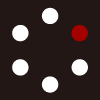
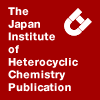
HETEROCYCLES
An International Journal for Reviews and Communications in Heterocyclic ChemistryWeb Edition ISSN: 1881-0942
Published online by The Japan Institute of Heterocyclic Chemistry
e-Journal
Full Text HTML
Received, 24th May, 2008, Accepted, 10th July, 2008, Published online, 10th July, 2008.
DOI: 10.3987/REV-08-SR(F)2
■ Progress towards the Total Synthesis of the Bioactive Calothrixins A and B
Tominari Choshi and Satoshi Hibino*
Faculty of Pharmacy and Pharmaceutical Sciences, Fukuyama University, Fukuyama, Hiroshima 729-0292, Japan
Abstract
During the past decade, the total synthesis of calothrixin A and B, bioactive metabolites from cyanobacteria Calothrix sp., has been independently reported by six groups. Here, we describe the development of these synthetic efforts, including two biomimetic routes via indolo[2,3-a]carbazole.INTRODUCTION
Calothrixins A (1) and B (2), which possess a novel indolo[3,2-j]phenanthridine pentacyclic ring system, were originally isolated from Calothrix cyanobacteria in 1999 by the group of Rickards (Figure 1).1 These unique pentacyclic quinones exhibit remarkable biological activity, most notably their growth inhibitory effects at nanomolar concentrations on a chloroquine resistant strain of the malarial parasite Plasmodium falciparum, as well as activity against human Hela cancer cells, and inhibition of RNA polymerase activity.1-6 Rickards et al. proposed that calothrixins A (1) and B (2) may be derived biosynthetically from a hypothetical metabolite 3 of the relatively common indolo[2,3-a]carbazole type,1 which is closely related to the known 6-cyano-5-methoxyindolo[2,3-a]carbazole (4)7,8 isolated from cyanobacterium Nostoc sphaericum. To date, eight synthetic schemes, including two biosynthetic routes, to calothrixins (1 and 2) have been reported by six research groups. In this review, we will summarize each total synthesis in turn, including the two biosynthetic routes.
1. KELLY’S TOTAL SYNTHESIS
The first total synthesis of calothrixin A (1) and B (2) was completed in short steps in 2000 by the Kelly group (Scheme 1).9 The known N-MOM-3-formylindole (5)10a and quinoline-4-carboxamide (6),10b derived from the disconnection at the C6a to C7 bond and the C12a to C13 bond in Scheme 1, were chosen for the construction of the C-ring on the basis of o-lithiation chemistry. Namely, treatment of 6 with 4 equivalents of lithium tetramethylpiperidide (LiTMP), followed by addition of the aldehyde 5 afforded the N-MOM calothrixin B (10) in a one pot operation (12% yield from 6). Removal of the N-MOM protecting group of 10 gave the calothrixin B (2) (74%), which was oxidized with mCPBA yielding calothrixin A (1) (71%).
2. CHAI’S TOTAL SYNTHESIS (1)
Chai’s retro synthetic analysis11,12 in Scheme 2 employs an initial disconnection at the C12a to C13 bond. A second disconnection is then made between C7a and C7 to give indole 11 and the quinoline-3-acyl chloride 12 (Scheme 2). Coupling of indole 11 to 3-chlorocarbonyl-4-methoxycarbonylquinoline (12)13a,b was carried out as follows. Treatment of indole 11 with ZnCl2 and MeMgCl followed by the addition of quinoline 12 under Friedel-Crafts conditions without AlCl3 reproducibly afforded the desired 3-acylindole 13 (80%). Subsequent treatment of the 3-acylindole 13 with NaH in THF followed by addition of MOMCl yielded N-MOM-3-acylindole 14 (99%). Lithiation of 14 with two equivalents of LDA (or LHMDS) in the presence of TMEDA gave the N-MOM calothrixin B (10) (54%). Cleavage of the N-MOM group was achieved by heating in conc. HCl to give calothrixin B (2) (83%).
