“Gene Targeting in the Rat? Cut it out!”
Knockout model organisms have proven to be valuable genetic tools for the study of gene function. In mammals, the ability to specifically target genomic sequences to disrupt gene function has traditionally been limited to the mouse. The technology requires homologous recombination (HR)-driven gene/exon targeting in pluripotent embryonic stem (ES) cells. ES cells of successfully manipulated clones are injected into blastocysts to produce chi-maeric mice that are outcrossed (to a more robust breeding strain) to accomplish germline transmission of the recombination-engineered (i.e., “recombineered”) transgene (1). This method is time-consuming and applicable to less than a handful of specific inbred mouse strains. Nevertheless, it still is the standard for producing mouse gene knockouts and conditional alleles and has contributed tremendously to our understanding of mammalian gene function.
The laboratory rat has a long and rich history of use in biomedical research (2). More recently, genetics- and genomics-oriented researchers have explored rat models for certain aspects of human health and disease, such as neurobiology, metabolic and cardiovascular disease, oncology, and toxicological (drug) testing, in order to discover novel human disease genes and pathways (3). Several genome manipulation technologies have emerged in the last decade; however, the production of knockout rats via HR in pluripotent ES cells has not been accomplished yet. The biggest hurdle has been the establishment of germline-competent pluri-potent rat ES cells [recently reviewed by Voigt and Serikawa (4)]. Nonetheless, a complex experimental path with contributions from multiple laboratories has recently led to the successful derivation and cultivation of germline-competent pluripotent rat ES cells from blastocysts (5, 6). In addition, similar culturing conditions appear to sustain the pluripotency of an induced pluripotent stem (iPS) cell line recently obtained from reprogrammed rat liver cells (7). These advances provide clear potential for ES cell–based gene targeting strategies in the rat, comparable to what has been available in the mouse for over two decades.
As the rat was gaining momentum as a genetic model organism, alternative routes to germline gene targeting were being explored (Figure 1), such as lentiviral transgenesis (8) in spermatogonial stem cells (SSC) (9) and somatic cell nuclear transfer (SCNT) (10), with the intention of ultimately targeting a gene by HR, but again none of these strategies has resulted in knockout rats. Other genome manipulation strategies to interfere with gene function have been successfully implemented (Figure 1), such as traditional transgenesis [reviewed by Tesson (11)], RNA-interference-mediated gene silencing (12), and two target-selected gene knockout technologies, namely ENU mutagenesis (13, 14) and transposon-mediated gene-trapping (15, 16).
Target-selected screening of ENU-mutagenized rat libraries has become routinely available and has yielded hundreds of putative mutations with potential consequences to gene function. The establishment of a cryopreserved archive of mutant sperm, in combination with intracytoplasmic sperm injection (ICSI) for recovery of a mutant rat, requires the screening of numerous target genes for rare, induced deleterious variants (17). Characterization of several ENU mutagenesis-induced rat knockouts have already provided novel insight in mammalian gene function in certain aspects of human health and disease for which the laboratory rat is a suitable model system, such as neurobiology (18) and tumor biology (19, 20). Because ENU mutagenesis induces random point mutations, an advantage of the method is the potential identification of multiple alleles for the same gene. A knockout allele can only be guaranteed, however, if the induced point mutation generates a premature stop codon in the coding region. On the other hand, insertional mutagenesis using, for example, the Sleeping Beauty transposon system1 yields a lower mutation rate, but the advantage of this method is that the allele generated––when the transposon jumps out of the trapped gene––is generally disruptive, resulting in a gene knockout. Although both technologies have successfully produced knockout alleles of target genes, the random nature of the mutagenesis necessitates screening large animal cohorts.
