The enhancement was much greater for mild nociceptive stimuli (44–45 °C) than for stronger stimuli (47 °C) (33) . The neural organization of this region of the posterior parietal cortex appears to be that of integrating nociceptive inputs with other sensory inputs in a manner that conveys information about the overall degree of threat presented to an organism. This integration is especially critical at the low end of the nociceptive stimulus range, wherein an organism must make a relevant decision about the extent of threat presented by an object. The processing of pain requires an evaluation of sensation in relationship to its overall context—an evaluation that may help link sensation with affect. This function would require integration of somatosensory input with other sensory modalities and with memory.
The contribution of somatosensory cortices (S1 and possibly S2) to pain sensation and pain affect
This corticolimbic serial mechanism directly implies that somatosensory cortices are involved in pain affect, an implication consistent with the previously described psychophysical studies in which pain unpleasantness was shown to be in series with and the result of pain sensation intensity (2, 6) . This explanation requires that somatosensory cortices directly function in the sensory aspects of pain and it predicts that lesions within STT–VPL–S1-2 would result in deficits in both pain sensation and affect. The neurological evidence for the involvement of STT–VPL–S1-2 in sensory dimensions of pain is based on several lines of evidence. First, considerable evidence now exists for the involvement of S1 in sensory features of pain. Lesions within S1 produce deficits in ability to detect sensory features of pain in both monkeys (35) and humans (30, 36, 37) . These deficits are sometimes small, particularly when the lesions of S1 are restricted to only a portion of S1 (35) . Second, several brain imaging studies have now shown that neural activity in S1 (measured by regional cerebral blood flow) closely co-varies with temporal and/or intensive aspects of pain sensations (38, 39) . Although not all brain imaging studies of pain show activation within S1 , negative results are likely the result of technical difficulties in neuroimaging activity in this portion of parietal cortex (40) . Third, as described above, nociceptive neurons definitely exist within S1 in several mammalian species, including primates (2, 21, 22) and their response characteristics parallel psychophysical characteristics of pain in human and non-human primates (2, 21, 41, 42) . Thus, the involvement of somatosensory cortices in sensory-discriminative aspects of pain, a requirement for the proposed corticolimbic serial mechanism, stands on firm ground.
The involvement of STT–VPL–S1-2 in the affective dimension of pain is less well established, mainly because classical models of pain processing simply assume that sensory and affective dimensions of pain are processed by completely parallel neural systems (1) . Nevertheless, several neurological studies support a role for STT–VPL–S1-2 in the affective dimension of pain. Perhaps the most recent and noteworthy study is that of Ploner et al. (43) . Their study involved a patient with an extensive lesion of the right S1 and S2 somatosensory cortex. The patient had a normal laser-evoked pain threshold on his right hand that was contralateral to his left intact cerebral hemisphere. However, neither pain sensation nor pain unpleasantness could be evoked from stimulation of the left hand (contralateral to his damaged somatosensory cortex) until the stimulus level was well above that of pain threshold of his right hand. Thus, the deficit included both sensory and affective dimensions. Interestingly, the only response obtained at the higher stimulus level was a vague feeling of unpleasantness in his left shoulder and arm, a feeling that was devoid of the usual sensory qualities of pain (43) . Other studies contain results that are similar in principle to those found by Ploner et al. Head and Holmes found patients with lesions of the lateral somatosensory thalamus (one of these was verified histologically to be within VPL) to have deficits in pain appreciation, including unpleasantness, throughout the low to moderate range of nociceptive stimulus intensities (44) . Like Ploner’s patient, they developed vague feelings of unpleasantness or “pain-related feelings” when the stimulus intensity was raised to levels well above normal pain threshold. The interruption of the pathway to the VPL or the S1 –S2 cortex produces deficits in appreciation of sensory qualities and intensities associated with pain and pain-related unpleasantness throughout the lower to moderate levels of nociceptive stimulation. When the stimulus intensity reaches a high enough level, other pathways contribute in a parallel manner to unpleasantness, often combined with the relative absence of the ability to recognize sensory features normally present in pain experience. Using direct scaling methods, Greenspan et al. found that a patient with damage to her lateral thalamus had distinct deficits in both pain sensation and pain unpleasantness throughout the low to moderate range of nociceptive stimulus intensities (12) .