3. GUINGANT’S TOTAL SYNTHESIS
Simultaneous creation of the C6-C6a and C13a-C13b bonds based on an intermolecular hetero Diels-Alder reaction has been carried out by the Gingant group.14,15 The key cycloaddition of the dienophile 1516a with the diene 1616b,c afforded the deprotected pentacyclic product 18 (80%) as a major component after 48 h. Similarly, dienophile 19 reacted with diene 16 to give the desired adduct 20 (80%). Deprotonation of 18 followed by triflation afforded a mixture of enol triflate 21 and aryl triflate 22. The mixture was subsequently heated in the presence of DDQ in dioxane to yield the triflate 22 (51% from two steps). Reductive cleavage of the O-triflate group with Pd(PPh3)4 and HCOOH provided calothrixin B (2) (75%). In addition, calothrixin B (2) can also be generated from the N-benzyl protected adduct 20. Oxidation of 20 with 10% Pd-C afforded phenol 23 (73%), which was then treated with Tf2O and Et3N to give the triflate 24 (94%). Reductive cleavage of the O-triflate group of 24 gave the N-benzyl calothrixin B 25 (95%). Finally, treatment with AlCl3 in benzene to remove the N-benzyl group produced calothrixin B (2) (57%).
4. HIBINO’S TOTAL SYNTHESIS
It was presumed that an 4-oxygenated 2,3,4-trisubstituted carbazole ring 26, derived from a disconnection at the N5-C6, might be obtained from 27 by an allene-mediated electrocyclic reaction of the 6π-electron system including the indole 2,3-bond (Scheme 4).17 The Wittig reaction of 2-formylindole 2818 with 2-nitrobenzyltriphenylphosphorane gave the trans-2-(2-styryl)indole 29 (96%). Subsequent treatment of 29 with Cl2CHOMe in the presence of AlCl3 afforded the 3-formylindole 30 (96%). The Grignard reaction of 30 with ethynylmagnesium bromide yielded the propargyl alcohol, which was protected with MOMCl and i-Pr2NEt to produce the O-MOM ether 27 (86% from 30). The desired 4-oxygenated 2,3,4-trisubstituted carbazole 26 was obtained by heating 27 in the presence of t-BuOK at 90oC (29%), along with an elimination of the phenylsulfonyl group. Sequential oxidation of 26 with DDQ followed by deprotection with 6N HCl gave the 3-formylcarbazole 32 (70%). Reduction of the nitro group of 31 with 10% Pd-C and H2 followed by the condensation afforded the indolo[3,2-j]phenanthridine 32, which was oxidized with CAN to provide calothrixin B (2) (67% from 31).
5. BENNASAR’S TOTAL SYNTHESIS
A new radical-based route at the C13 and C13a of calothrixin B (2) has been developed by the Bennasar group (Scheme 5).19 A radical precursor, selenoester 33 is synthesized from N-Boc-3-formylindole 34 as shown in Scheme 5. The reaction of 34 with 3-lithio-2-bromoquinoline, prepared from 2-bromoquinoline 35 with LDA, followed by Et3SiH reduction of the resulting alcohol provided the 3-alkylated 2-bromoquinoline 36 (55%). The 2-bromoquinoline 36 was converted into the deprotected quinoline 37 by treatment with n-Bu3SnH (90%). After N-protection of 37 with MOMCl and NaH (90%), hydrolysis of 38 with LiOH, followed by phenylselenation of the resulting carboxylic acid, gave the target compound 33 (80%) as a radical precursor. The connection of the C13 and C13a bond with the N-MOM-substituted 2-indolylacyl radical, generated from selenoester 33 under reductive conditions (TTMSS, AIBN), yields the pentacyclic phenol 39 (90%). The phenol 39 was converted to the known N-MOM calothrixin B 10 by oxidation with molecular oxygen in NaOH medium (98%).
6. CHAI’S TOTAL SYNTHESIS (2)
Two synthetic strategies for construction of the B-ring have been attempted by intramolecular aryl-aryl coupling reaction of 9-anilinophenanthridine 40 derived from a disconnection at the C7a and C7b, and a nitrene insertion reaction of 8-(2-nitrophenyl)phenanthridine 41 derived from a disconnection at the N12 and C12a (Scheme 6).20 However, attempts to cyclize the anilinophenanthridine 40 using catalytic amounts of Pd(OAc)2 in refluxing glacial AcOH in the presence of molecular oxygen failed. A second strategy involved using a nitrene intermediate generated from the 8-(2-nitropheny)phenanthridine 41 and its equivalent 42. The nitrophenylphenanthridine 42 was synthesized by using Suzuki-Miyaura coupling reaction of the 8-bromophenanthridine 46 (prepared from 3-bromo-2,5-dimethoxybenzoic acid (43) in three steps in 82% yield, according to the Harayama’s phenanthridine route21) with 2-nitrophenyl boronic acid (47) in the presence of Pd(OAc)2, PPh3, K2CO3 in DMF at 150o C (96%). The nitro compound 42 was then cyclized in the presence of triethylphosphite in a sealed tube at 174o C under microwave irradiation to the indolophenanthridinone 48 (89% yield). Subsequent reduction of the amide group of 48 with LiAlH4 gave the 7,10-dimethoxyindolo[3,2-j]phenanthridine 49, which was treated with excess BBr3 followed by quenching with dry MeOH and molecular oxygen to form calothrixin B (2) (97% from 48).
