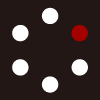
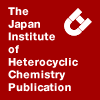
HETEROCYCLES
An International Journal for Reviews and Communications in Heterocyclic ChemistryWeb Edition ISSN: 1881-0942
Published online by The Japan Institute of Heterocyclic Chemistry
e-Journal
Full Text HTML
Received, 7th February, 2008, Accepted, 6th March, 2008, Published online, 7th March, 2008.
DOI: 10.3987/COM-08-S(F)3
■ Secoiridoid Di-glycosides from Osmanthus ilicifolius
Shigeaki Sakamoto, Koichi Machida, and Masao Kikuchi*
Department of 2nd Analytical Chemistry, Tohoku Pharmaceutical University, 4-4-1 Komatsushima, Aoba-ku, Sendai 981-8558, Japan
Abstract
Four new secoiridoid di-glycosides, 3’-O-β-D-glucopyranosyl ligustroside (1), 3’-O-β-D-glucopyranosyl 10-acetoxyligustroside (2), 3’-O-β-D- glucopyranosyl oleuropein (3) and 3’-O-β-D-glucopyranosyl 10-acetoxyole- uropein (4) were isolated from the leaves of Osmanthus ilicifolius. Their structures were established on the basis of chemical and spectral data. Furthermore, the structure of hiiragilide, previously elucidated to be 2’-O-β-D-glucopyranosyl 10-hydroxyligustroside, was revised as 3’-O-β-D-glucopyranosyl 10-hydroxyligustroside (5).As the part of our continued studies on the constituents of oleaceous plant, we previously reported the isolation and identification of two bis-iridoid glycosides1 along with three known secoiridoid glycosides2 and ten known lignan glycosides2,3 from the leaves of Osmanthus ilicifolius (Oleaceae: Japanese name, hiiragi). The leaves of this plant has been used in Japan as an herbal drug for vitiligo vulgaris.4 In the course of further studies on the constituents of this plant, four new secoiridoid di-glycosides have been isolated. This paper deals with the structural elucidation and identification of these compounds.
Furthermore, we previously reported the isolation of a secoiridoid di-glycoside, named hiiragilide, from the same plant and characterized as 2′-O-β-D-glucopyranosyl 10-hydroxyligustroside.5 In the present study, we have isolated one more secoiridoid di-glycoside (5), and direct comparison of the spectral data of the acetate (5a) and hiiragilide nonaacetate, led to the conclusion that the structure of hiiragilide should be revised to 3′-O-β-D-glucopyranosyl 10-hydroxyligustroside (5).
Compound 1 was obtained as an amorphous powder, [α]D –138.2 ° (MeOH). The molecular formula of 1, C31H42O17, was confirmed by HR-FAB-MS. The 13C NMR spectrum of 1 was almost identical to that of 6 (ligustroside) isolated from the same plant,5 except for the presence of an additional hexosyl moiety. In the 1H NMR spectrum of 1, the coupling constant of the anomeric proton signal of the additional hexosyl moiety was 7.8 Hz [δH 4.58 (H-1′′′)]. Acid hydrolysis proved that both of two sugars in 1 are D-glucose. The location of the additional β-D-gluco- pyranosyl moiety in 1 was deduced to be at 3′-OH of 6, because the signal due to C-3′ was markedly displaced downfield at δC 87.6 (+ 9.1 ppm), when comparing the 13C NMR spectrum of 1 with that of 6. This deduction was supported by the 1H-dedected heteronuclear multiple bond correlation (HMBC) between H-1′′′ and C-3′. Consequently, the structure of 1 was determined to be 3′-O-β-D-glucopyrano- syl ligustroside.