Both pain sensations and pain affect are disrupted by STT–VPL–S1-2 lesions because this pathway makes serial interconnections to cortico-limbic structures involved in pain-related affect (27, 32) . This anatomical connection is consistent with psychological studies that show serial interactions between pain sensation intensity and pain unpleasantness (2, 6) . Thus, a major contribution to pain affect is provided from STT–VPL–S1-2 . This pathway projects to brain areas that integrate nociceptive input with contextual information and memory to provide cognitive mediation of pain affect. The involvement of STT–VPL–S1-2 in both sensory and affective dimensions of pain is in direct opposition to a classic parallel processing view of the spinothalamic tract.
The pivotal role of the anterior cingulate cortex (ACC) in pain affect
As illustrated in Figure 2⇑, the anterior cingulate cortex (ACC) receives anatomical projections from several sources, including the insular cortex. ACC, in turn, is part of the brain’s attentional and motivational network because it projects to prefrontal cortical areas involved in executive functions and to the supplementary motor area involved in response selection (46–48) . Parts of the ACC are consistently activated during pain and in fact the ACC is the most consistent brain region activated in brain imaging studies of pain (2, 49) . Brain imaging studies show that ACC is more directly involved in pain affect than in appreciating the sensory qualities of pain. A positron emission tomography (PET) study used hypnotic suggestions to selectively increase or decrease unpleasantness ratings of experimental pain (50) . Significant pain-related activation occurred in the somatosensory area I, (S1), ACC area 24, and IC during control conditions. Hypnotic suggestions resulted in much larger unpleasantness ratings during the “high unpleasantness” condition as compared to the “low unpleasantness” condition, with no differences in pain sensation ratings. Consistent with these changes, activity in the posterior sector of ACC area 24 was much greater in the high as compared to the low unpleasantness condition yet no differences occurred for S1 (Figure 3⇓). A separate regression analysis, controlling for factors such as pain sensation intensity ratings, showed that pain unpleasantness ratings were significantly associated with activity in the posterior sector of ACC area 24 (R = 0.55, p < 0.001). A subsequent study that used hypnosis to modify pain sensation intensity showed corresponding changes in S1 cortex (51) .
Pain affect modulation. Left side: Hypnotic suggestions for increased (High) and decreased (Low) pain unpleasantness produce corresponding increases (left sagittal scan) or decreases (right sagittal scan) in neural activity (measured by rCBF) in anterior cingulate cortex (ACC) but no change in S-1 somatosensory cortex (S1), similar to respective effects on subjects unpleasantness and sensation intensity ratings. Right side: Correlation between ACC activity and unpleasantness ratings after pain intensity and inter-subject related variances are removed from both factors (ANCOVA).
A second PET study confirmed the results of Rainville et al. (50) by using a different experimental approach to induce selective variation in pain unpleasantness (52) . Using noxious heat and four successive experimental pain trials, Tolle et al. found that lower ratios of pain unpleasantness to pain sensation intensity were evoked on the first two trials and higher ratios on the second (52) . Regression analysis showed that although several brain structures were activated during pain, only pain unpleasantness was encoded in ACC area 24 (52) . Both studies suggest that ACC, rather than the somatosensory cortical areas, may be more proximal to the production of pain affect (50, 52) ; however, the latter also contribute to pain-affect by virtue of their interconnections to IC and, thus, to ACC.
The proximal causal role of ACC in the affective component of pain is supported by an experiment in which neurons of the rostral ACC were destroyed in rodents (53) . Using the hind-paw formalin model, Johansen et al. measured a learned-behavior place-conditioning paradigm that directly reflects the affective component of pain as well as formalin-induced nociceptive behaviors (i.e., paw lifting, licking, and flinching) that reflect the intensity and localization of the nociceptive stimulus (53) . Destruction of neurons within the rostral but not caudal ACC reduced formalin-induced conditioned place avoidance without reducing acute pain-related behaviors. Their results provide evidence that neurons in the ACC are necessary for some aspects of the affective dimension of pain, particularly those related to behavioral responses associated with avoiding or escaping the nociceptive stimulus.
The functional role of ACC and related limbic structures could be further clarified by consideration of how they fit into the general circuitry associated with pain processing. The general ways in which multiple ascending pathways and brain regions could participate in the various components of pain affect are shown in Figure 2⇑. Nociceptive pathways originating from the spinal cord dorsal horn directly activate brain structures involved in rudimentary aspects of autonomic system activation, escape, motoric orientation, arousal, and fear (2, 11–22, 49) . These include medullary and midbrain reticular formation nuclei, deep layers of the superior colliculus, central grey, amygdala, hypothalamus, and specific medial thalamic nuclei. Activation of these structures likely occurs during the early phase of pain, wherein fear, defensive behavior, and autonomic responses would take place. These responses would occur somewhat automatically and involve a minimum amount of cognition.
