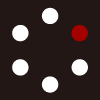
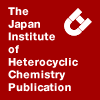
HETEROCYCLES
An International Journal for Reviews and Communications in Heterocyclic ChemistryWeb Edition ISSN: 1881-0942
Published online by The Japan Institute of Heterocyclic Chemistry
e-Journal
Full Text HTML
Received, 2nd June, 2008, Accepted, 20th June, 2008, Published online, 3rd July, 2008.
DOI: 10.3987/COM-08-S(D)1
■ Out-of-plane Deformation of the Azulene Ring along Its Short Molecular Axis in Crystal and Theoretical Structures of 1,3-Diheterolyl- and 1,3-Diphenylazulenes
Akira Ohta, Shigeyasu Kuroda,* Nguyen Chung Thana, Kouhei Terasawa, Kunihide Fujimori, Keita Nakajima, and Mitsunori Oda*
bDepartment of Applied Chemistry, Faculty of Engineering, University of Toyama, Gofuku 3190, Toyama, 930-8555 Japan
Abstract
Crystal structures of four simple azulene derivatives, 1,3-diheterolyl- and 1,3-diphenylazulenes, were determined by X-ray diffraction analysis. Three of them showed clear out-of-plane deformation of the azulene ring along its short molecular axis, and one showed a similar but very small deformation. Structural and conformational analyses on substituted azulenes were also performed by DFT molecular orbital calculations. Based on the results of the calculations it was indicated that azulenes could exhibit such deformation depending on their conformations except one case, supporting the results of X-ray analysis. A similar deformation was also found in the crystal structure of a previously reported 1,3-disubstituted azulene whose data were collected from an X-ray crystal structure database.INTRODUCTION
Azulene (1), an isomer of naphthalene, belongs to a typical non-alternant hydrocarbon and plays an important role in understanding the cyclic π-conjugation and aromaticity.1 In contrast to colorless naphthalene which is an isomer of azulene,2 it appears blue and hence has fascinated physical and synthetic organic chemists for a long time.3 Structural analysis of 1 based on X-ray and electron diffractions and molecular orbital calculations indicate its planarity and bond-convergence.4,5 Planarity of an aromatic ring can be disrupted by the circumstances around the ring, such as bulky substituents attached at the ring carbons as shown in structural analysis of the substituted acenes,6 bridging a ring with another carbocycle as observed frequently in cyclophanes,7 and molecular distortion as found in helicenes.8 The azulene ring in azulene derivatives similarly deforms; Itô and Fukazawa reported the bending of the azulene ring along its long molecular axis through the C2–C6 line in azulenophanes 2 and 3.9 The case of these azulenophanes is an example of aromatic compounds strained by a forced bridge. On the other hand, Hoppe et al. reported that the azulene derivative 4 showed an out-of-plane deformation along its short molecular axis through the C4–C8 line.10 Compound 4 has many substituents around the azulene ring and the Dreiding model study of 4 indicates that steric hindrance between the substituents is severe and the rotation of the phenyl rings at the five-membered ring is seriously restricted. Recently, Ito and Yamamura reported the distortion of the azulenic part in the helical structures of 5 and 6 (Figure 1).11
In this paper we report structural analyses of 1,3-diarylazulenes 7 (Figure 2), some of which show clear-cut out-of-plane deformation of the azulene ring along the short molecular axis. Note that these compounds in our study are azulene derivatives, which contain the rotary aryl groups at the peri positions of the azulene ring, and are structurally very different from 2–6. We also describe the results of DFT calculations, which predict that these derivatives show deformation depending on their conformations.12 It is also described that a similar distortion can be found in the crystal structure of an inclusion complex of a previously well-studied derivative 8,13 collected from an X-ray structure database,14 although the deformation was not commented in the original paper reporting the crystal structure of 8. Furthermore, we describe results of DFT calculations of unknown compounds (9, 10 and 11) related to 8 for prediction of planarity of their azulene ring.
RESULTS AND DISCUSSION
Synthesis and X-ray crystallographic analysis of 1,3-diarylazulenes.
