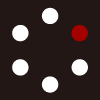
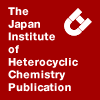
HETEROCYCLES
An International Journal for Reviews and Communications in Heterocyclic ChemistryWeb Edition ISSN: 1881-0942
Published online by The Japan Institute of Heterocyclic Chemistry
e-Journal
Full Text HTML
Received, 22nd May, 2008, Accepted, 8th July, 2008, Published online, 10th July, 2008.
DOI: 10.3987/COM-08-S(F)19
■ Catalytic Asymmetric Intramolecular Allylation of Aldehyde
Tomohiro Sato and Kiyoshi Tomioka*
Graduate School of Pharmaceutical Sciences, Kyoto University, 46-29 Yoshida-shimoadachi-machi, Sakyo-ku, Kyoto 606-8501, Japan
Abstract
The intramolecular asymmetric allylation of aldehydes was catalyzed by a combination of pyrrolidine derivatives and Pd(0) to give 5-exo-cyclized aldehyde with fair ee in good yield.INTRODUCTION
Chiral amidomonophosphanes, for example 1, have been one of our targets to visualize their ligand applicability in catalytic asymmetric carbon-carbon bond forming reactions.1 Their characteristics are there in phosphorous and amide carbonyl moieties as soft and hard coordinating sites for soft and hard metals. It was a logical extension to investigate the utility of the parent diphenylphosphinylmethyl¬pyrrolidine (2) in chiral pyrrolidine-mediated asymmetric reaction of aldehydes.2 The pioneering work has been done by Hiroi, where the intermolecular asymmetric allylation of enamine preformed from β-keto esters and 2 was catalyzed by palladium giving allylated quaternary carbon with high ee.3 We set our goal in the development of catalytic asymmetric intramolecular allylation of aldehydes4 by using catalytic or substoichiometric amount of chiral pyrrolidine derivatives. We describe herein the catalytic asymmetric intramolecular allylation of aldehydes with use of L-proline (3) and (S)-2-((diphenyl¬phosphino)¬methyl)¬pyrrolidine (2).5
RESULTS AND DISCUSSIONS
We began our studies with intramolecular allylation of allylic bromide bearing a terminal formyl group 6a using a stoichiometric amount of L-proline (3) as an enamine-forming agent. The requisite starting aldehydes 6a,b were prepared in one step from a known alkene (5)6 by olefin metathesis with allyl bromide and allyl carbonate in moderate yields.
The reaction of 6a in DMSO was conducted in the presence of 0.2 equiv of tetrakistriphenylphosphine palladium, 1.3 equiv of triethylamine, and 1.2 equiv of L-proline (3) at rt for 0.5 h to give, after treatment with 10% HCl for 0.5 h, a 10:1 trans/cis mixture of 5-membered cyclized 77 in 70% yield and trace amount of 7-membered 8 (Table 1, entry 4). The effect of Pd(0) was apparent from the decreased 15% yield of 7 under the absence of it (entries 1 and 2). The presence of triethylamine was also important to find decreased 43% yield by the reaction under its absence (entries 1 and 3). In the absence of 3 the desired products 7 and 8 were not produced (entry 5). DMSO was superior to chloroform and DMF to give higher yield of 7 (entries 6 and 7). The enantioselectivity of the reaction was not high to give 7 with fair ee of 19-27%.8
Fortunately the catalytic 0.2 equiv of L-proline (3) mediated the allylic cyclization of 6a for 0.5 h to give 7 in 57% yield in the similar level of enantioselectivity, 27% ee, observed in the stoichiometric reaction (entry 8). On the other hand, catalytic 0.2 equiv and stoichiometric amounts of prolinol (4) and phosphane 2 were not the choice to give the trace amount of 7 (entries 9 and 10). Production of SN2 adduct of 6a with 2 and 4 was suspected in these two unsuccessful cases.