Recently, the development of zinc-finger nucleases (ZFNs) has provided the opportunity to create targeted double-strand breaks (DSB) at specific sites in the genome, such as an exon of a target gene. ZFNs are proteins that fuse the nucleolytic cleavage domain of the Fok1 restriction enzyme to a target-specific DNA recognition domain (21). The recognition domain consists of three-to-six zinc finger motifs that are selected and validated to recognize optimally the target sequence (22). The site-specific ZFNs lie apposed across the sequence-specific DNA site and the Fok1 domain of each ZFN creates a blunt DSB. Because the Fok1 domain is not very precise, it will effect DNA scission at differing sites, giving rise to deletions of various sizes. The error-prone non-homologous end joining (NHEJ) part of the DSB repair system closes the gap. If directed towards an exon of a target gene, this process will often result in a nonsense mutation, creating a knockout allele. A major advantage of this technology is that it works in a wide variety of cell types, including one-cell stage embryos, which opens the opportunity to use it as a knockout tool in models organisms lacking pluripotent ES cells, such as Drosophila (23) and zebrafish (22).
Recently, Geurts and colleagues reported the application of the ZFN technology via microinjection into rat embryos to generate targeted knockouts (24). Some ZFNs were designed to target Gfp in a Gfp-transgenic rat line; other ZFNs were designed to target the endogenous rat genes IgM and Rab38. Several ZFN candidates per target were assembled using PCR, tested in a yeast proxy system, and validated in cultured rat cells. Optimal ZFNs were injected as part of a linearized expression vector into pronuclei or as in vitro transcribed mRNA into pronuclei or cytoplasm of one-cell stage embryos. Successfully injected embryos were transferred to the oviducts of pseudopregnant females to complete in utero development. The resulting founder animals were tested for presence of the targeted gene disruption using the Surveyor nuclease assay, a mutation detection assay based on enzymatic heteroduplex cleavage (25). Of the 295 founders screened, thirty-five harbored a deletion in the target gene, corresponding to an overall allele targeting efficiency of 12%. Target site disruption occurred in founders of both inbred and outbred strains, although comparative strain efficiencies are yet to be determined. Strikingly, one of the founders appeared to have both alleles targeted, directly resulting in a homozygous knockout animal.
A possible complication of injecting a linearized plasmid into an embryo is random genome integration with functional consequences to the site of integration. The report addresses this by PCR screening five founders animals, injected with the greatest plasmid concentration. None of these tests appeared to produce a band indicative of unwanted ZFN plasmid integration, suggesting that ZFN targeting is mediated by transient expression from an extrachromosomal transgene in the pronucleus. Another serious complication could be the induction of off-target DSBs, resulting in more DNA damage than originally anticipated. Off-target site cleavage by ZFNs has previously been estimated (22, 26). A bio-informatics tool was developed to predict the most likely putative off-target sites and applied to the rat genome by Geurts et al. (24). By monitoring twenty predicted off-target sites in five founder animals using the Surveyor assay, two sites in multiple animals were shown to have unusual activity, which could be explained by the presence of a single nucleotide polymorphism (SNP) and not by an induced deletion as the result of off-target ZFN activity. Although unlikely, off-target site ZFN activity may occur beyond the predicted sites assayed, but only sequencing of the entire genome of founder animals would reveal such events.
Proven experimentally sound and highly efficient, the ZFN technology is likely to become the standard for targeted knockout rat production. A point of debate is whether the ZFN technology is also suitable for targeted-genome engineering by gene replacement in the rat germline. Gene replacement by HR in mouse ES cells has facilitated tremendous versatility in the application of functional alterations of target genes, for example tissue-specific or -inducible (conditional) knockout alleles [reviewed by Capecchi (27)]. As mentioned above, pluripotent rat ES cells have recently been produced; however, it has not been proven that these are able to withstand the cellular stress of genetic manipulation procedures.
