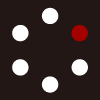
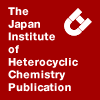
HETEROCYCLES
An International Journal for Reviews and Communications in Heterocyclic ChemistryWeb Edition ISSN: 1881-0942
Published online by The Japan Institute of Heterocyclic Chemistry
e-Journal
Full Text HTML
Received, 26th May, 2008, Accepted, 27th June, 2008, Published online, 30th June, 2008.
DOI: 10.3987/COM-08-S(F)20
■ An Improved Synthesis of 1-epi-ED-71, a Biologically Interesting Diastereomer of 1α,25-Dihydroxy-2β-(3-hydroxypropoxy)vitamin D3 (ED-71)
Kohei Eto, Ayako Fujjiyama, Mai Kaneko, Keisuke Takahashi, Jun Ishihara, Susumi Hatakeyama, Yoshiyuki Ono, and Noboru Kubodera*
Chugai Pharmaceutical Company, Ltd., 2-1-1, Nihonbashi-Muromachi, Chuo-ku, Tokyo 103-8324, Japan
Abstract
A linear synthesis and an improved convergent synthesis of biologically interesting 1-epi-ED-71, a diastereomer of 1α,25-dihydroxy-2β-(3-hydroxypropoxy)vitamin D3 (ED-71) at the 1-position of the A-ring, are described.INTRODUCTION
In Japan, active vitamin D3 (calcitriol, 1), a hormonally active form of vitamin D3, and its synthetic prodrug (alfacalcidol, 2) have been widely used for the treatment of osteoporosis for more than 25 years.3 Calcitriol (1) and alfacalcidol (2) have been recognized as very safe medicines showing mild or moderate increase in bone mineral density (BMD) in osteoporotic patients. In the US and Europe, on the other hand, bisphosphonates such as sodium alendronate, sodium risedronate and sodium ibandronate, are mainly prescribed for treating osteoporosis because of strong increment of BMD.4 Therefore, there is intense interest in obtaining active vitamin D3 analogs more potent than calcitriol (1)/alfacalcidol (2) or comparable to bisphosphonates in increasing BMD and preventing bone fracture with the objective of treating osteoporosis. 1α,25-Dihydroxy-2β-(3-hydroxylpropoxy)vitamin D3 (ED-71, 3), an analog of calcitriol (1), which possesses a hydroxypropoxy substituent at the 2-position of the A-ring of 1, is such an analog that shows potent effects on bone therapy.5-7 ED-71 (3) has shown to be more effective than calcitrol (1) and alfacalcidol (2) in increasing BMD and mechanical bone strength in ovariectomized
model rats.8,9 Based on animal model experiments, phase III current clinical studies of ED-71 (3) as a
promising candidate for the treatment of osteoporosis are being successfully conducted in Japan in comparison with alfacalcidol (2). These studies will be completed at the end of this year after 3 years of medication of osteoporotic patients.10
The detailed mode of action of the enhanced activity of ED-71 (3) beyond calcitriol (1) and alfacalcidol (2) toward bone remains to be clarified. The long duration of 3 in the blood stream arises from its strong affinity for vitamin D binding protein (DBP) (2-fold ~ 4-fold v. 1) and might explain, in part, the enhanced biological effects of 3.11 We, therefore, were highly interested in an active vitamin D3 analog with strong affinity for DBP. It was reported that the epimerization of calcitriol (1) at the A-ring 1-position remarkably enhances the affinity for DBP. Norman and co-workers reported that 1-epi-calcitriol (4) shows a 65.7-fold increase in affinity for DBP as compared to 1.12 These findings stimulated our interest in the biological profile of epimerized ED-71 (3) at the 1-position namely, 1-epi-ED-71 (5), including DBP affinity and its effects on bone. Accordingly, we describe an initial linear synthesis of 1-epi-ED-71 (5) and a subsequent convergent approach to 5 as an improved method (Figure 1).