7. HYPOTHETICAL BIOSYNTHETIC ROUTE OF CALOTHRIXINS A (1) AND B (2)
A biosynthetic route to generate calothrixins A (1) and B (2) has been proposed by Rickards group as follows. The pentacyclic indolo[3,2-j]phenanthridine ring system may be derived biosynthetically from a hypothetical metabolite, 6-formyl-5-hydroxyindolo[2,3-a]carbazole (3). Oxidation of a phenol 3 to quinone-imine 50, followed by hydrolytic cleavage of the resulting imino group affords an o-aminophenylcarbazole-1,4-quinone 51. Rotation around the biaryl bond and condensation of an amine with a formyl group provides the pentacyclic calothrixin B (2). N-Oxidation generates the pentacyclic calothrixin A (1) (Scheme 7).1 This ring system of 1 is unique amongst natural products and was proposed to arise in nature from the more common tryptophan derived indolo[2,3-a]carbazole framework.8 Recently, two biomimetic total syntheses of 1 and 2 have appeared in the literature.22,23,24
8. HIBINO’S BIOMIMETIC TOTAL SYNTHESIS
It was envisaged that a 6-hydroxy-5-formylindolo[2,3-a]carbazole (3) or its derivative 60 might be derived through an allene-mediated electrocyclic reaction of the 6π-electron system involving two [b]-bond of indoles, generated from a propargyl ether 58 (Scheme 8).22 The Suzuki-Miyaura coupling reaction of 2-bromo-3-formylindole (52)25 with indole-2-boronic acid 53 gave the bisindole 54 (96%). Cleavage of the N-Boc group in 54 with TFA (82%), followed by protection of the nitrogen atom of bisindole 55 with MOMCl and NaH afforded N,N’-bis(methoxymethyl)bisindole 56 (71%). The Grignard reaction of 56 with ethynylmagnesium bromide yielded the propargyl alcohol 57 (86%), which was protected with MOMCl to produce the MOM ether 58 (78%). The propargyl ether 58 was treated with t-BuOK in t-BuOH amd THF at 90oC to yield the desired indolo[2,3-a]carbazole 59 (93%). Oxidation of 5-methylindolocarbazole 59 to 5-formylindolocarbazole 60 was attempted with DDQ in DMF to generate the expected indolocarbazole 60 (55%) as a protected metabolite 3. Further oxidation of 60 in order to convert to a quinone-imine like compound was examined with cerium ammonium nitrate (CAN) to give the N-MOM calothrixin 10 directly (40%). Treatment of 10 with conc. HCl afforded calothrixin B (2) (65%).
9. MOODY’S BIOMIMETIC TOTAL SYNTHESIS
A synthetic route to the key indolo[2,3-a]carbazole 3 is based on the synthesis of 5-cyano-6-methoxy-12-methylindolo[2,3-a]carbazole starting from indigo 63 (Scheme 9)23,24 according to the reaction scheme devised by Somei.26 Namely, a mixture of indigo 63 and tin powder in a solution of Ac2O in AcOH was heated at 64-66oC to give the monoacylated bisindole 64 (85%). Acylation of 65 with Cl2CH2COCl in EtOAc gave the dichloroacetylated bisindole 65 (79%), which was treated with aqueous NH3 to cause ring closure, thus producing cis-chlorohydrin 66 (75%). The reduction of 66 with Zn and NH4Cl afforded the known 5-hydroxyindolo[2,3-a]carbazole 67 (55%) and subsequent Vilsmeier reaction provided the desired 5-formyl-6-hydroxyindolocarbazole 3 (82%). In an attempt to effect the proposed biomimetic transformation, the indolocatbazole 3 was subjected to many different oxidation conditions. Unfortunately, however, all attempts to oxidize 3 have been unsuccessful. As a result, it was found that the fully protected indolocarbazole 6022 with MOM-group (44%) could be oxidized by CAN to produce the N-MOM calothrixin B 10 (31%). Finally, deprotection of N-MOM group with conc. HCl afforded calothrixin B (2) (100%).