Compound 2 was obtained as an amorphous powder, [α]D –174.4 ° (MeOH). The molecular formula of 2, C33H44O19, was confirmed by HR-FAB-MS. The 13C NMR spectrum of 2 was almost identical to that of 7 (10-acetoxyligustroside) isolated from the same plant,5 except for the presence of an additional hexosyl moiety and difference in the chemical shift at C-3′ [δC 87.5 (+ 9.1 ppm)]. Acid hydrolysis proved that both of two sugars in 2 are D-glucose. The location of the additional β-D-glucopyranosyl moiety [δH 4.59 (1H, d, J=7.8 Hz, H-1′′′)] in 2 was deduced to be at 3′-OH of 7, because the 13C NMR chemical shifts due to glycosyl moieties in 2 was closely similar to that of 1. This deduction was supported by the HMBC between H-1′′′ and C-3′. Consequently, the structure of 2 was determined to be 3′-O-β-D-glucopyranosyl 10-acetoxyligustroside.
Compound 3 was obtained as an amorphous powder, [α]D –122.6 ° (MeOH). The molecular formula of 3, C31H42O18, was confirmed by HR-FAB-MS. Acid hydrolysis of 3 yielded D-glucose. The 1H and 13C NMR spectra of 3 were almost identical to those of 8 (oleuropein) isolated from the same plant,5 except for the presence of an additional β-glucopyranosyl group [δH 4.58 (1H, d, J=7.3 Hz, H-1′′′)]. The location of the additional β-D-glucopyranosyl moiety in 3 was deduced to be at 3′-OH of 8, because the 13C NMR chemical shifts due to glycosyl moieties in 3 was closely similar to that of 1. This deduction was supported by the HMBC between H-1′′′ and C-3′ (δC 87.6). Consequently, the structure of 3 was determined to be 3′-O-β-D-glucopyranosyl oleuropein.
Compound 4 was obtained as an amorphous powder, [α]D –125.0 ° (MeOH). The molecular formula of 4, C33H44O20, was confirmed by HR-FAB-MS. Acid hydrolysis of 4 yielded D-glucose. The 1H and 13C NMR spectra of 4 were almost identical to those of 9 (10-acetoxyoleuropein) isolated from the same plant,5 except for the presence of an additional β-glucopyranosyl group [δH 4.59 (1H, d, J=7.3 Hz, H-1′′′)]. The location of the additional β-D-glucopyranosyl moiety in 4 was deduced to be at 3′-OH of 9, because the 13C NMR chemical shifts due to glycosyl moieties in 4 was closely similar to that of 1. This deduction was supported by the HMBC between H-1′′′ and C-3′ (δC 87.5). Consequently, the structure of 4 was determined to be 3′-O-β-D-glucopyranosyl 10-acetoxyoleuropein.
We previously reported the isolation of a secoiridoid di-glycoside, named hiiragilide, from the same plant.5 In the present study, we have isolated one more secoiridoid di-glycoside (5: 3′-O-β-D-gluco- pyranosyl 10-hydroxyligustroside), and direct comparison of the spectral data of the acetate (5a) and hiiragilide nonaacetate, led to the conclusion that the two are identical. Accordingly, the structure of hiiragilide, previously elucidated to be 2′-O-β-D-glucopyranosyl 10-hydroxyligustroside, was revised as 5. The structure of 5 ([α]D –115.7°, C31H42O18) was determined as follows. Acid hydrolysis of 5 yielded D-glucose. The 1H and 13C NMR spectra of 5 were almost identical to those of 10 (10-hydroxy ligustroside) isolated from the same plant,5 except for the presence of an additional β-D-glucopyranosyl moiety [δH 4.59 (1H, d, J=7.8 Hz, H-1′′′)] and difference in the chemical shift at C-3′ [δC 87.6 (+ 9.1 ppm)]. The location of the additional β-D-glucopyranosyl moiety in 5 was deduced to be at 3′-OH of 10 by the HMBC between H-1′′′ and C-3′. The structure of 5 was determined to be 3′-O-β-D-glucopyranosyl 10-hydroxyligustroside.
