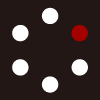
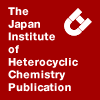
HETEROCYCLES
An International Journal for Reviews and Communications in Heterocyclic ChemistryWeb Edition ISSN: 1881-0942
Published online by The Japan Institute of Heterocyclic Chemistry
e-Journal
Full Text HTML
Received, 27th May, 2008, Accepted, 2nd July, 2008, Published online, 3rd July, 2008.
DOI: 10.3987/COM-08-S(F)22
■ Synthesis and Properties of Disk-Shaped Bipolar Symmetric Cyclic Triindole Derivative
Hidetaka Hiyoshi,* Tetsuya Aoyama, Tatsuo Wada, Takaaki Sonoda, and Shuntaro Mataka
Planning Section, Development Department, Ihara Chemical Industry Co., Ltd., 4-26 Ikenohata 1-chome, Taito-ku, Tokyo 110-0008, Japan
Abstract
The 1,3,4-oxadiazole-substituted donor-π-acceptor cyclic triindole, 5,10,15-tris(2-ethylhexyl)-2,7,12-tris-[4-(4-t-butylphenyl-1,3,4-oxadiazol-2-yl)- phenyl]-10,15-dihydro-5H-5,10,15-triazadiindeno[1,2-a;1’,2’-c]fluorene (3), showed an electroluminescence in a single-layer device which was fabricated by a spin-coating process.INTRODUCTION
Organic light emitting diodes (OLEDs) had seen increased to interest in investigating the use of nitrogen containing hetero aromatic compounds with either electron-donating or electron-withdrawing abilities. Single-layer OLED devices fabricated by a wet process have seen much attention due to a simple process and economical profits, and the preparation of high performance materials suitable for a wet process is essential for the development of OLEDs.1 Recently, we prepared the donor-π-acceptor type triindole, 5,10,15-tris- (2-ethylhexyl)-2,7,12-cyano-10,15-dihydro-5H-5,10,15-triazadiindeno-
[1,2-a;1',2'-c]fluorene2 (1) (Figure 1), which showed a relatively strong fluorescence due to intramolecular charge transfer (ICT) between the symmetric cyclic triindole ring of a strongly electron-donating nature and electron-withdrawing cyano groups at the terminal positions.3 A multi-layer electroluminescence (EL) device with 1 as a light-emitting material was fabricated, however, this device did not show any electroluminescence from triindole-layer. The device exhibited an emission from tris-(8-hydroxyquinolinato)aluminum (Alq3). These results suggest that 1 does not have electron-transporting and hole-blocking abilities. Therefore, 1 was considered unsuitable as an emitting material for a single-layer EL device.
In this paper, we designed a new donor-π-acceptor type triindole 3, which combined the 1,3,4-oxadiazole ring as an acceptor part and a symmetric triindole as a donor part. The 1,3,4-oxadiazole unit has been widely used as an electron-transporting hole-blocking (ETHB) material.4
RESULTS AND DISCUSSION
For producing a single layer EL device, highly efficient blue light emitting materials are needed to make a full-color EL display with a color filter. 5,10,15-Tris-(2-ethylhexyl)-2,7,12-tris-(4-cyanophenyl)- 10,15-dihydro-5H-5,10,15-triazadiindeno[1,2-a;1',2'-c]fluorene (2),3 which emitted a blue fluorescence (λem = 480 nm) with a moderate relative quantum yield (φ = 0.47), was then selected and evaluated as an emitting material for the OLEDs. However, 2 did not have any film forming ability based on the differential scanning calorimetry (DSC).
We designed a new triindole derivative 3 which might have good film morphology and show an electroluminescence even in a single-layer device. For improvement of the film forming ability, bulky alkyl groups were introduced to prevent aggregation in order to make allow the fabrication of the device by a spin-coating process. We then prepared a new donor-π-acceptor type triindole 3 by combining the 1,3,4-oxadiazole rings as the acceptor and the symmetric cyclic triindole ring as the donor part (Figure 3) by the Suzuki-Miyaura coupling reaction (Scheme 1 and 2).
