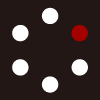
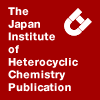
HETEROCYCLES
An International Journal for Reviews and Communications in Heterocyclic ChemistryWeb Edition ISSN: 1881-0942
Published online by The Japan Institute of Heterocyclic Chemistry
e-Journal
Full Text HTML
Received, 30th January, 2008, Accepted, 8th April, 2008, Published online, 11th April, 2008.
DOI: 10.3987/COM-08-S(F)1
■ One-Pot Synthesis of 3,3-Dimethylpyrrolidine-2-carbonitriles from 4-Chloro-2,2-dimethylbutanal in Water
Matthias D’hooghe, Berten Van Driessche, and Norbert De Kimpe*
Department of Organic Chemistry, Faculty of Bioscience Engineering, Ghent University, Coupure Links 653, B-9000 Ghent, Belgium
Abstract
Treatment of 4-chloro-2,2-dimethylbutanal, prepared in high yield by means of a three-step procedure starting from ethyl isobutyrate, with successively sodium bisulphite, a primary amine and potassium cyanide in water afforded novel 1-alkyl- and 1-aryl-3,3-dimethylpyrrolidin-2-carbonitriles in a convenient and environmentally benign way.INTRODUCTION
In recent years, pyrrolidine-2-carbonitriles have attracted considerable attention from medicinal chemists due to their diverse biological activities. In particular, many efforts have been devoted to the synthesis of novel 2-cyanopyrrolidines as dipeptidyl peptidase IV inhibitors, which are of importance for the treatment of type II diabetes.1 The most advanced 2-cyanopyrrolidine, vildagliptin 1, is now even on the verge of entering the market. Furthermore, pyrrolidine-2-carbonitriles are of interest as building blocks in synthetic organic chemistry, especially as precursors for the preparation of proline analogues.2 Also other applications have been reported, such as the synthesis of biologically relevant alkaloids and cage compounds.3 Consequently, the development of new and efficient entries towards 2-cyanopyrrolidines remains of significant importance from both a biological and a synthetic point of view.
In the literature, few general procedures for the preparation of monocyclic 1-alkylpyrrolidine-2-carbonitriles are available. For example, 1-alkyl-2-cyanopyrrolidines have been prepared via transformation of 2-carbamoyl-4 and 2-(alkoxycarbonyl)pyrrolidines,5 via indium-promoted conjugate addition-cyclization reactions of ethenetricarboxylates with propargylamines,6 via reductive cyanation of pyrrolidin-2-ones through acyliminium intermediates,7 and via oxidation of 1-alkylpyrrolidines with ClO2 followed by the in situ reaction of intermediate iminium ions with cyanide.8 As for the synthesis of 1-arylpyrrolidin-2-carbonitriles, an electrochemical cyanation9 and a ruthenium-catalyzed oxidative cyanation of 1-arylpyrrolidines10 have been reported. From this overview, it is clear that the lack of simple and general approaches towards 1-alkyl- and 1-arylpyrrolidin-2-carbonitriles impedes the study of this type of heterocycles as substrates for further elaboration or as relevant target compounds.
In the present paper, a novel and elegant protocol for the preparation of a variety of 1-substituted 3,3-dimethylpyrrolidin-2-carbonitriles will be discussed, based on the cyclization of γ-chloroimines in water triggered by the addition of cyanide across the imino moiety. In recent years, the demand for environmentally benign processes has become an important element in the design of new synthetic methodologies. In this respect, organic reactions in aqueous media constitute an attractive alternative for the use of classical solvents.11 Despite of the simplicity, only one report is available in the literature based on this approach, affording an elegant entry into 1-(1-phenylethyl)pyrrolidine-2-carbonitriles through cyclization of γ-chloroimines. For this purpose, 2-methyl-2-[(1-phenylethyl)amino]propanenitrile was used as an in situ source of α-phenylethylamine and hydrogen cyanide, resulting in the formation of intermediate (4-chlorobutylidene)-N-(1-phenylethyl)amines from the corresponding aldehydes, followed by cyclization upon nucleophilic addition of cyanide in acetonitrile.12 Also α,α,γ-trichlorinated aldehydes have been used previously for the synthesis of intermediate α,α,γ-trichlorinated imines, which were subsequently cyclised towards pyrrolidine13 or pyrroline14 derivatives. The analogous reductive cyclization of δ-haloimines towards the corresponding piperidines has been studied in more detail in the past,15 although in only one case the synthesis of 2-cyanopiperidines has been reported.16
RESULTS AND DISCUSSION
4-Chloro-2,2-dimethylbutanal 5 was chosen as a substrate for the synthesis of a variety of corresponding imines by condensation with the appropriate primary amines. The cyclization of the latter γ-chloroimines would then be evaluated as a new approach towards 2-cyanopyrrolidines.