The iridoid glycoside which comprised an oleoside moiety as a framework is called oleoside-type secoiridoid glycoside, and this type occurs only in Oleaceae plant. Most of them isolated so far are the 1-O-mono-glycoside. To our knowledge, this is the second report of an oleoside-type secoiridoid 1-O-di-glycoside.6
EXPERIMENTAL
General Optical rotation were taken with a JASCO DIP-360 digital polarimeter. UV spectra were recorded with a Beckman DU-64 spectrometer. The 1H and 13C NMR spectra were recorded with JEOL JNM-LA 400 (400 MHz, 100 MHz, respectively) spectrometer. Chemical shifts are given in a δ (ppm) scale with tetramethylsilane (TMS) as an internal standard. FAB-MS were recorded on a JEOL JMS-DX 303 mass spectrometer. Column chromatography was carried out on Kieselgel 60 (Merck; 230−400 mesh) and Sephadex LH-20 (Pharmacia Fine Chemicals). HPLC was carried out on a Tosoh HPLC system [pump, CCPS; detector, UV-8020; column, TSK gel ODS 120T (7.8 mm i.d. × 30 cm, Tosoh), TSK gel Amide-80 (7.8 mm i.d. × 30 cm, Tosoh) and Cosmosil 5SL (10 mm i.d. × 25 cm, Nacalai)].
Material The leaves of O. ilicifolius were collected in August, 2005 in Sendai, Miyagi prefecture, Japan, and identified by one of the authors (M. Kikuchi). A voucher specimen is held in the laboratory of M. Kikuchi.
Extraction and Isolation Fresh leaves of O. ilicifolius (2.6 kg) were extracted with MeOH at rt for eight months. The MeOH extract was concentrated under reduced pressure and the residue (466 g) was suspended in water. This suspension was successively extracted with CHCl3, AcOEt, n-BuOH and H2O. The n-BuOH-soluble fraction was concentrated under reduced pressure to produce a residue (167 g). The extract (74 g) was chromatographed on a silica gel column using CHCl3−MeOH−H2O (50 : 10 : 1, 30 : 10 : 1, 10 : 10 : 1) and the eluate was separated into five fractions (frs. 1−5). Fr. 2 was chromatographed on a Sephadex LH-20 column using 50 % MeOH and the eluate was separated into twelve fractions (frs. 2-1−2-12). Part of the fr. 2-6 (1.5 g) was subjected to preparative HPLC [column, TSK gel ODS 120T; mobile phase, MeOH−H2O (2 : 3); UV detector, 205 nm; flow rate, 1.5 mL / min; column temperature, 40 °C] to give ten peaks (peaks 1−10). Peak 2 was subjected to preparative HPLC [column, TSK gel Amide-80; mobile phase, MeCN−H2O (9 : 1); UV detector, 205 nm; flow rate, 1.5 mL / min; column temperature, 40 °C] to give 10 (40.8 mg) and 5 (2.5 mg). Peak 3 was subjected to preparative HPLC [column, TSK gel Amide-80; mobile phase, MeCN−H2O (9 : 1); UV detector, 205 nm; flow rate, 1.5 mL / min; column temperature, 40 °C] to give 9 (70.8 mg) and 4 (7.0 mg). Peak 4 was subjected to preparative HPLC [column, TSK gel Amide-80; mobile phase, MeCN−H2O (9 : 1); UV detector, 205 nm; flow rate, 1.5 mL / min; column temperature, 40 °C] to give 8 (21.3 mg) and 3 (2.8 mg). Peak 8 was subjected to preparative HPLC [column, Cosmosil 5SL; mobile phase, CHCl3−MeOH-H2O (50 : 10 : 1); UV detector, 230 nm; flow rate, 1.5 mL / min; column temperature, 40 °C] to give 7 (305.0 mg) and 2 (30.0 mg). Peak 10 was subjected to preparative HPLC [column, Cosmosil 5SL; mobile phase, CHCl3−MeOH−H2O (50 : 10 : 1); UV detector, 230 nm; flow rate, 1.5 ml / min; column temperature, 40 °C] to give 6 (40.8 mg) and 1 (3.3 mg).