Allylic carbonate 6b changed the situation from the reaction of bromide 6a to provide the circumstances fitted with catalytic conditions (Table 2). The reaction of 6b was catalyzed with 0.2 equiv of L-proline (3) and 0.2 equiv of Pd(0) to give 7 in the range of yields 52-67% when acidic proton sources such as triethylamine hydrochloride, triethylamine acetate, and acetic acid were presented (entries 1-5). Under the absence conditions of such acidic sources the production of 7 did not exceed 20% yield. The reaction was also catalyzed by 2 in the presence of acidic proton sources to give 7 in maximum 45% yield with the similar level of 26% ee as the reaction with 3 (entries 6-8). DMSO was again the choice of solvent other than chloroform, acetonitrile and THF (entries 9-11).
The structure of 8 was determined by separation from 7 after reduction to a separable mixture of the corresponding alcohols 9 and 10. 5-Exo-cyclization is reasonably rationalized for the formation of 7 preferentially to 8.
CONCLUSION
The aminophosphane was found to catalyze the intramolecular allylation of allylic carbonate bearing terminal formyl group giving the corresponding 5-exo-cyclized aldehyde in moderate efficiency. L-Proline was also fond to catalyze the cyclization of allylic bromide as well as carbonate to give 5-exo-cyclization product in good yield. The level of enantioselectivity was not high enough to apply in the target synthesis.
EXPERIMENTAL
1H and 13C-NMR spectra were taken at 500 and 125 MHz, respectively. 13C peak multiplicity assignments were made based on DEPT data. Chemical shift values are expressed in ppm relative to internal TMS. Abbreviations are as follows: s, singlet; d, doublet; t, triplet; q, quartet; m, multiplet; br, broad. Merck precoated silica gel plate was used for tlc analysis.
(E)-3-(2-(3-Bromoprop-1-enyl)phenyl)propanal (6a): Under argon atmosphere, a mixture of aldehyde 56 (3.6 g, 22.7 mmol), allyl bromide (19 mL, 227 mmol) and Grubbs’ 2nd catalyst, benzylidene[1,3-bis(2,4,6-trimethylphenyl)-2-imidazolidinylidene]dichloro(tricyclohexylphosphine)ruthenium,9 (5.8 mg, 6.8 mmol) in CH2Cl2 (450 mL) was stirred for 5 h under reflux. Concentration and silica gel column chromatography (hexane/AcOEt = 9/1) gave a 9:1 E/Z mixture of 6a (1.5 g, 26%) as a pale yellow oil of Rf 0.5 (hexane/AcOEt = 9/1×3). major E-olefin: 1H-NMR (CDCl3) δ : 2.74 and 3.01 (each 2H, t, J = 7.9 Hz), 4.17 (2H, d, J = 7.6 Hz), 6.30 (1H, td, J = 7.6, 15.2 Hz), 6.87 (1H, d, J = 15.2 Hz), 7.15–7.24 (3H, m), 7.45 (1H, m), 9.83 (1H, s). 13C-NMR (CDCl3) δ: 25.3 (CH2), 33.1 (CH2), 44.7 (CH2), 126.6 (CH), 126.9 (CH), 127.6 (CH), 128.5 (CH), 129.4 (CH), 131.5 (CH), 134.6 (C), 138.1 (C), 201.3 (CH). minor Z-olefin: 1H-NMR (CDCl3) δ : 2.74 (2H, t, J = 7.9 Hz), 2.92 (2H, t, J = 7.9 Hz), 4.03 (2H, d, J = 8.2 Hz), 6.07 (1H, td, J = 8.2, 11.3 Hz), 6.68 (1H, d, J = 11.3 Hz), 7.15–7.24 (3H, m), 7.45 (1H, m), 9.80 (1H, s). IR (neat): 1720 cm–1. EIMS m/z: 254 (M++2), 252 (M+), 173. HREIMS m/z: 252.0154 (Calcd for C12H13OBr: 252.0150).