LINEAR SYNTHESIS OF 1-EPI-ED-71
The generally established synthetic procedure for the epimerization of the 1α- to 1β-hydroxy group of the A-ring of vitamin D3 analogs by manganese dioxide oxidation followed by reduction with
sodium borohydride (NaBH4) was unsuccessful in case of ED-71 (3) because of the steric hindrance attributed to the allylic alcohol moiety at the 1-position of 3.13 Therefore, we adopted a different
oxidation/reduction sequence of the 1-hydroxyl group of tetraol (7) which was obtained as a key intermediate for the synthesis of ED-71 (3) from lithocholic acid (6) in a 25-step sequence.5 Thus, the 5,7-diene moiety in 7 was protected as the 4-phenyl-1,2,4-triazoline-3,5-dione (PTAD) adduct (8), in 54% yield, which was oxidized with pyridinium dichromate (PDC) to provide a mixture of keto-alcohol (9) and keto-aldehyde (10) in 56% yield. The mixture was stereoselectively reduced to alcohol (11) with NaBH4 in 71% yield. The 5,7-diene system in 12 was regenerated in 68% yield with simply heating 11 in 1,3-dimethyl-2-imidazolidinone (DMI) at 140 °C.14 Finally 12 was converted to 1-epi-ED-71 (5) in only 8% yield (0.35 mg) by irradiation using a high pressure mercury lamp through Vycor filter in ethanol at 0 °C followed by thermal isomerization in refluxing ethanol. Since the amount of 1-epi-ED-71 (5) obtained in this sequence is very limited, only in vitro biological activity could be evaluated. As anticipated, 1-epi-ED-71 (5) showed enhanced affinity for DBP (1.6-fold v. ED-71).15 In order to conduct further in vivo evaluation of 5 using ovariectomized model rats, more efficient convergent synthetic route needed to be developed since the linear method was long and produced only low yields of 5 (Scheme 1).16
CONVERGENT SYNTHESIS OF 1-EPI-ED-71
Considering the potential clinical application of ED-71 (3) as a useful drug in the near future, we have been investigating a practical synthesis of 3 for industrial scale production that centers on a convergent approach.17,18 As previously reported, our improved convergent route to ED-71 (3) involves the Trost coupling reaction, in which ene-yne (15) served as the A-ring synthon and bromomethylene (17) served as the C/D-ring synthon. Coupling of these two synthons produced the triene system of vitamin D3.19,20 The synthesis of the (R)-isomer of ene-yne (15) proceeded via epoxide (14) which, in turn, was derived from the C2-symmetrical epoxide (13)21 in 9-steps (27% overall yield). The (R)-isomer (15) was produced as a separable diastereomeric mixture along with the (S)-diastereomer ene-yne (16) in a 3:2 ratio, respectively. Ene-yne (16) possessed the requisite stereochemistry for 1-epi-ED-71. Thus, upon treatment of ene-yne (16) and excess bromomethylene (17) (prepared from 25-hydroxyvitamin D3 as described before)18 in the presence of tetrakis(triphenylphosphine)palladium (0) [Pd(PPh3)4] and triethylamine (Et3N) in boiling toluene, the coupled product (18) was obtained as an inseparable mixture with recovered 17. The resulting mixture was desilylated using 47% hydrofluoric acid (HF) in acetonitrile (MeCN) at room temperature to afford 1-epi-ED-71 (5) in 37% yield from (16) (15.4 mg). Compared to the aforementioned linear synthesis, the present Trost-based convergent approach afforded an improved yield of 1-epi-ED-71(5) in sufficient amounts that allows for in vivo evaluation of 5 (Scheme 2).
CONCLUSION
A linear and convergent synthetic route to biologically interesting 1-epi-ED-71 (5) has been developed. Although the linear method provided 5 in poor overall yields and limited quantities, the convergent synthesis produced sufficient amounts of 5 for in vivo biological evaluation and represents an improved route. Further biological studies with 5 including effects on bone will be reported elsewhere.
EXPERIMENTAL
Anhydrous THF was purchased from Kanto Chemical Co., Inc., MeOH and EtOH were distilled from sodium, toluene was distilled from phosphorus pentoxide, and CH2Cl2, Et3N, DMF and pyridine were distilled from calcium hydride. All other purchased solvents and reagents were used without further purification. All reactions were carried out under an atmosphere of argon unless otherwise noted. Celite 545 was purchased from Nacarai Tesque Inc. All extracts were dried over magnesium sulfate and evaporated under reduced pressure with a rotary evaporator. Chromatographic purification was carried out with Silica Gel 60N Cat. No. 37560-84 purchased from Kanto Chemical Co., Inc., flash column chromatography with Silica Gel 60N Cat. No. 37563-84 from Kanto Chemical Co., Inc., and preparative thin layer chromatography (TLC) with Merck Kieselgel 60 PFR254 Art. 1.05744.0009 or Art. 1.05715.0009.