CONCLUSIONS
The first total synthesis of calothrixin A and B was achieved by the Kelly group, which involved two key C-C bond forming reactions at C6a and C7, followed by C12a and C13, using an o-lithiation strategy.9 The structures 1 and 2 have been confirmed. Two further total syntheses have been reported by the Chai group. One is a short and concise route to calothrixin B (2) using Friedel-Crafts and lithiation reactions for the formation at the C7 to C7a, and the C12a to C13.11,12 The alternative synthesis is based on N-C bond formation at the N12 to C12a atom, utilizing a nitrene intermediate.20 This route, which involves seven steps, gave the highest overall yield (68%) among the eight reported synthetic schemes. Next, the Guingant group used a regioselective hetero Diels-Alder strategy as a key synthetic step in order to construct the D-ring.14,15 The cycloaddition reaction between the catbazole-1,4-quinone 15 and 2-azadiene 16 proceeds in 80% yield based on simultaneous C-C-bond formation at the C6 to C7a and C13a to C13b atoms. We chose an allene-mediated electrocyclic reaction involving the indole 2,3-bond for the construction of an appropriate 4-oxygenated 2,3,4-trisubstituted carbazole ring 26, which was converted to calothrixin B (2) through condensation at N5 to C6.17 The Bennasar group19 used a regioselective intramolecular homolytic acylation at C13 to C13a. The 2-indolylacyl radical, generated from the phenylselenoester 33, underwent reaction to give the indolo[3,2-j]phenanthridine 39 in 90% yield. In 1995, a synthetic approach to the pentacyclic system of calothrixins using an electrocyclic reaction of 2,3-dialkenylindole, including the indole [b]-bond,27 was reported by the Srinivasan group.28 Recently, synthesis of a calothrixin B isomer with a novel 7H-indolo[2,3-j]phenanthridine-7,13(8H)- dione structure has been completed by the Gingant group employing a similar reaction.29
The first biomimetic total synthesis of calothrixin A and B was reported by our research group. Construction of a hypothetical metabolite, 5-formylindolo[2,3-a]carbazole ring 60 was carried out using an allene-mediated electrocyclic reaction involving two [b]-bonds of indoles.22 Oxidation of the fully MOM-protected 5-formylindolo[2,3-a]carbazole 60 with CAN provided N-MOM calothrixin B (2) in 40% yield. Moreover, the Moody group23,24 generated 5-formylindolo[2,3-a]carbazole 3 from indigo 6. Unfortunately, despite many attempts, oxidation of the non-protected carbazole 3 to give the pentacyclic calothrixin B (2) could not be achieved. Therefore, they employed our fully protected indolo[2,3-a]carbazole 60 as an intermediate for the synthesis of calothrixin A and B. Efficient oxidation of 60 was achieved using CAN, and subsequent condensation provided N-MOM calothrixin B (2). The biosynthetic route to calothrixins A (1) and B (2), proposed by Rickards group,1 has been proved by two independent research groups.
ACKNOWLEDGEMENTS
Our synthetic work, including a biomimetic route to calothrixins, was partly supported by Grant-in Aid for Scientific Research (C) from the Ministry of Education, Culture, Sports, Science and Technology of Japan. We thank to Miss. J. Nobuhiro, Mrs, S. Tohyama (Ms.) and A. Yamabuki (Ms.) as co-workers.
Dedicated to Dr. Keiichiro Fukumoto, HETEROCYCLES Editor, Emeritus Professor at Tohoku University on the occasion of his 75th birthday.
References
1. R. W. Rickards, J. M. Rothschild, A. C. Willis, N. M. de Chazal, J. Kirk, K. Kirk, K. J. Saliba, and G. D. Smith, Tetrahedron, 1999, 55, 13513. CrossRef
2. N. T. Doan, R. W. Rickards, J. M. Rothchild, and G. D. Smith, J. Appl. Phycol., 2000, 12, 409. CrossRef
3. N. T. Doan, P. R. Stewart, and G. D. Smith, FEMS Mocrobiol. Lett., 2001, 196, 135. CrossRef
4. P. H. Bernardo, C. L. L. Chai, G. A. Heath, P. J. Mahon, G. D. Smith, P. Waring, and B. A. Wilkes, J. Med. Chem., 2004, 47, 4958. CrossRef
5. P. H. Bernardo, C. L. L. Chai, M. Le Guen, G. D. Smith, and P. Waring, Bioorg. Med. Chem. Lett., 2007, 17, 82. CrossRef