A corresponding boronic acid was prepared by the reaction using bis(pinacolate)diborone under Miyaura reaction conditions in the presence of a palladium (II) catalyst (Scheme 1).5 The reaction of 2-(4-t-butylphenyl)-5-(4-bromophenyl)-1,3,4-oxadiazole6 (4) with bis(pinacolate)diborone produced 2-(4-t-butylphenyl)-5-[4-(4,4,5,5-tetramethyl-1,3,2-dioxobororan-2-yl)phenyl]-1,3,4-oxadiazole (5) in 45% yield. (Scheme 1)
The desired 1,3,4-oxadiazolyl triindole 3 was produced by the reaction of 5,10,15-tris-(2-ethylhexyl)- 2,7,12-tribromo-10,15-dihydro-5H-5,10,15-triazadiindeno[1,2-a;1',2'-c]fluorene (6), which was perpared by the cyclotrimerization reaction of N-(2-ethylhexyl)-5-bromoindolin-2-one,3 with 5 in refluxing toluene in the presence of a palladium (0) catalyst in 55% yield.
The absorption and fluorescence spectra of 1,3,4-oxadiazolyl triindole 3 are shown in Figure 4 and Table 1. The absorption spectrum of 3 exhibited two peaks, similarly to that of 3, around 310 and around 360 nm, respectively. These were assigned to the π → π* transitions (310 nm) and charge transfer transitions (360 nm), respectively. Although the π-conjugation system of 3 is longer than that of 2, the absorption of 3 (360 nm) showed only a slight red-shift (9 nm). This suggests that the phenyl groups of the triindole ring and the 1,3,4-oxadiazole ring were twisted around each other. The fluorescence of 3 was observed around 490 nm in CH2Cl2 and the relative quantum yield of 3 was determined to be 0.53 using quinine sulfate as the reference5 as shown in Table 1. Similar to the absorption spectrum, the fluorescence one of 3 showed a 10 nm red-shift. The relative quantum yield of 3 was the largest in the donor-π-acceptor type triindole derivatives.3
The electrochemical properties of 3 was studied by cyclic voltammetry measurements in CH2Cl2 in the presence of Bu4NPF6 as the supporting electrolyte using a glassy-carbon working electrode and Ag/AgCl couple in electrolyte solution as the reference at room temperature (Figure 5). Both the oxidation and reductive wave were observed. The oxidation potential (Eo) was evaluated at 0.51 V as a reversible process, however, the reduction potential (Epc) around -1.45 V was irreversible. It was supposed that the 1,3,4-oxadiazole side chain increased the electron affinity of 3 as expected. The irreversible wave in the reduction process was attributed to the poor stability of the radical anion.
The thermal property of 3 was investigated by differential scanning calorimetry (DSC). The glass transition temperature (Tg) was observed at 95 ℃ in the first cooling run. No peaks due to crystallization or melting were observed after the second heating and cooling process. These properties are favorable for fabricating an OLED device with 3 by a solution process.
Preliminary evaluations of the electroluminescent properties of 3 are shown in Table 2. The device was fabricated by a spin-coat method. A chloroform solution (3/CHCl3 = 15 mg/mL) of 3 was spin-coated on ITO, then dried in an oven at 50 ℃ under vacuo for 15 h. A blue EL spectrum was observed around 490 nm, in a single-layer device (device A), though very weak. The maximum luminance of 56 cd/m2 at 17 V was shown in device B. The EL peaks of these devices corresponded to the PL ones.
The HOMO energy level of 3 was determined to be -5.0 eV by ultraviolet photoelectron spectroscopy (UPS). The LUMO energy level of 3 was estimated to be -2.1 eV by combining the HOMO energy level together with the energy band gap obtained from the edge of the absorption spectrum (Figure 6). The HOMO energy level of 3 was relatively close to the Fermi level of the anode. On the other hand, the energy gap between the LUMO level of 3 and the cathode was large. Thus, holes were injected into the 1,3,4-oxadiazolyl triindole layer, while a sufficient injection of electrons was prevented.