(E)-Methyl 3-(2-(3-oxopropyl)phenyl)allyl carbonate (6b): Under argon atmosphere, the mixture of aldehyde 5 (2.0 g, 0.012 mol), allyl methyl carbonate (13.7 mL, 0.12 mol) and Hoveyda-Grubbs Catalyst 2nd Generation, (1,3-bis-(2,4,6-trimethylphenyl)-2-imidazolidinylidene)dichloro(o-isopropoxyphenylmethylene)ruthenium,10 (752 mg, 1.2 mmol) in CH2Cl2 (240 mL) was stirred for 13 h under reflux. Concentration, column chromatography (hexane/AcOEt = 9/1) and Kugel distillation gave E-6b (565 mg, 19%) as a colorless oil of Rf 0.2 (hexane/AcOEt = 9/1×3). 1H-NMR (CDCl3) δ: 2.72 and 3.01 (each 2H, t, J = 7.3 Hz), 3.81 (3H, s), 4.81 (2H, d, J = 6.4 Hz), 6.18 (1H, dt, J = 15.5, 6.4 Hz), 6.91 (1H, d, J = 15.5 Hz), 7.1–7.3 (3H, m), 7.44 (1H, m), 9.82 (1H, s). 13C-NMR (CDCl3) δ: 25.7 (CH2), 45.0 (CH2), 55.1 (CH3), 68.7 (CH2), 125.3 (CH), 127.0 (CH), 127.2 (CH), 128.7 (CH), 129.7 (CH), 132.3 (CH), 135.3 (C), 138.4 (C), 156.0 (C), 201.6 (CH). IR (neat): 1751 cm–1. EIMS m/z: 248 (M+), 172. Anal. Calcd for C14H16O4: C, 67.73; H, 6.50. Found: C, 67.79; H, 6.57.
(1R*,2S*)-1-Vinyl-2,3-dihydro-1H-indene-2-carbaldehyde (trans-7) from 6a using 3: Under argon atmosphere, a suspension of aldehyde 6a (126 mg, 0.5 mmol, E:Z = 9:1), L-proline (3) (11.5 mg, 0.1 mmol), Pd(PPh3)4 (115 mg, 0.1 mmol), and triethylamine (0.09 mL, 0.65 mmol) in DMSO (20 mL) was stirred for 0.5 h at rt. After addition of 10% HCl (5 mL) the mixture was stirred for another 0.5 h at rt. The organic layer was separated and the aqueous layer was extracted with benzene (60 mL). Combined organic layers were washed successively with water, 10% HCl, satd. aq. NaHCO3, and brine, and then dried over sodium sulfate. Concentration and silica gel column chromatography (hexane/benzene = 2/1) gave a 9:1 trans/cis mixture of 7 (49 mg, 57%) as a pale yellow oil of [α]25D –37.9 (c 1.40, CHCl3) and Rf 0.5 (hexane/benzene = 2/1×3). Trace amount of 8 was detected by gas chromatography. Ees of trans- and cis-7 were determined by gas chromatography (chirasil dex CB, 120 °C, helium gas constant flow of 4 mL/min, trans-7: Rt = 12.5 min (64%), 12.8 min (37%); cis-7: 17.2 min (48%), 18.1 min (52%)) to be 27% ee and 4% ee, respectively. major trans-7: 1H-NMR (CDCl3) δ: 3.10–3.28 (3H, m), 4.09 (1H, m), 5.20–5.26 (2H, m), 5.91 (1H, ddd, J = 8.3, 10.1, 17.1 Hz), 7.15–7.24 (4H, m), 9.85 (1H, d, J = 1.9 Hz). 13C-NMR (CDCl3) δ: 31.8 (CH2), 50.5 (CH), 58.2 (CH), 117.1 (CH), 124.64 (CH), 124.69 (CH), 127.00 (CH), 127.5 (CH), 138.7 (CH2), 140.8 (C), 143.1 (C), 202.4 (CH). minor cis-7: 1H-NMR (CDCl3) δ: 3.04 (1H, m), 3.35-3.43 (2H, m), 4.26 (1H, m), 5.16–5.26 (2H, m), 5.86 (1H, m), 7.15–7.24 (4H, m), 9.79 (1H, d, J = 2.4 Hz). IR (neat): 1720 cm–1. EIMS m/z: 172 (M+). HREIMS m/z: 172.0890 (Calcd for C12H12O: 172.0888).