3′-O-β-D-Glucopyranosyl ligustroside (1) An amorphous powder; [α]D27 –138.2 ° (c 0.13, MeOH); UV λmax (MeOH) nm (log ε): 226 (4.2), 240sh (4.1), 276 (3.3); FAB-MS m/z: 709 [M+Na]+; HR-FAB-MS m/z: 709.2318 (Calcd for C31H42O17Na, 709.2320); 1H NMR (CD3OD) δ: 1.64 (3H, dd, J=7.3, 1.5 Hz, H3-10), 2.44 (1H, dd, J=14.1, 9.3 Hz, H-6A), 2.70 (1H, dd, J=14.1, 4.4 Hz, H-6B), 2.82 (2H, br t, J=6.8 Hz, H2-β), 3.53 (1H, dd, J=8.8, 7.8 Hz, H-2′), 3.61 (1H, dd, J=8.8, 8.3 Hz, H-3′), 3.63 (1H, dd, J=11.7, 5.9 Hz, H-6′A), 3.67 (1H, dd, J=12.2, 6.0 Hz, H-6′′′A), 3.71 (3H, s, 11-CO2CH3), 3.89 (1H, dd, J=11.7, 2.4 Hz, H-6′B), 3.90 (1H, dd, J=12.2, 2.0 Hz, H-6′′′B), 3.97 (1H, dd, J=9.3, 4.4 Hz, H-5), 4.10 (1H, dt, J=10.7, 6.8 Hz, H-αA), 4.22 (1H, dt, J=10.7, 6.8 Hz, H-αB), 4.58 (1H, d, J=7.8 Hz, H-1′′′), 4.84 (1H, d, J=7.8 Hz, H-1′), 5.91 (1H, br s, H-1), 6.05 (1H, br q, J=7.3 Hz, H-8), 6.71 (2H, d, J=8.3 Hz, H-3′′, 5′′), 7.05 (2H, d, J=8.3 Hz, H-2′′, 6′′), 7.51 (1H, s, H-3); 13C NMR (CD3OD): Table 1.
3′-O-β-D-Glucopyranosyl 10-acetoxyligustroside (2) An amorphous powder; [α]D27 –174.4 ° (c 1.21, MeOH); UV λmax (MeOH) nm (log ε): 226 (4.6), 230sh (4.4), 276 (3.6); FAB-MS m/z: 767 [M+Na]+; HR-FAB-MS m/z: 767.2358 (Calcd for C33H44O19Na, 767.2374); 1H NMR (CD3OD) δ: 2.01 (3H, s, 10-COCH3), 2.49 (1H, dd, J=15.1, 9.8 Hz, H-6A), 2.77 (1H, dd, J=15.1, 3.9 Hz, H-6B), 2.83 (2H, br t, J=6.8 Hz, H2-β), 3.54 (1H, dd, J=9.8, 7.8 Hz, H-2′), 3.62 (1H, br t, J=9.0 Hz, H-3′), 3.64 (1H, dd, J=11.7, 6.1 Hz, H-6′A), 3.69 (1H, dd, J=12.2, 5.9 Hz, H-6′′′A), 3.72 (3H, s, 11-CO2CH3), 3.89 (1H, dd, J=11.7, 2.2 Hz, H-6′B), 3.90 (1H, dd, J=12.2, 2.0 Hz, H-6′′′B), 4.00 (1H, dd, J=9.8, 3.9 Hz, H-5), 4.14 (1H, dt, J=10.7, 6.8 Hz, H-αA), 4.25 (1H, dt, J=10.7, 6.8 Hz, H-αB), 4.56 (1H, ddd, J=13.5, 6.1, 1.5 Hz, H-10A), 4.59 (1H, d, J=7.8 Hz, H-1′′′), 4.76 (1H, dd, J=13.5, 7.6 Hz, H-10B), 4.87 (1H, d, J=7.8 Hz, H-1′), 5.98 (1H, br s, H-1), 6.06 (1H, br dt, J=6.1, 1.5 Hz, H-8), 6.71 (2H, d, J=8.8 Hz, H-3′′, 5′′), 7.05 (2H, d, J=8.8 Hz, H-2′′, 6′′), 7.53 (1H, s, H-3); 13C NMR (CD3OD): Table 1.