CONCLUSIONS
The 1,3,4-oxadiazole-substituted triindole 3 was prepared by the Suzuki-Miyaura coupling reaction. Compound 3 had a good film-forming ability. Due to the donor-π-acceptor property, 3 exhibited reversible oxidation potential (E0) and irreversible reduction potential (Epc). The 1,3,4-oxadiazolyl triindole 3 showed an electroluminescence around 490 nm in the single-layer device fabricated by a spin-coating process. Based on this investigation, the 1,3,4-oxadiazole-substituted symmetric cyclic triindole 3 showed possibility as an EL material in a single-layer OLED device.
EXPERIMENTALS
General
All melting points are uncorrected. The 1H-NMR spectra were determined in CDCl3 using a VARIAN Mercury 300 spectrometer. Residual solvent protons were used as the internal standard and the chemical shifts (δ) are given relative to tetramethylsilane (TMS). The coupling constants (J) are reported in hertz (Hz). The elemental analyses were performed at the Elemental Analytical Center, Kyushu University. The electron impact mass spectrometry (EI-MS) spectra were recorded by a JEOL JMS-70 mass spectrometer at 70 eV using a direct inlet system. The fast atom bombardment mass spectrometry (FAB-MS) spectra were recorded by a JEOL JMS-70 mass spectrometer with m-nitrobenzyl alcohol (NBA) as the matrix. The UV-vis spectra were measured by a JASCO V-570 spectrophotometer in a 1.0 cm wide quartz cell (0.01 mM). The fluorescence spectra were measured by a HITACHI F-4500 fluorescence spectrophotometer. The cyclic voltammetry measurements were performed by a BAS model 600 electrochemical analyzer in deaerated dichloromethane solution (0.5 mM) containing tetrabutyl ammonium hexafluorophosphate (0.1 M) as the supporting electrolyte at 298 K (100 mV s-1). The glassy carbon working electrode was polished with a BAS polishing alumina suspension and rinsed with acetone before use. The counter electrode was a platinum wire. The measured potentials were recorded with respect to an Ag/AgCl (saturated KCl) reference electrode. The analytical TLC was carried out on a silica-gel coated glass plate (Merck 60 F254). Column chromatography was carried out on silica gel (KANTO 60N). The reagents were purchased from commercial suppliers and used without further purification.
Preparation of 2-(4-t-butylphenyl)-5-[4-(4,4,5,5-tetramethyl-1,3,2-dioxobroran-2-yl)phenyl]- 1,3,4-oxadiazole (5)
A mixture of potassium acetate (309 mg, 3.15 mmol), 2-(4-t-butylphenyl)-5-(4-bromophenyl)-1,3,4- oxadiazole 4 (1.07 g, 3 mmol), bispinacolate diborone (798mg, 3.14mmol), [1,1-bis(diphenylphosphino)-
ferocene]dichloro palladium (II) dichloromethane complex (73.5 mg, 0.09 mmol) and dimethyl sulfoxide (30 mL) was heated at 80 ℃ for 4 h. The reaction mixture was then cooled to rt, poured into water (300 mL) and extracted with EtOAc (200 mL). The organic layer was washed with brine (100 mL), dried over anhydrous MgSO4, and evaporated in vacuo to dryness. The residue was separated by silica gel column chromatography eluting with hexane/EtOAc to give 5 in 45% yield (540 mg).
White powder: mp 193.9-195.8 ℃: EI-MS; m/z 404 (M+): 1H-NMR;δ1.38 (s, 21H), 7.55 (d, J=8.7 Hz, 2H), 7.96 (d, J=8.7 Hz, 2H), 8.08 (d, J=8.7 Hz, 2H), 8.13(d, J=8.7 Hz, 2H).