(1R*,2S*)-1-Vinyl-2,3-dihydro-1H-indene-2-carbaldehyde (trans-7) from 6b using 2: Under argon atmosphere, a suspension of aldehyde 6b (50 mg, 0.2 mmol), aminophosphane 2 (11 mg, 0.04 mmol), Pd(PPh3)4 (46 mg, 0.04 mmol), acetic acid (0.015 mL, 0.26 mmol) and triethylamine (0.036 mL, 0.26 mmol) in DMSO (10 mL) was stirred for 61 h at rt. After addition of 10% HCl (5 mL) the reaction mixture was stirred for 0.5 h at rt. The organic layer was separated and the aqueous layer was extracted with benzene (35 mL). Combined organic layers were washed successively with water, 10% HCl, satd. aq. NaHCO3, and brine, and then dried over sodium sulfate. Concentration and silica gel column chromatography (hexane/benzene = 1/1) gave a 94:6 trans/cis mixture of 7 (15.5 mg, 45%) as a pale yellow oil of [α]25D –29.2 (c 0.53, CHCl3). Ees of trans- and cis-7 were determined to be 27% ee and 26% ee by gas chromatography.
Isolation of benzo[c]cyclohepta-5-enylmethanol (10) by reduction of 7 and 8: A solution of an 89:11 trans/cis-mixture of 7, 8 (40 mg, 0.23 mmol, 7:8 = 85:15) and sodium borohydride (35 mg, 0.92 mmol) in methanol (0.4 mL) was stirred for 1 h at rt. After addition of water (5 mL) the mixture was stirred for another 10 min and then extracted with AcOEt (35 mL). Combined organic layers were washed with brine and dried over sodium sulfate. Concentration and silica gel column chromatography (hexane/AcOEt = 4/1) gave 9 (27 mg, 67%) as colorless needle of mp 81–82 °C and 10 (3 mg, 7%) as pale yellow oil. 9: Rf 0.25 (hexane/AcOEt = 9/1×3). 1H-NMR (CDCl3) δ: 1.43 (1H, br), 2.46 (1H, m), 2.76 (1H, dd, J = 9.3, 15.7 Hz), 3.09 (1H, dd, J = 8.1, 15.7 Hz), 3.56 (1H, m), 3.77 (1H, m), 3.87 (1H, m), 5.17–5.22 (2H, m), 5.86 (1H, ddd, J = 8.8, 10.1, 16.8 Hz), 7.11 (1H, m), 7.17–7.19 (2H, m), 7.22 (1H, m). 13C-NMR (CDCl3) δ: 34.9 (CH2), 49.3 (CH), 52.8 (CH), 65.4 (CH2), 116.4 (CH2), 124.4 (CH), 124.6 (CH), 126.4 (CH), 126.9 (CH), 140.5 (CH), 142.4 (C), 144.8 (C). IR (neat): 3425 cm–1. EIMS m/z: 174 (M+). Anal. Calcd For C12H14O: C, 82.72; H, 8.10. Found: C, 82.76; H, 8.08. 10: Rf 0.21 (hexane/AcOEt = 9/1×3). 1H-NMR (CDCl3) δ: 1.33 (1H, br), 2.14–2.26 (2H, m), 2.45 (1H, m), 2.74 (1H, dd, J = 8.2, 14.1 Hz), 2.87 (1H, dd, J = 1.8, 14.1 Hz), 3.56 (2H, m), 5.91 (1H, dt, J = 4.8, 12.0 Hz), 6.45 (1H, m), 7.10–7.19 (4H, m). 13C-NMR (CDCl3) δ: 33.7 (CH2), 37.9 (CH2), 41.9 (CH), 66.9 (CH2), 126.1 (CH), 126.8 (CH), 129.5 (CH), 130.1 (CH), 130.3 (CH), 130.4 (CH), 136.6 (C), 139.2 (C). IR (neat): 3409 cm–1. EIMS m/z: 174 (M+). HREIMS m/z: 174.1043 (Calcd for C12H14O: 174.1045).