Optical rotations were measured with JASCO DIP-370 polarimeter. 1H and 13C NMR spectra were recorded on VARIAN Gemini-300 and Gemini-400 spectrometers using CDCl3 as a solvent. Chemical shifts are reported in parts per million (ppm) calibrated from CHCl3 (7.26 ppm) or tetramethylsilane (0.00 ppm) for 1H NMR and CDCl3 (77.1 ppm) for 13C NMR. Infrared (IR) spectra were obtained using JASCO FT/IR-5300, JEOL JIR-6000, and Hitachi 270-30 spectrophotometers. Mass spectra (MS) were measured with JEOL JMS-DX303 instrument. High resolution mass spectra (HRMS) were recorded on JEOL JMS-AX-500 and VG Auto Spec Q instruments. Ultra violet (UV) spectra were obtained with Shimadzu UV-1600PC instrument using EtOH as a solvent.
PTAD adduct of 2β-(3-hydroxypropoxy)-1α,3β,25-trihydroxycholesta-5,7-diene (8): To a stirred solution of 7 (54 mg, 0.110 mmol) in CH2Cl2 (10 mL), was added a solution of PTAD (19.5 mg, 0.110 mmol) in CH2Cl2 (1 mL). The resulting mixture was stirred at rt for 2 h and evaporated. The residue was purified by preparative TLC developed with CH2Cl2-EtOH (10:1) to give 8 (40 mg, 54%) as a white powder. 1H-NMR (CDCl3): δ 7.46-7.27 (5H, m), 6.38 (1H, d, J=8.0 Hz), 6.23 (1H, d, J=8.0 Hz), 4.00-3.60 (7H, m), 1.20 (6H, s), 1.04 (3H, s), 0.95 (3H, d, J=6.3 Hz), 0.80 (3H, s). MS (EI) m/z 654 (M+-PTAD).
PTAD adduct of 2β-(3-hydroxypropoxy)-1-oxo-3β,25-dihydroxycholesta-5,7-diene (9) and PTAD adduct of 2β-(2-formylethoxy)-1-oxo-3β,25-dihydroxycholesta-5,7-diene (10): To a stirred solution of 8 (40 mg, 0.060 mmol) in CH2Cl2 (13 mL), was added PDC (13 mg, 0.035 mmol) at rt. The resulting mixture was stirred at rt for 2 h, poured into H2O, extracted with AcOEt and evaporated. The residue was purified by preparative TLC developed with CH2Cl2-EtOH (10:1) to give 9 (7.0 mg, 18%) and 10 (14.0 mg, 38%) each as a colorless oil. 9: IR (neat): ν 3450, 2970, 1760, 1700, 1410, 1080, 760 cm-1. 1H NMR (CDCl3): δ 7.46-7.27 (5H, m), 6.54 (1H, d, J=8.2 Hz), 6.23 (1H, d, J=8.2 Hz), 4.70-4.64 (1H, m), 4.22 (1H, d, J=4.6 Hz), 3.86-3.67 (4H, m), 1.25 (3H, s), 1.21 (6H, s), 0.96 (3H, d, J=6.3 Hz), 0.81 (3H, s). MS (EI) m/z 488 (M+-PTAD), 119 (100%). 10: IR (neat): ν 3500, 3000, 1770, 1710, 1420, 1100, 760 cm-1. 1H NMR (CDCl3): δ 9.80 (1H, s), 7.46-7.27 (5H, m), 6.53 (1H, d, J=8.3 Hz), 6.23 (1H, d, J=8.3 Hz), 4.74-4.64 (1H, m), 4.22 (1H, d, J=4.6 Hz), 4.03-3.92 (2H, m), 1.28 (3H, s), 1.21 (6H, s), 0.96 (3H, d, J=6.3 Hz), 0.81 (3H, s). MS (EI) m/z 486 (M+-PTAD), 60 (100%).