Based on literature data, 4-chloro-2,2-dimethylbutan-1-ol 4 was prepared on a large scale upon alkylation of ethyl isobutyrate 2 with one equivalent of 1-bromo-2-chloroethane in THF in the presence of 1.1 equivalent of LDA (lithium diisopropylamide) affording ethyl 4-chloro-2,2-dimethylbutyrate 3 in 95% yield, followed by reduction of the latter ester 3 using 1.5 equivalents of lithium borohydride (LiBH4) in dichloromethane upon reflux for 16 hours (Scheme 1).17 The desired 4-chloro-2,2-dimethylbutanal 518 could then easily be prepared by oxidation of alcohol 4 utilizing two equivalents of pyridinium chlorochromate (PCC) coated on silicagel in dichloromethane at room temperature for 2 hours (Scheme 1).19 The application of Swern oxidation conditions was unsuccessful and yielded only the starting compound.
The condensation of β-chloroimines with primary amines (e.g. tert-butylamine, isopropylamine, benzylamine, aniline, 1-amino-2,2-dimethylcyclopropanecarbonitrile) towards the corresponding imines by means of standard conditions (MgSO4/CH2Cl2, TiCl4/Et2O or MgSO4/Et2O) has been studied before.20 In analogy, aldehyde 5 was treated with different primary amines in order to synthesize the corresponding γ-chloroimines 6 as substrates for further elaboration. However, in some cases the attempts to isolate the desired γ-chloroimines 6 in a pure state failed, and rather complex reaction mixtures were obtained. These observations prompted us to investigate an alternative one-pot approach without the isolation of the intermediate imines 6. Thus, the consecutive treatment of 4-chloro-2,2-dimethylbutanal 5 with one equivalent of sodium bisulphite, two equivalents of an amine and two equivalents of potassium cyanide in water afforded novel 3,3-dimethylpyrrolidin-2-carbonitriles 7 in acceptable yields, taking into account the difficulty to generate the intermediate reactive aldimines 6. The pyrrolidine-2-carbonitriles 7 were purified by means of column chromatography in order to obtain analytically pure samples (Scheme 2, isolated yields after column chromatography). In this way, 1-alkyl-, 1-allyl-, 1-benzyl- and 1-phenylpyrrolidin-2-carbonitriles were prepared for the first time via a convenient and straightforward approach. As described before, the cyclization of δ-chloroimines to piperidines through addition of potassium cyanide only occurred upon heating under reflux,15b,16 whereas pyrrolidines 7 were formed smoothly at room temperature. The fact that water is used as an alternative for classical organic solvents clearly adds value to this methodology from an environmental point of view. To underline the importance of the solvent, it is interesting to note that with the use of methanol as solvent, only complex reaction mixtures were obtained. Remarkably, the omission of sodium bisulphite in the three-step sequence from 5 to 7 resulted in a significant decrease of the yield, e.g. 13% instead of 36% for 1-allyl-3,3-dimethylpyrrolidine-2-carbonitrile 7a, pointing to the peculiar role of the inorganic salt in this transformation (stable adduct formation).
In summary, an easy and straightforward one-pot approach for the preparation of 1-alkyl- and 1-aryl-3,3-dimethylpyrrolidin-2-carbonitriles has been described involving the consecutive treatment of 4-chloro-2,2-dimethylbutanal with sodium bisulphite, an amine and potassium cyanide in water.
EXPERIMENTAL
1H NMR spectra were recorded at 300 MHz (JEOL ECLIPSE+) with CDCl3 as solvent and tetramethylsilane as internal standard. 13C NMR spectra were recorded at 75 MHz (JEOL ECLIPSE+) with CDCl3 as solvent. Mass spectra were obtained with an AGILENT 1100, 70 eV. IR spectra were measured with a Spectrum One FT-IR spectrophotometer. Tetrahydrofuran was distilled over sodium benzophenone ketyl and dichloromethane was distilled over calcium hydride. Other solvents were used as received from the supplier. Elemental analyses were performed with a PerkinElmer Series II CHNS/O Analyzer 2400.