ACKNOWLEDGEMENTS
This research was supported by the COE Program “Knowledge Information Infrastructure for Genome Science”, a Grant-in-Aid for Scientific Research on Priority Areas “Advanced Molecular Transformations”, and a Grant-in-Aid for Scientific Research from the Ministry of Education, Culture, Sports, Science and Technology, Japan.
#Dedicated to Professor Emeritus Keiichiro Fukumoto on the occasion of his 75th birthday.
References
1. (a) M. Kuriyama, T. Soeta, X. Hao, Q. Chen, and K. Tomioka, J. Am. Chem. Soc., 2004, 126, 8128; CrossRef (b) T. Soeta, M. Kuriyama, and K. Tomioka, J. Org. Chem., 2005, 70, 297; CrossRef (c) Q. Chen, T. Soeta, M. Kuriyama, K. Yamada, and K. Tomioka, Adv. Synth. Catal., 2006, 348, 2604; CrossRef (d) K. Tomioka, Pure Appl. Chem., 2006, 78, 2029; CrossRef (e) T. Soeta, K. Selim, M. Kuriyama, and K. Tomioka, Tetrahedron, 2007, 63, 6573; CrossRef (f) T. Soeta, K. Selim, M. Kuriyama, and K. Tomioka, Adv. Synth. Catal., 2007, 349, 629; CrossRef (g) K. Selim, T. Soeta, K. Yamada, and K. Tomioka, Chem. Asian J., 2008, 3, 342. CrossRef
2. (a) Enantioselective Organocatalysis: Reactions and Experimental Procedures, ed. by P. I. Dalko, 2007, Wiley-VCH, Weinheim; (b) S. Mukherjee, J. W. Yang, S. Hoffmann, and B. List, Chem. Rev., 2007, 107, 5471. CrossRef
3. K. Hiroi and J. Abe, Tetrahedron Lett., 1990, 31, 3623. CrossRef
4. Efficient intramolecular asymmetric alkylation instead of allylation of aldehyde has been reported. N. Vignola and B. List, J. Am. Chem. Soc., 2004, 126, 450. CrossRef
5. Allylation of carbonyl compounds has been reported recently. (a) I. Ibrahem and A. Córdova, Angew. Chem. Int. Ed., 2006, 45, 1952; CrossRef (b) F. Bihelovic, R. Matovic, B. Vulovic, and R. N. Saicic, Org. Lett., 2007, 9, 5063. CrossRef
6. G. A. Schmid and H.-J. Borschberg, Helv. Chim. Acta, 2001, 84, 388. CrossRef
7. The trans-stereochemistry was assigned to major 7 based on its behavior under acidic isomerization conditions. H. Kogen, K. Tomioka, S. Hashimoto, and K. Koga, Tetrahedron, 1981, 37, 3951. CrossRef
8. The absolute configuration of 7 was not determined yet.
9. M. Scholl, S. Ding, C. W. Lee, and R. H. Grubbs, Org. Lett., 1999, 1, 953. CrossRef
10. J. S. Kingsbury, J. P. A. Harrity, P. J. Bonitatebus, and A. H. Hoveyda, J. Am. Chem. Soc., 1999, 121, 791 CrossRef