3′-O-β-D-Glucopyranosyl oleuropein (3) An amorphous powder; [α]D27 –122.6 ° (c 0.11, MeOH); UV λmax (MeOH) nm (log ε): 202 (4.4), 230 (4.2), 280 (3.4); FAB-MS m/z: 725 [M+Na]+; HR-FAB-MS m/z: 725.2256 (Calcd for C31H42O18Na, 725.2268); 1H NMR (CD3OD) δ: 1.66 (3H, dd, J=7.3, 1.5 Hz, H3-10), 2.44 (1H, dd, J=14.1, 8.8 Hz, H-6A), 2.70 (1H, dd, J=14.1, 4.6 Hz, H-6B), 2.76 (2H, br t, J=6.8 Hz, H2-β), 3.52 (1H, dd, J=8.8, 7.8 Hz, H-2′), 3.61 (1H, br t, J=8.8 Hz, H-3′), 3.63 (1H, dd, J=11.7, 5.9 Hz, H-6′A), 3.69 (1H, dd, J=12.2, 5.4 Hz, H-6′′′A), 3.71 (3H, s, 11-CO2CH3), 3.89 (1H, dd, J=11.7, 2.4 Hz, H-6′B), 3.90 (1H, dd, J=12.2, 2.0 Hz, H-6′′′B), 3.97 (1H, dd, J=8.8, 4.6 Hz, H-5), 4.10 (1H, dt, J=10.7, 6.8 Hz, H-αA), 4.20 (1H, dt, J=10.7, 6.8 Hz, H-αB), 4.58 (1H, d, J=7.3 Hz, H-1′′′), 4.87 (1H, d, J=7.8 Hz, H-1′), 5.91 (1H, br s, H-1), 6.06 (1H, br q, J=7.3 Hz, H-8), 6.54 (1H, dd, J=8.3, 2.0 Hz, H-6′′), 6.66 (1H, d, J=2.0 Hz, H-2′′), 6.68 (1H, d, J=8.3 Hz, H-5′′), 7.51 (1H, s, H-3); 13C NMR (CD3OD): Table 1.
3′-O-β-D-Glucopyranosyl 10-acetoxyoleuropein (4) An amorphous powder; [α]D27 –125.0 ° (c 0.28, MeOH); UV λmax (MeOH) nm (log ε): 202 (4.5), 230 (4.2), 280 (3.5); FAB-MS m/z: 783 [M+Na]+; HR-FAB-MS m/z: 783.2339 (Calcd for C33H44O20Na, 783.2324); 1H NMR (CD3OD) δ: 2.02 (3H, s, 10-COCH3), 2.50 (1H, dd, J=14.6, 9.8 Hz, H-6A), 2.77 (1H, dd, J=14.6, 3.9 Hz, H-6B), 2.77 (2H, br t, J=6.8 Hz, H2-β), 3.54 (1H, dd, J=9.3, 7.8 Hz, H-2′), 3.62 (1H, dd, J=9.3, 8.3 Hz, H-3′), 3.64 (1H, dd, J=12.0, 5.8 Hz, H-6′A), 3.68 (1H, dd, J=12.2, 5.4 Hz, H-6′′′A), 3.72 (3H, s, 11-CO2CH3), 3.89 (1H, dd, J=12.0, 2.0 Hz, H-6′B), 3.90 (1H, dd, J=12.2, 2.0 Hz, H-6′′′B), 4.00 (1H, dd, J=9.8, 3.9 Hz, H-5), 4.14 (1H, dt, J=10.7, 6.8 Hz, H-αA), 4.22 (1H, dt, J=10.7, 6.8 Hz, H-αB), 4.58 (1H, ddd, J=13.7, 5.8, 1.5 Hz, H-10A), 4.59 (1H, d, J=7.3 Hz, H-1′′′), 4.77 (1H, dd, J=13.7, 7.8 Hz, H-10B), 4.88 (1H, d, J=7.8 Hz, H-1′), 5.97 (1H, br s, H-1), 6.07 (1H, br dt, J=7.8, 1.5 Hz, H-8), 6.54 (1H, dd, J=8.3, 2.4 Hz, H-6′′), 6.66 (1H, d, J=2.4 Hz, H-2′′), 6.68 (1H, d, J=8.3 Hz, H-5′′), 7.53 (1H, s, H-3); 13C NMR (CD3OD): Table 1.