Synthesis of 3,3-dimethylpyrrolidine-2-carbonitriles 7
The synthesis of 1-allyl-3,3-dimethylpyrolidine-2-carbonitrile 7a is described here as a representative example for the synthesis of 3,3-dimethylpyrrolidine-2-carbonitriles 7. A solution of sodium bisulphite (1.41 g, 7.43 mmol, 1 equiv) in water (10 mL) was added dropwise to a stirred solution of 4-chloro-2,2-dimethylbutanal 5 (1.00 g, 7.43 mmol) in water (10 mL) at rt. The resulting mixture was stirred for 2 h at rt, after which allylamine (0.85 g, 14.87 mmol, 2 equiv) was added. This mixture was again stirred for 2 h at rt and subsequently treated with potassium cyanide (0.96 g, 14.87 mmol, 2 equiv) in water (10 mL). After stirring overnight (18 h) at rt, the aqueous phase was extracted with Et2O (3×30 mL). Drying (MgSO4), filtration of the drying agent, evaporation of the solvent in vacuo and purification by means of column chromatography (hexane/EtOAc 10/1) yielded pure 1-allyl-3,3-dimethylpyrolidine-2-carbonitrile 7a (0.12 g, 2.67 mmol, 36%).
1-Allyl-3,3-dimethylpyrrolidine-2-carbonitrile 7a. Yield 36%. Colorless oil. Column chromatography: hexane/EtOAc 10/1, Rf = 0.33. 1H NMR (300 MHz, CDCl3): δ 1.20 and 1.28 (2×3H, 2×s, 2×CH3), 1.58-1.78 (2H, m, CH2C(CH3)2), 2.71 (1H, ~t×d, J = 9.2 Hz, J = 6.1 Hz, C(H)HN), 2.81-2.89 (1H, m, C(H)HN), 3.14 (1H, d×d, J = 13.3 Hz, J = 7.3 Hz, C(H)HCH=CH2), 3.35 (1H, s, CHCN), 3.38 (1H, d×d×t, J = 13.3 Hz, J = 5.5 Hz, J = 1.4 Hz, C(H)HCH=CH2), 5.14-5.33 (2H, m, CH=CH2), 5.78-5.91 (1H, m, CH=CH2). 13C NMR (75 MHz, CDCl3): δ 26.24 and 29.94 (2×CH3), 37.89 (CH2C(CH3)2), 41.80 (C(CH3)2), 50.94 (CH2CH2N), 55.86 (CH2CH=CH2), 65.43 (CHCN), 116.98 (CN), 118.14 (CH=CH2), 134.52 (CH=CH2). IR (NaCl, cm-1): νCN = 2221. MS (70 eV): m/z (%): 165 (M+1+, 100). Anal. Calcd for C10H16N2: C 73.13, H 9.82, N 17.06. Found: C 73.28, H 10.09, N 16.92.
1-Benzyl-3,3-dimethylpyrrolidine-2-carbonitrile 7b. Yield 16%. Colorless oil. Column chromatography: hexane/EtOAc 10/1, Rf = 0.50. 1H NMR (300 MHz, CDCl3): δ 1.20 and 1.28 (2×3H, 2×s, 2×CH3), 1.61-1.80 (2H, m, CH2C(CH3)2), 2.69 (1H, ~t×d, J = 9.6 Hz, J = 6.2 Hz, C(H)HN), 2.87-2.95 (1H, m, C(H)HN), 3.28 (1H, s, CHCN), 3.63 (1H, d, J = 13.2 Hz, C(H)HC5H6), 3.93 (1H, d, J = 13.2 Hz, C(H)HC5H6), 7.23-7.56 (5H, m, C6H5). 13C NMR (75 MHz, CDCl3): δ 26.05 and 28.92 (2×CH3), 37.64 (CH2C(CH3)2), 41.76 (C(CH3)2), 50.78 (CH2N), 56.74 (CH2C6H5), 65.23 (CHCN), 116.68 (CN), 127.35, 128.44 and 128.66 (CHarom), 137.80 (NCH2Cq). IR (NaCl, cm-1): νCN = 2210. MS (70 eV): m/z (%): 215 (M+1+, 100). Anal. Calcd for C14H18N2: C 78.46, H 8.47, N 13.07. Found: C 78.31, H 8.71, N 12.94.