Anal. Calcd for C24H29BN2O3: C, 71.30; H, 7.23; N, 6.93. Found: C, 70.80; H, 7.00; N, 7.07
Preparation of 5,10,15-tris-(2-ethylhexyl)-2,7,12-tris-[4-(4-t-buthylphenyl-1,3,4-oxadiazol-2-yl)- phenyl]-10,15-dihydro-5H-5,10,15-triazadiindeno[1,2-a;1',2'-c]fluorene (3)
To a mixture of 5,10,15-tris-(2-ethylhexyl)-2,7,12-tribromo-10,15-dihydro-5H-5,10,15-triazadiindeno- [1,2-a;1',2'-c]fluorene 6 (200 mg, 0.218 mmol), tetrakis(triphenylphosphine)palladium(0) (40 mg, 5 mol%) in toluene (40 mL), and saturated aqueous NaHCO3 (20 mL) was added 5 (600 mg, 1.49 mmol) in EtOH (5 mL) under a nitrogen atmosphere. The resulting mixture was heated at refluxing temperature for 4 h. The reaction mixture was poured into water (300 mL) and extracted with AcOEt (200 mL). The organic layer was washed with brine (100 mL), dried over anhydrous MgSO4, and evaporated in vacuo to dryness. The residue was separated by silica gel column chromatography eluting with hexane/EtOAc to give 3 in 55% yield (180 mg).
Yellow powder: mp 289 ℃: FAB-MS(NBA, positive), m/z 1511([M+H]+): 1H-NMR;δ0.60-0.40 (m, 9H), 0.70-0.60 (m, 9H), 1.20-0.70 (m, 24H), 1.40 (s, 27H), 2.10-2.00 (m, 3H), 5.02 (d, J=6.6 Hz, 6H), 7.59 (d, J=8.4 Hz, 6H), 7.71 (d, J=8.7 Hz, 3H), 7.78 (d, J=8.4 Hz, 3H), 7.98 (d, J=8.4 Hz, 6H), 8.13 (d, J=8.4 Hz, 6H), 8.31 (d, J=8.1 Hz, 6H), 8.54 (s, 3H).
Anal. Calcd for C102H111N9O3: C, 81.08; H, 7.40; N, 8.34. Found: C, 81.41; H, 7.37; N, 8.51
This paper is dedicated to Professor Emeritus Keiichiro Fukumoto on the occasion of his 75th birthday.
References
1. a) C. Huang, C.-G. Zhen, S. Ping, K. P. Loh, and Z.-K. Chen, Org. Lett., 2005, 7, 391; CrossRef b) Z. Ge, T. Hayakawa, S. Ando, M. Ueda, T. Akiike, H. Miyamoto, T. Kajita, and M. Kakimoto, Chem. Lett., 2008, 73, 294; CrossRef c) Z. Ge, T. Hayakawa, S. Ando, M. Ueda, T. Akiike, H. Miyamoto, T. Kajita, and M. Kakimoto, Chem. Mater., 2008, 20, 2532. CrossRef
2. H. Hiyoshi, H. Kumagai, and H. Ooi, International Application published under the Patent Cooperation Treaty (PCT), International Publication No.: WO/2005/077956..
3. H. Hiyoshi, H. Kumagai, H. Ooi, T. Sonoda, and S. Mataka, Heterocycles, 2007, 72, 231. CrossRef
4. a) F.-I. Wu, C.-F. Shu, C.-H. Chien, and Y.-T. Tao, Synthetic Metals, 2005, 148, 133; CrossRef b) G. Huges, and M. Bryce, J. Mater. Chem., 2005, 15, 94; CrossRef c) S. Oyston, C. Wang, G. Huges, A. S. Batsanov, I. F. Perepichka, M. R. Bryce, J. H. Ahn, C. Pearson, and M. C. Petty, J. Mater. Chem., 2005, 15, 194; CrossRef d) K. T. Kamtekar, C. Wang, S. Bettington, A. S. Batsnov, I. F. Perepichka, M. R. Bryce, J. H. Ahn, M. Rabinal, and M. C. Petty, J. Mater. Chem., 2006, 16, 3823. CrossRef
5. T. Ishiyama, M. Murata, and N. Miyaura, J. Org. Chem., 1995, 60, 7508. CrossRef
6. S. W. Cha, S.-H. Choi, K. Kim, and J.-I. Jin, J. Mater. Chem., 2003, 13, 479. CrossRef
7. a) S. Lacic and O. Inganäs, J. Appl. Phys., 2005, 97, 124901; CrossRef b) S. C. Tse, S. W. Tsang, and S. K. So, J. Appl. Phys., 2006, 100, 063708 CrossRef