Hiiragilide (5: 3′-O-β-D-Glucopyranosyl 10-hydroxyligustroside) An amorphous powder; [α]D27 –115.7 ° (c 0.10, MeOH); UV λmax (MeOH) nm (log ε): 225 (4.2), 235sh (4.0), 275 (3.3); FAB-MS m/z: 725 [M+Na]+; HR-FAB-MS m/z: 725.2290 (Calcd for C31H42O18Na, 725.2269); 1H NMR (CD3OD) δ: 2.49 (1H, dd, J=14.9, 9.8 Hz, H-6A), 2.72 (1H, dd, J=14.9, 4.4 Hz, H-6B), 2.82 (2H, br t, J=7.1 Hz, H2-β), 3.54 (1H, dd, J=8.8, 7.8 Hz, H-2′), 3.61 (1H, dd, J=9.3, 8.8 Hz, H-3′), 3.65 (1H, dd, J=11.7, 5.9 Hz, H-6′A), 3.66 (1H, dd, J=12.2, 6.3 Hz, H-6′′′A), 3.70 (3H, s, 11-CO2CH3), 3.89 (1H, dd, J=11.7, 2.5 Hz, H-6′B), 3.90 (1H, dd, J=12.2, 1.8 Hz, H-6′′′B), 3.94 (1H, dd, J=9.8, 4.4 Hz, H-5), 4.21 (4H, m, H2-10, H2-α), 4.59 (1H, d, J=7.8 Hz, H-1′′′), 4.86 (1H, d, J=7.8 Hz, H-1′), 5.96 (1H, br s, H-1), 6.13 (1H, br t, J=6.1 Hz, H-8), 6.71 (2H, d, J=8.3 Hz, H-3′′, 5′′), 7.04 (2H, d, J=8.3 Hz, H-2′′, 6′′), 7.52 (1H, s, H-3); 13C NMR (CD3OD): Table 1.
Acetylation of 5 Compound 5 (1.5 mg) was acetylated with Ac2O-pyridine in the usual manner to give 5a (2.0 mg). An amorphous powder; [α]D27 –101.9 ° (c 0.12, MeOH); UV λmax (MeOH) nm (log ε): 207 (4.1), 216sh (4.1), 226 (4.1); FAB-MS m/z: 1103 [M+Na]+; HR-FAB-MS m/z: 1103.3204 (Calcd for C49H60O27Na, 1103.3219); 1H NMR (CDCl3) δ: 2.01 (6H, s, COCH3), 2.00, 2.04, 2.05, 2.06, 2.08, 2.11 (each 3H, s, COCH3), 2.29 (3H, s, 4′′-COCH3), 2.41 (1H, dd, J=15.4, 9.3 Hz, H-6A), 2.75 (1H, dd, J=15.4, 3.9 Hz, H-6B), 2.90 (2H, br t, J=7.1 Hz, H2-β), 3.68 (1H, ddd, J=9.8, 4.1, 2.2 Hz, H-5′′′), 3.73 (1H, m, H-5′), 3.73 (3H, s, 11-CO2CH3), 3.95 (1H, dd, J=9.3, 3.9 Hz, H-5), 3.96 (1H, t, J=9.4 Hz, H-3′), 4.04-4.40 (6H, m, H2-α, H2-6′, H2-6′′′), 4.63 (1H, d, J=8.1 Hz, H-1′′′), 4.66 (1H, m, H-10A), 4.77 (1H, dd, J=13.4, 7.1 Hz, H-10B), 4.90 (1H, dd, J=9.5, 8.1 Hz, H-2′′′), 4.92 (1H, d, J=8.3 Hz, H-1′), 4.99-5.17 (4H, m, H-2′, 4′, H-3′′′, 4′′′), 5.68 (1H, br s, H-1), 5.97 (1H, br t, J=6.1 Hz, H-8), 7.01 (2H, d, J=8.5 Hz, H-3′′, 5′′), 7.19 (2H, d, J=8.5 Hz, H-2′′, 6′′), 7.44 (1H, s, H-3); 