3,3-Dimethyl-1-phenylpyrrolidine-2-carbonitrile 7c. Yield 24%. White crystals. Mp 67 °C. Column chromatography: hexane/EtOAc 10/1, Rf = 0.26. 1H NMR (300 MHz, CDCl3): δ 1.16 en 1.43 (2×3H, 2×s, 2×CH3), 1.82-1.89 (1H, m, C(H)HC(CH3)2), 2.04-2.14 (1H, m, C(H)HC(CH3)2), 3.47 (2H, d×d, J = 9.1 Hz, J = 4.7 Hz, CH2N), 4.03 (1H, s, CHCN), 6.65-6.68, 6.79-6.84 and 7.25-7.32 (2H, 1H and 2H, 3×m, C6H5). 13C NMR (75 MHz, CDCl3): δ 24.60 and 26.11 (2×CH3), 37.47 (CH2C(CH3)2), 42.38 (C(CH3)2), 46.51 (CH2N), 60.13 (CHCN), 112.31, 117.93 and 129.44 (CHarom), 117.69 (CN), 145.49 (CqN). IR (NaCl, cm-1): νCN = 2217. MS (70 eV): m/z (%): 201 (M+1+, 100). Anal. Calcd for C13H16N2: C 77.96, H 8.05, N 13.99. Found: C 77.78, H 8.23, N 14.11.
3,3-Dimethyl-1-isopropylpyrrolidine-2-carbonitrile 7d. Yield 13%. Colorless oil. Column chromatography: hexane/EtOAc 10/1, Rf = 0.42. 1H NMR (300 MHz, CDCl3): δ 1.08 and 1.12 (2×3H, 2×d, J = 6.3 Hz, CH(CH3)2), 1.19 and 1.28 (2×3H, 2×s, C(CH3)2), 1.57-1.85 (2H, m, CH2C(CH3)2), 2.61 (1H, ~t×d, J = 9.3 Hz, J = 6.1 Hz, C(H)HN), 2.77 (1H, sept, J = 6.3 Hz, CH(CH3)2), 3.04 (1H, ~t×d, J = 9.3 Hz, J = 5.4 Hz, C(H)HN), 3.50 (1H, s, CHCN). 13C NMR (75 MHz, CDCl3): δ 19.68 and 21.76 (CH(CH3)2), 25.88 and 28.84 (C(CH3)2), 37.53 (CH2C(CH3)2), 42.45 (C(CH3)2), 48.31 (CH2N), 51.47 (CHCH3)2), 63.81 (CHCN), 117.26 (CN). IR (NaCl, cm-1): νCN = 2229. MS (70 eV): m/z (%): 167 (M+1+, 10). Anal. Calcd for C10H18N2: C 72.24, H 10.91, N 16.85. Found: C 72.40, H 11.13, N 16.71.
1-tert-Butyl-3,3-dimethylpyrrolidine-2-carbonitrile 7e. Yield 17%. Colorless oil. Column chromatography: hexane/EtOAc 10/1, Rf = 0.41. 1H NMR (300 MHz, CDCl3): δ 1.08 and 1.28 (2×3H, 2×s, CH(CH3)2), 1.15 (3×3H, s, C(CH3)3), 1.56 (1H, d×d×d, J = 12.3 Hz, J = 6.9 Hz, J = 3.4 Hz, C(H)HC(CH3)2), 1.74-1.83 (1H, m, C(H)HC(CH3)2), 2.85 (1H, ~t×d, J = 8.5 Hz, J = 7.0 Hz, C(H)HN), 2.99 (1H, t×d, J = 8.5 Hz, J = 3.5 Hz, C(H)HN), 3.43 (1H, s, CHCN). 13C NMR (75 MHz, CDCl3): δ 25.72 and 27.37 (C(CH3)2), 26.73 (C(CH3)3), 38.56 (CH2C(CH3)2), 41.86 (C(CH3)2), 44.70 (CH2N), 53.33 (C(CH3)3), 59.59 (CHCN), 121.48 (CN). IR (NaCl, cm-1): νCN = 2231. MS (70 eV): m/z (%): 181 (M+1+, 80). Anal. Calcd for C11H20N2: C 73.28, H 11.18, N 15.54. Found: C 73.46, H 11.37, N 15.71.
ACKNOWLEDGEMENTS
The authors are indebted to the “Fund for Scientific Research - Flanders (Belgium)” (F.W.O.-Vlaanderen) and to Ghent University (GOA) for financial support.
This paper is dedicated to Professor Emeritus Keiichiro Fukumoto on the occasion of his 75th birthday.