An attractive ES cell-independent alternative employing the ZFN technology has recently been reported in Drosophila. Simple coinjection of a linearized construct for HR-directed repair after the ZFN-induced DSB does not yield many founders with the target properly replaced, probably because NHEJ occurs more frequently. However, in a NHEJ-defective genetic background (embryos lacking lig4, the NHEJ-essential gene DNA ligase IV) the efficiency of replaced targets mediated by the intact HR repair pathway, appeared to be comparable (up to 10%) to the knockout efficiency using the ZFN technology in wild-type embryos (28). One major drawback for implementation of this strategy in the mammalian model systems is that homozygous mouse knockouts for lig4 are lethal in the embryo stage (29). Mouse knockouts of other components of the NHEJ pathway are similarly embryonically lethal or lead to severe combined immunodeficiency (SCID)-related syndromes (30). This route of targeted gene replacement may become applicable if the NHEJ pathway could be suppressed temporarily in one-cell stage embryos without disrupting developmental processes via increased DNA damage. For example, transient expression of short interfering RNAs (siRNAs) directed against certain components of the NHEJ pathway may restrain the NHEJ pathway in a manner long and robust enough to favor HR-mediated DSB repair for integration of the coinjected construct.
The report by Geurts and colleagues describes the implementation of an efficient targeted gene knockout approach for the rat, employing the ZFN technology in one-cell stage embryos (24). The approach is independent of pluripotent ES cells. If only a gene-knockout model is needed, this approach would be favored. The logical next step would be to produce targeted gene replacement rat models. As various routes can now be explored, such as classical HR-based methods in pluripotent ES/iPS cells, or ZFN-based methods in one-cell stage embryos or pluripotent ES/iPS cells, we anticipate this technology to become available for the rat in the near future. Targeted gene disruptions and soon targeted genome engineering by gene replacement will advance our knowledge of the contribution of genes and pathways to the human diseases mimicked in selectively bred rat models.
Schematic overview of the development of rat genome manipulation strategies and targeted gene modifications in the rat germline. The timelines start in the early 1990s with the development of traditional transgenesis by pronuclear microinjection in zygotes. The majority of advances have been made in the last decade. Importantly, targeted-gene knockout rats have been recently produced by Geurts et al. via the ZFN technology (24). Homologous recombination-based gene replacement models, however, have not been produced. With the steady development of these technologies, HR-based gene replacement rat models are anticipated in the near future. ENU, n-ethyl-n-nitrosourea; RNAi, RNA interference; ZFN, Zinc-finger nucleases; SCNT, Somatic cell nuclear transfer; SSC, Spermatogonial stem cells; ES cells, Embryonic stem cells; iPS cells, induced Pluripotent stem cells; HR, Homologous recombination.
Footnotes
-
↵1 The Sleeping Beauty transposon system inserts a gene into a human chromosome without the need of a viral vector (www.discoverygenomics.net/sbts.html).
- Copyright © 2009
References
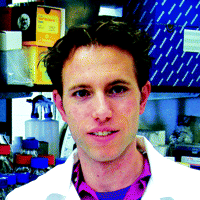
Bart M. G. Smits, PhD, obtained his doctoral degree at the Hubrecht Institute for Developmental Biology and Stem Cell Research (Utrecht, The Netherlands). In the lab of Prof. Edwin Cuppen, he worked on the development of rat gene knockout models via ENU mutagenesis. Currently, he is a postdoctoral fellow at the McArdle Lab for Cancer Research, University of Wisconsin–Madison, focusing on the characterization of low-penetrance breast cancer alleles by utilizing genetic rat models and human cell-based models.
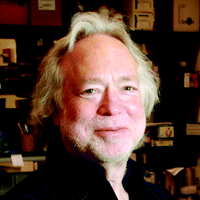
Michael N. Gould, PhD, obtained his doctoral degree in Radiological Sciences at the University of Wisconsin–Madison, where he joined the faculty following a brief postdoc at Argonne National Laboratory. Currently, he is Professor of Oncology at the McArdle Lab for Cancer Research, where his lab has been focusing on employing genetic rat models to study the molecular biology of mammary carcinogenesis. His lab has broad experience in generating traditional transgenic rat models. In 2003, his lab reported on the first gene knockout rat models, produced via ENU mutagenesis. E-mail gould{at}oncology.wisc.edu; fax 608-262-2824.