PTAD adduct of 2β-(3-hydroxypropoxy)-1β,3β,25-trihydroxycholesta-5,7-diene (11): To a stirred solution of 9 (7.0 mg, 0.010 mmol) and 10 (14.0 mg, 0.021 mmol) in EtOH (1.2 mL), was added NaBH4 (1.2 mg, 0.032 mmol) at rt. The resulting mixture was stirred at rt for 2 h and evaporated. The residue was purified by preparative TLC developed with CH2Cl2-EtOH (10:1) to give 11 (15.0 mg, 71%) as a colorless oil. IR (neat): ν 3450, 2950, 1740, 1700, 1400, 1080, 910, 740 cm-1. 1H NMR (CDCl3): δ 7.47-7.26 (5H, m), 6.39 (1H, d, J=8.4 Hz), 6.23 (1H, d, J=8.4 Hz), 4.50-4.38 (1H, m), 4.04-3.94 (1H, m), 3.88-3.70 (5H, m), 1.20 (6H, s), 0.98 (3H, s), 0.95 (3H, d, J=6.3 Hz), 0.78 (3H, s). MS (EI) m/z 490 (M+-PTAD), 60 (100%).
2β-(3-Hydroxypropoxy)-1β,3β,25-trihydroxycholesta-5,7-diene (12): A mixture of 11 (15.0 mg, 0.022 mmol) in DMI (15 mL) was stirred at 140 °C for 3h. The mixture was extracted with AcOEt, washed with H2O and evaporated. The residue was purified by preparative TLC developed with CH2Cl2-EtOH (10:1) to give 12 (7.5 mg, 68%) as a colorless oil. IR (neat): ν 3380, 2950, 1470, 1380, 1080, 1020, 760 cm-1. 1H NMR (CDCl3): δ 5.70-5.62 (1H, m), 5.38-5.31 (1H, m), 4.01-3.58 (7H, m), 1.22 (3H, s), 1.02 (3H, s), 0.95 (3H, d, J=6.3 Hz), 0.60 (3H, s). MS (EI) m/z 490 (M+), 60 (100%). UV λmax: 294, 283, 272 nm.
(5Z,7E)-(1S,2R,3R)-2-(3-Hydroxypropoxy)-9,10-secocholesta-5,7,10(19)-triene-1,3,25-triol (5): A solution of 12 (4.4 mg, 0.009 mmol) in EtOH (200 mL) was irradiated 400W high pressure mercury lamp with a Vycor filter at 0 °C for 1.5 min, refluxed for 2 h and evaporated. The residue was purified by preparative TLC developed with CH2Cl2-EtOH (10:1) to give 5 (0.35 mg, 8%) as a white powder. IR (neat): ν 3400, 2930, 2850 cm-1. 1H NMR (CDCl3): δ 6.43 (1H, d, J=12.0 Hz), 6.03 (1H, d, J=12.0 Hz), 5.38 (1H, s), 5.08 (1H, s), 4.33-4.28 (1H, brs), 4.10-4.01 (1H, m), 3.91 (2H, t, J=5.4 Hz), 3.84 (2H, t, J=5.4 Hz), 3.64-3.58 (1H, m), 1.22 (6H, s), 0.94 (3H, d, J=6.5 Hz), 0.54 (3H, s). HRMS (EI) calcd for C30H50O5 (M+) 490.3658, found 490.3706. UV λmax: 264 nm, λmin: 227 nm.16
(3R,4R,5R)-4-(3-Triethylsilyloxypropoxy)-3,5-bis(triethylsilyloxy)oct-1-en-7-yne (15) and (3S,4R,5R)-4-(3-Triethylsilyloxypropoxy)-3,5-bis(triethylsilyloxy)oct-1-en-7-yne (16): To a stirred solution of trimethylsilylacetylene (0.5 mL, 3.0 mmol), was added n-BuLi (1.6 M solution in THF, 1.86 mL, 3.0 mmol) at -78 °C. The mixture was stirred at -78 °C for 30 min. To the stirred mixture, was added boron trifluoride etherate (BF3-OEt2 0.5 mL, 3.0 mmol) and 14 (200 mg, 0.56 mmol) in THF (2 mL). The stirring was continued at -78 °C for 1 h and at rt for 1 h. The mixture was quenched with saturated NaHCO3 (2 mL) diluted with CH2Cl2, washed with H2O and saturated NaCl and evaporated to give a residue (150 mg), which was used without further purification. A mixture of the residue (150 mg) and 10 M NaOH (3 mL) in MeOH (3 mL) was stirred at rt for 3 h. The mixture was evaporated, extracted with AcOEt. The aqueous layer was further