References
1. For a review, see: J.-U. Peters, Curr. Top. Med. Chem., 2007, 7, 579. CrossRef
2. (a) P.-Q. Huang and H.-Y. Huang, Synth. Commun., 2004, 34, 1377; CrossRef (b) G. Sunilkumar, D. Nagamani, N. P. Argade, and K. N. Ganesh, Synthesis, 2003, 2304; CrossRef (c) M. V. Gorichko, O. O. Grygorenko, and I. V. Komarov, Tetrahedron Lett., 2002, 43, 9411. CrossRef
3. (a) G. Gauthier, J. Royer, and H.-P. Husson, Eur. J. Org. Chem., 2002, 1484; CrossRef (b) M. Oba, S. Koguchi, and K. Nishiyama, Tetrahedron, 2002, 58, 9359; CrossRef (c) F. F. Fleming, L. Funk, R. Altundas, and Y. Tu, J. Org. Chem., 2001, 66, 6502. CrossRef
4. (a) F. Couty, O. David, B. Larmanjat, and J. Marrot, J. Org. Chem., 2007, 72, 1058; CrossRef (b) C. Li, C. Chan, A. C. Heimann, and S. J. Danishefsky, Angew. Chem. Int. Ed., 2007, 46, 1444. CrossRef
5. C. Pellegrini, C. Straessler, M. Weber, and H.-J. Borschberg, Tetrahedron: Asymmetry, 1994, 5, 1979. CrossRef
6. S. Morikawa, S. Yamazaki, Y. Furusaki, N. Amano, K. Zenke, and K. Kakiuchi, J. Org. Chem., 2006, 71, 3540. CrossRef
7. J.-O. Durand, M. Larcheveque, and Y. Petit, Tetrahedron Lett., 1998, 39, 5743. CrossRef
8. C. K. Chen, A. G. Hortmann, and M. R. Marzabadi, J. Am. Chem. Soc., 1988, 110, 4829. CrossRef
9. W. Liu, Y. Ma, Y. Yin, and Y. Zhao, Bull. Chem. Soc. Japan, 2006, 79, 577. CrossRef
10. S.-I. Murahashi, N. Komiya, and H. Terai, Angew. Chem. Int. Ed., 2005, 44, 6931. CrossRef
11. (a) C.-J. Li and T. H. Chan, ‘Organic Reactions in Aqueous Media’, Wiley, New York, 1997; (b) ‘Organic Synthesis in Water’, ed. by P. A. Grieco, Thomson Science, Glasgow, 1998; (c) ‘Organic Reactions in Water’, ed. by U. Lindström, Blackwell Publishing, Oxford, 2007.
12. O. O. Grygorenko, N. A. Kopylova, P. K. Mikhailiuk, A. Meissner, and I. V. Komarov, Tetrahedron: Asymmetry, 2007, 18, 290. CrossRef
13. L. De Buyck, N. Menke, and N. Schamp, Bull. Soc. Chim. Belges, 1990, 99, 121.
14. G. Verniest, S. Claessens, F. Bombeke, T. Van Thienen, and N. De Kimpe, Tetrahedron, 2005, 61, 2879. CrossRef
15. (a) W. H. Pearson, P. Stoy, and Y. Mi, J. Org. Chem., 2004, 69, 1919; CrossRef (b) W. Aelterman and N. De Kimpe, Tetrahedron, 1998, 54, 2563; CrossRef (c) C. Stevens and N. De Kimpe, J. Org. Chem., 1993, 58, 132. CrossRef
16. P. Sulmon, N. De Kimpe, and N. Schamp, Tetrahedron, 1989, 45, 3907. CrossRef
17. J.-L. Dasseux and C. D. Oniciu, PCT Int. Appl., WO 2003087040 A2, 2003, 123 pp (Chem. Abstr., 2003, 139, 337722).
18. (a) R. Tschesche and J. Reden, Liebigs Ann. Chem., 1974, 853; (b) P. Kocienski and M. Todd, J. Chem. Soc., Perkin Trans. 1, 1983, 1777. CrossRef
19. F. A. Luzzio, R. W. Fitch, W. J. Moore, and K. J. Mudd, J. Chem. Educ., 1999, 76, 974.
20. (a) P. Sulmon, N. De Kimpe, R. Verhe, L. De Buyck, and N. Schamp, Synthesis, 1986, 192; CrossRef (b) W. Aelterman, K. Abbaspour Tehrani, W. Coppens, T. Huybrechts, N. De Kimpe, D. Tourwé, and J.-P. Declercq, Eur. J. Org. Chem., 1999, 239 CrossRef