Posterior parietal cortical areas that integrate somatosensory input with other sensory modalities and with learning and memory project to the same cortical and subcortical limbic structures (ACC, IC, and amygdala) that receive direct input from spinal pain pathways (2, 32, 47, 48) . Convergence at the level of ACC would be consistent with a mechanism in which somatic perceptual and cognitive features of pain would be integrated with attentional and rudimentary emotion mechanisms. Based on neurological evidence (46) , the ACC may have a complex pivotal role in interrelating attentional and evaluative functions with that of establishing emotional valence and response priorities. Response priorities would be closely related to pre-motor functions that are integrally related to motivation and emotions and may be associated with immediate efforts to cope with, escape, or avoid the pain and pain-evoking situation. In this view, cortical areas controlling sensory, attentional, pre-motor, and affective functions of pain are largely in series, an interpretation supported by both psychological (2, 6) and brain-imaging studies (50–52) described above.
Response priorities change over an extended period of time. Pain unpleasantness endured over time engages prefrontal cortical areas involved in reflection and rumination over the future implications of a persistent pain condition. The ACC may serve this function by coordinating somatosensory features of pain with prefrontal cerebral mechanisms involved in attaching significance and long term implications to pain, a function associated with secondary-pain affect. Thus, ACC may be a region that coordinates inputs from parietal areas involved in perception of bodily threat with frontal cortical areas involved in plans and response priorities for pain-related behavior. Both functions would help explain observations on patients with prefrontal lobotomy and patients with pain asymbolia as described above. The former have deficits in spontaneous concern or rumination about their pain but can experience the immediate threat of pain once it is brought to their attention (37, 54) . In contrast, asymbolia patients appear incapable of perceiving the threat of nociceptive stimuli under any circumstances (28, 29, 55) .
A Parallel–Serial Model of Pain Affect
This view of pain-affect mechanisms is that of a central network of brain structures and pathways that contains both serial and parallel connections (Figure 2⇑). Direct spinal inputs to lower brainstem and limbic structures may contribute to rudimentary aspects of pain affect, such as arousal, autonomic, and somatomotor activation. Spinothalamic pathways to medial thalamic nuclei provide direct input regions involved in monitoring the overall state of the body (IC), directing attention (ACC), and assigning response priorities (ACC). However, ACC receives a major serial input from a ventrally directed somatosensory-limbic pathway that contributes varying degrees of cognitive evaluation to pain affect (Figure 2⇑). This pathway is consistent with evidence that pain-unpleasantness is in series with sensory aspects of pain and is cognitively mediated (Figure 1⇑). Additional parallel contributions to pain affect could include arousal and consequences of autonomic–somatomotor activation and would be consistent with both psychological (Figure 1⇑) and neuroanatomical (Figure 2⇑) evidence. The ACC is a pivotal area that receives multiple inputs and is more closely associated with pain-unpleasantness than are cortical structures and ascending pathways that project there. Secondary pain-affect is sustained by pain unpleasantness and may depend on ACC–prefrontal cortical interactions that add further cognitive evaluation to emotions associated with pain.
Top-Down Modulation of Pain Sensation and Pain Affect by Cognitive Factors
The schematic illustration of Figure 2⇑ refers to ascending pathways and afferent circuitry involved in pain processing. Absent from this model are neural circuits that provide top-down modulation of pain experience. Central neural mechanisms associated with such phenomena as placebo and nocebo manipulations (giving inert agents with suggestions for respectively reducing or enhancing symptoms), hypnotic suggestion, attention, distraction, and even ongoing emotions are now thought to modulate pain by decreasing or increasing neural activity within many of the brain structures shown in Figure 2⇑. This modulation includes endogenous pain-inhibitory and pain-facilitation pathways that descend to spinal dorsal horn, the origin of ascending spinal pathways for pain (56) . Recent brain imaging studies have begun to reveal the cortical areas involved in pain modulation. Zubieta et al. examined the function of the endogenous opioid system and μ -opioid receptors in the brains of normal human subjects undergoing sustained experimentally induced pain (57) . Sustained pain resulted in regional release of endogenous opioids interacting with μ -opioid receptors in a number of cortical and subcortical brain regions. Receptor activation was associated with reductions in the sensory and affective ratings of pain. This study is nicely complemented by a study by Petrovic et al. that demonstrate that placebo and opioid analgesia share a common neuronal brain network (58) . Using PET, they confirmed that both opioid and placebo analgesia are associated with increased activity in the rostral ACC (rACC). They also observed a covariation between rACC activity and brainstem activity during both opioid and placebo analgesia, but not during the baseline pain condition.