evaporated and extracted with THF. The combined AcOEt and THF solution was washed with saturated NaCl and evaporated. The residue was chromatographed on silica gel. Elution with n-hexane-AcOEt (1:1-0:1) gave a colorless oil (110 mg). To a stirred solution of the colorless oil (110 mg) in CH2Cl2 (5 mL), were added Et3N (0.73 mL, 5.16 mmol) and triethylsilyl trifluoromethanesulfonate (TESOTf 0.48 mL, 2.57 mmol) at -40 °C. The stirring was continued at -40 °C for 2 h. After the addition of H2O, the mixture was extracted with CH2Cl2, washed with saturated NaCl, evaporated and chromatographed on silica gel. Elution with n-hexane gave a mixture (190 mg, 61 % yield from 14) as a colorless oil. The mixture was further chromatographed on silica gel. Elution with n-hexane-AcOEt (500:1-400:1) gave analytically pure 15 and 16 (60:40). 15: 1H NMR (CDCl3): δ 5.90 (1H, ddd, J=6.8, 10.8, 17.6 Hz), 5.24 (1H, d, J=17.6 Hz), 5.13 (1H, d, J=10.8 Hz), 4.21 (1H, t, J=6.8 Hz), 4.00-3.97 (1H, m), 3.75-3.66 (4H, m), 3.26 (1H, dd, J=2.4, 5.8 Hz), 2.46 (1H, ddd, J=2.8, 4.9, 17.1 Hz), 2.38 (1H, ddd, J=2.8, 7.3, 17.1 Hz), 1.93 (1H, t, J=2.8 Hz), 1.79 (2H, quin, J=6.4 Hz), 0.98-0.93 (27H, m), 0.66-0.56 (18H, m). 16: [α]D -2.00 ° (c 0.50, CHCl3). IR (neat): ν 1461, 1414, 1238, 1072, 1009 cm-1. 1H NMR (CDCl3): δ 5.90 (1H, ddd, J=6.9, 10.5, 17.4 Hz), 5.23 (1H, d, J=17.4 Hz), 5.15 (1H, d, J=10.5 Hz), 4.33-4.29 (1H, m), 3.88-3.65 (5H, m), 3.35 (1H, dd, J=1.2, 5.7 Hz), 2.57-2.40 (2H, m), 1.94 (1H, t, J=2.7 Hz), 1.76 (2H, quin, J=6.6 Hz), 1.00-0.93 (27H, m), 0.64 (18H, m). 13C NMR (CDCl3): δ 138.2, 115.9, 85.7, 82.1, 74.3, 70.9, 69.71, 69.67, 60.3, 33.7, 23.5, 6.9, 6.82, 6.75, 5.2, 5.0, 4.4. HRMS (EI) calcd for C29H60O4Si3 (M+) 556.3799, found 556.3796.
(5Z,7E)-(1S,2R,3R)-2-(3-Hydroxypropoxy)-9,10-secocholesta-5,7,10(19)-triene-1,3,25-triol (5): To a stirred mixture of 16 (78.4 mg, 0.141 mmol) and 17 (93 mg, 0.26 mmol) in toluene (2.6 mL) at rt, was added Et3N (1 mL). To the degassed resulting mixture, was added Pd(PPh3)4 (60 mg, 0.052 mmol). The resulting mixture was refluxed for 2 h, filtrated through Celite, evaporated and chromatographed on silica gel. Elution with n-hexane-AcOEt (10:1) gave a colorless mixture (70 mg) of 18 and 17, which was used without further purification. To the stirred mixture (70 mg) in MeCN (1.2 mL) at 0 °C, was added 47% HF (25 µL). The resulting mixture was stirred at rt for 1 h, basified with NaHCO3 (20 mg) at 0 °C, extracted with AcOEt and evaporated. The residue was purified by preparative TLC developed with AcOEt and subsequent HPLC (ODS-M-80) with MeCN-H2O (1:1) to give 5 (15.4 mg, 37% from 16) as a white powder, which was completely identical with the material obtained by the linear method.16
ACKNOWLEDGEMENTS
We are grateful to Professor David Horne of Division of Molecular Medicine, City of Hope for helpful suggestions and reading of the manuscript.
*This paper is dedicated to Dr. Keiichiro Fukumoto, Professor Emeritus of Tohoku University, on the occasion of his 75th birthday.