13C NMR (CDCl3) δ: 20.35, 20.37, 20.56, 20.58, 20.66, 20.90, 20.91, 21.12, 21.13 (COCH3), 30.9 (C-5), 34.3 (C-β), 39.9 (C-6), 51.6 (11-CO2CH3), 60.7 (C-10), 61.7 (C-6′), 61.8 (C-6′′′), 65.0 (C-α), 68.0 (C-4′), 71.2 (C-2′′′), 71.8 (C-5′), 72.2 (C-5′′′), 72.4 (C-2′), 72.9 (C-3′′′), 78.6 (C-3′), 92.6 (C-1), 96.8 (C-1′), 101.0 (C-1′′′), 108.5 (C-4), 121.6 (C-3′′, 5′′), 124.3 (C-8), 129.8 (C-2′′, 6′′), 131.0 (C-9), 135.2 (C-1′′), 149.4 (C-4′′), 152.8 (C-3), 166.4 (C-11), 168.9, 169.1, 169.30, 169.34, 169.6, 170.4, 170.5, 170.66 (COCH3), 170.7 (C-7).
Acid Hydrolysis of 1−5. Each of compounds (ca. 1.0 mg) was refluxed with 1M HCl (1 mL) for 5 h. The reaction mixture was neutralized with Ag2CO3 and filtered. The solution was concentrated in vacuo and dried to give a sugar fraction. The sugar fraction was analyzed by HPLC under the following conditions: column, TSK gel Amide-80 (7.8 mm i.d.× 30 cm, Tosoh); column temperature, 45 °C; mobile phase, MeCN−H2O (4 : 1); flow rate, 1.0 mL/min; chiral detection (JASCO OR-2090). Identification of D-glucose present in the sugar fraction was carried out by the comparison of the retention time and optical rotation with that of authentic sample; tR (min) 39.0 (D-glucose, positive optical rotation).
ACKNOWLEDGEMENTS
The authors are grateful to Mrs. S. Sato and T. Matsuki of their university for NMR and MS measurements.
This paper is dedicated to Professor Emeritus Keiichiro Fukumoto on the occasion of his 75th birthday.
References
1. S. Sakamoto, K. Machida, and M. Kikuchi, Heterocycles, 2007, 74, 937. CrossRef
2. S. Sakamoto, K. Machida, and M. Kikuchi, J. Tohoku Pharm. Univers., 2007, 54, in press.
3. S. Sakamoto, K. Machida, and M. Kikuchi, J. Nat. Med., 2008, 62, in press.
4. K. Asakura, ‘Newly Revised Wakan-Yaku,’ Ishikaku Publishers, Tokyo, 1980, p. 259.
5. M. Kikuchi and Y. Yamauchi, Yakugaku Zasshi, 1985, 105, 442.
6. T. Tanahashi, Parida, Y. Takenaka, N. Nagakura, K. Inoue, H. Kuwajima, and C.-C. Chen, Phytochemistry, 1998, 49, 1333. CrossRef