The modulation of pain by endogenous opioid systems by cortical and subcortical networks is consistent with other types of brain imaging experiments that show that anticipation of pain affects cortical nociceptive regions. Interestingly, these brain regions are the same ones that are directly activated during pain itself. Porro and colleagues used functional magnetic resonance imaging (fMRI) to study changes of activity of cortical nociceptive brain regions in healthy volunteers while they expected the nociceptive stimulation of one foot, which could or could be not stimulated with painful subcutaneous injection of ascorbic acid (59) . Mean fMRI signal intensity increased over baseline values during both actual nociceptive stimulation and anticipation of pain in the absence of stimulation. These increases occurred precisely in the same cortical areas, including contralateral S1 , bilaterally in ACC, insular cortex, and medial prefrontal cortex (see Figure 2⇑ for locations). In all of these areas, changes in signal intensity during anticipation of pain were of the same direction as during pain itself but about 30–40 % as large. Their results provide evidence for top-down mechanisms involved in sensory and affective dimensions of pain even in the absence of actual nociceptive stimulation. Thus, the cortical networks shown in Figure 2⇑ may be directly influenced by cognitive factors. These are likely to include not only anticipation of the presence of pain, but also anticipation of its reduction, as in the case of placebo or hypnotic analgesia. The modulatory mechanisms are likely to be diverse, extending from those which are confined only to neural interactions within the brain to those involving activation of brain-to-spinal cord circuits long known to potently reduce or enhance pain, as represented in Figure 4⇓ (56) .
Afferent pathways for pain and descending brain-to-spinal cord modulatory pathways. Left: ascending pathways for pain, including receptors and primary afferent neurons, ascending spinothalamic tract, and thalamocortical pathways to somatosensory cortex. Right: descending pain modulatory pathways. This system originates in cerebral cortical areas, including amygdala, and projects to central grey. The latter, in turn projects to cells of the rostroventral medulla, which in turn project to the dorsal horn. Both inhibitory and facilitatory effects are exerted at the level of the dorsal horn. Thus, there is bi-directional control of nociceptive transmission at this level.
Concluding Remarks
The combination of anatomical, neurological, and neurophysiological approaches to understanding the brain mechanisms underlying sensory and affective dimensions of pain has led to a vastly improved ability to answer questions that only ten years ago were relatively impenetrable. In particular, studies that combine brain imaging with psychophysical methods and sophisticated experimental designs have led to the possibility of understanding complex mechanisms by which sensory and affective dimensions of pain are interrelated and how these dimensions can be modulated by cognitive factors. The brain networks for these mechanisms are extensive and involve both serial and parallel circuitry that is itself under dynamic control from several brain regions. This view is radically different from classic models that present central pain pathways as two parallel lines, one for affect and the other for sensation or that propose one or two discrete pain centers of the brain. Challenges and puzzles remain, but the old questions now seem much more answerable. We are witnessing at the beginning of the new century a merging of physiology, molecular biology, and psychology of pain, with profound consequences for the field of neuroscience and the care of pain patients.
- © American Society for Pharmacology and Experimental Theraputics 2002
References
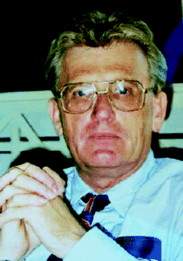
Donald D. Price, PhD , is a Professor in the Departments of Neuroscience and Oral and Maxillofacial Surgery in the McKnight Brain Institute at the University of Florida. His research interests span neuroscience and psychology in addressing questions about neural and psychological mechanisms of pain and emotions. His most recent book on this topic is “Psychological Mechanisms of Pain and Analgesia”, IASP Press, Seattle, 1999. Send correspondence to DDP at the Department of Oral and Maxillofacial Surgery, Health Science Center, PO Box 100416, University of Florida, Gainesville, FL, 32610-0416
E-mail dprice{at}dental.ufl.edu