References
1. This forms part 35 of “Synthetic Studies of Vitamin D Analogs” by N. Kubodera at Chugai Pharmaceutical Co., Ltd. Part 34; A. Fujiyama, M. Kaneko, K. Takahashi, J. Ishihara, S. Hatakeyama, and N. Kubodera, Heterocycles, 2007, 71, 2263. CrossRef
2. A part of this work has appeared as a preliminary communication in reference 16.
3. R. Eastell and B. L. Riggs, ‘Vitamin D and Osteoporosis’ Vitamin D Second Edition, ed. by D. Feldman, J. W. Pike, and F. H. Glorieux, Elsevier Academic Press, Burlington, 2005, pp. 1101-1120. CrossRef
4. S. E. Papapoulos, ‘Bisphosphonates. Pharmacology and Use in the Treatment of Osteoporosis’ Osteoporosis, ed. by R. Marcus, D. Feldman, and J. Kelsey, Academic Press, San Diego, 1996, pp. 1209-1234.
5. K. Miyamoto, E. Murayama, K. Ochi, H. Watanabe, and N. Kubodera, Chem. Pharm. Bull., 1993, 41, 1111 and references cited therein.
6. Y. Ono, H. Watanabe, A. Shiraishi, S. Takeda, Y. Higuchi, K. Sato, N. Tsugawa, T. Okano, T. Kobayashi, and N. Kubodera, Chem. Pharm. Bull., 1997, 45, 1626.
7. Y. Ono, A. Kawase, H. Watanabe, A. Shiraishi, S. Takeda, Y. Higuchi, K. Sato, T. Yamauchi, T. Mikami, M. Kato, N. Tsugawa, T. Okano, and N. Kubodera, Bioorg. Med. Chem., 1998, 6, 2517. CrossRef
8. T. Kobayashi, T. Okano, N. Tsugawa, M. Murano, S. Masuda, A. Takeuchi, K. Sato, and Y. Nishii, Bioorg. Med. Chem. Lett., 1993, 3, 1815. CrossRef
9. Y. Uchiyama, Y. Higuchi, S. Takeda, T. Masaki, A. Shira-Ishi, A. Sato, N. Kubodera, K. Ikeda, and E. Ogata, Bone, 2002, 30, 583. CrossRef
10. T. Matsumoto, T. Miki, H. Hagino, T. Sugimoto, S. Okamoto, T. Hirota, Y. Tanigawara, Y. Hayashi, M. Fukunaga, M. Shiraki, and T. Nakamura, J. Clin. Endocrinol. Metab., 2005, 90, 5031. CrossRef
11. T. Okano, N. Tsugawa, S. Masuda, A. Takeuchi, T. Kobayashi, Y. Takita, and Y. Nishii, Biochem. Biophys. Res. Commun., 1989, 163, 1444. CrossRef
12. A. W. Norman, R. Bouillon, M. C. Farach-Carson, J. E. Bishop, L.-X. Zhou, I. Nemere, J. Zhao, K. R. Muralidharan, and W. H. Okamura, J. Biol. Chem., 1993, 268, 20022.
13. M. Sheves, N. Friedman, and Y. Mazur, J. Org. Chem., 1977, 42, 3597. CrossRef
14. N. Kubodera, K. Miyamoto, H. Watanabe, M. Kato, K. Sasahara, and K. Ochi, J. Org. Chem., 1992, 57, 5019. CrossRef
15. Relative binding affinity of calcitriol, ED-71 and 1-epi-ED-71 for DBP and vitamin D receptor (VDR) are as follows; DBP: calcitriol: 100, ED-71: 410, 1-epi-ED-71: 670 (normalized by the potency of calcitriol=100). VDR: calcitriol: 100, ED-71: 70, 1-epi-ED-71: 0.3 (normalized by the potency of calcitriol=100).
16. Y. Ono, H. Watanabe, A. Kawase, N. Kubodera, T. Okano, N. Tsugawa, and T. Kobayashi, Bioorg. Med. Chem. Lett., 1994, 4, 1523. CrossRef
17. S. Hatakeyama, A. Kawase, Y. Uchiyama, J. Maeyama, Y. Iwabuchi, and N. Kubodera, Steroids, 2001, 66, 267. CrossRef
18. J. Maeyama, H. Hiyamizu, K. Takahashi, J. Ishihara, S. Hatakeyama, and N. Kubodera, Heterocycles, 2006, 70, 295. CrossRef
19. B. M. Trost and J. Dumas, J. Am. Chem. Soc., 1992, 114, 1924. CrossRef
20. B. M. Trost, J. Dumas, and M. Villa, J. Am. Chem. Soc., 1992, 114, 9836. CrossRef
21. K. C. Nicolaou, D. P. Papahatjis, D. A. Claremon, R. L. Magolda, and R. E. Dolle, J. Org. Chem., 1985, 50, 1440. CrossRef