6. X. X. Chen, G. D. Smith, and P. Waring, J. Appl. Phycol., 2003, 15, 269. CrossRef
7. G. Knübel, L. K. Larsen, R. E. Moore, I. A. Levine, and G. M. L. Patterson, J. Antibiot., 1990, 43, 1236.
8. C. Sanchez, C. Mendez, and J. A. Salas, Nat. Prod. Rep., 2006, 23, 1007. CrossRef
9. T. R. Kelly, Y. Zhao, M. Cavero, and M. Torneiro, Org. Lett., 2000, 2, 3735. CrossRef
10. (a) D. L. Comins and M. O. Killpack, J. Org. Chem., 1987, 52, 104; CrossRef (b) T. S. Work, J. Chem. Soc., 1942, 429. CrossRef
11. P. H. Bernardo, C. L. L. Chai, and J. A. Elix, Tetrahedron Lett., 2002, 43, 2939. CrossRef
12. P. H. Bernardo and C. L. L. Chai, J. Org. Chem., 2003, 68, 8906. CrossRef
13. (a) K. Hohenlohe-Oehringen, A. Rhomberg, and H. Bretschneider, Monatsh Chem., 1966, 97, 135; CrossRef (b) A. Godard and G. Queguiner, J. Heterocycl. Chem., 1980, 17, 465. CrossRef
14. D. Sissouma, S. C. Collet, and A. Y. Guingant, Synlett, 2004, 2612. CrossRef
15. D. Sissouma, L. Maingot, S. Collet, and A. Guingant, J. Org. Chem., 2006, 71, 8384. CrossRef
16. (a) K. Oikawa and O. Yonemitsu, J. Org. Chem., 1977, 42, 1213; CrossRef (b) S. Collet, J. F. Remi, C. Cariou, S. Laib, A. Guingant, N. Q. Vu, and G. Dujardin, Tetrahedron Lett., 2004, 45, 4911; CrossRef (c) N. Q. Vu, G. Dujardin, S. Collet, E. A. Reiber, A. Gingant, and M. Evain, Tetrahedron Lett., 2005, 46, 7669. CrossRef
17. S. Tohyama, T. Choshi, K. Matsumoto, A. Yamabuki, K. Ikegata, J. Nobuhiro, and S. Hibino, Tetrahedron Lett., 2005, 46, 5263. CrossRef
18. M. F. Saulnier and G. W. Gribble, J. Org. Chem., 1982, 47, 757. CrossRef
19. M.-L. Bennasar, T. Roca, and F. Ferrando, Org. Lett., 2006, 8, 561. CrossRef
20. P. H. Bernardo, W. Fitriyanto, and C. L. L. Chai, Synlett, 2007, 1935. CrossRef
21. T. Harayama, H. Akamatsu, K. Okamura, T. Miyagoe, T. Akiyama, H. Abe, and Y. Takeuchi, J. Chem. Soc., Perkin Trans. 1, 2001, 523. CrossRef
22. A. Yamabuki, H. Fujinawa, T. Choshi, S. Tohyama, K. Matsumoto, K. Ohmura, J. Nobuhiro, and S. Hibino, Tetrahedron Lett., 2006, 47, 5859. CrossRef
23. J. Sperry, C. S. P. McErlean, A. M. Z. Slawin, and C. J. Moody, Tetrahedron Lett., 2007, 48, 231. CrossRef
24. C. S. P. McErlean, J. Sperry, A. J. Blake, and C. J. Moody, Tetrahedron, 2007, 63, 10963. CrossRef
25. K. E. Shulte, J. Reisch, and U. Stoess, Arch. Pharmaz., 1972, 305, 523. CrossRef
26. M. Somei, F. Yamada, Y. Suzuki, S. Ohmoto, and H. Hayashi, Heterocycles, 2004, 64, 483. CrossRef
27. (a) S. Kano, E. Sugino, and S. Hibino, J. Chem. Soc., Chem. Commun. 1980, 1241. ; CrossRef (b) S. Kano, E. Sugino, S. Shibuya, and S. Hibino, J. Org. Chem., 1981, 46, 3856; CrossRef (c) S. Hibino, A. Tonari, T. Choshi, and E. Sugino, Heterocycles, 1993, 35, 441. CrossRef
28. A. K. Mohanakrishnan and P. C. Srinivasan, J. Org. Chem., 1995, 60, 1939. CrossRef
29. L. Maingot, F. Thuaud, D. Sissouma, S.Collet, A. Gingant, and M. Evain, Synlett, 2008, 263. CrossRef