Crystal structures of 1,3-diarylazulenes 7a–d were determined by X-ray diffraction analysis. Even though Lai et al. had recently reported X-ray crystallographic analysis of 7b,15 we independently analyzed its crystal structure.12 Both results were nearly identical. It is worth noting that Lai et al. did not claim any deformation of the azulene ring in their paper. The X-ray structure of 1,3-di(2-pyrrolyl)azulene (7e) was reported by Eichen et al.16 We have previously reported the syntheses of 7a,17 7b,18, 19 and 7c.19 Synthesis of 1,3-di(2-furyl)azulene (7d) was carried out by Negishi cross-coupling of 1,3-dibromoazulene (12)20 with 2-furylzinc chloride, as shown in Scheme 1.
Crystal data for 7a–d are summarized in Table 1. ORTEP drawings are shown in Figures 3–6. Crystal structures of 7a–c are asymmetrical, whereas 7d shows a C2 symmetrical structure. All of them hold bond-convergence of azulene and benzene rings. The bromophenyl and thienyl rings at the 1 and 3 positions in 7a and 7b connect to the azulenyl core with average dihedral angles of 35.9° and 38.3°, respectively.21
As shown in the side views of Figures 3–5, two phenyl rings of 7a and similarly two thienyl rings of 7b and 7c slant in different ways against the azulenyl plane. Both the sulfur atoms of the thienyl rings in 7b are located at the side of the 2 position of the azulene ring, while two 5-iodothienyl rings in 7c connect to the azulenyl core with different dihedral angles of 40.2° and 18.6°.21 One sulfur atom in 7c is located at the side of the 2 position and other at the side of the 4(8) position. Both crystal structures of 7a and 7b are similar to that of the most stable conformers calculated for 7f and 7b, respectively (vide ínfra), but that of 7c is different from the one calculated for 7b and has smaller inter-ring dihedral angles. In a crystal cell, 7c shows a weak intermolecular S···C interaction between one of the sulfur atoms and the carbon atom at the 3a position on the azulene ring of another molecule of 7c, with a distance of 3.444 Å. Since the
thienyl ring containing this sulfur atom is inverted and has a shallow dihedral angle, the S···C interaction seems to cause a structural change from the most stable conformer for 7c in the cell. The furan rings of 7d connect to the azulenyl core with an angle of 9.5° (Figure 6). Both the oxygen atoms in 7d are located at the side of the 4(8) position and direct toward up and down. The crystal structure of 7d is similar to that of the most stable conformer calculated. In the crystal structure of 7e, reported by Eichen et al., the planes of the two pyrrole rings are tilted slightly with a torsion angle of 29.1° (C2-C1-C2'-N1') and both N–H bonds point in the same direction.16 The hydrogens at the pyrrole nitrogen of 7e show NH–π interactions with electron-rich carbons of another azulene molecule in the cell. The azulene ring of 7e is planar. The azulene rings in the crystal structures of 7a–c show a clear out-of-plane deformation of the azulene ring along the short molecular axis, whereas 7d shows the similar but very small deformation. Out-of-plane deformation of an aromatic ring can be expressed better by a twist angle of a short molecular line against a long molecular line.22 Twist angles of the C3a–C8a, C4–C8, and C5–C7 lines against the C1-C3 line for 7a–e are shown in Table 2. The deformation of the azulene rings is much clearly shown by twist angles for 7a–c, among which the values for 7a were the largest. Deviations of the seven-membered ring carbons from the plane of C1–C2–C3 are shown in Figure 7, where plus values indicate that the carbons are in the front of the plane and minus values indicate that they are at the rear side of the plane. The carbon atoms at the 6' position in 7a and the 3' position in 7b and the sulfur atom at the 1' position in 7c are in the front of the plane, and the carbon atoms at the 6" position in 7a, the 3" position in 7b and the 3" position in 7c are at the rear side of the plane. Thus, the hydrogen atoms at the 4 and 8 positions of 7a are located away from the neighboring H6' and H6" hydrogen atoms, those of 7b are located away from the H3' and H3" hydrogen atoms, and those of 7c are located away from the S1' sulfur atom and H3" hydrogen atom. On the other hand, pyrrole rings in 7e slant in the same direction and the azulene ring is almost planar. Thus, these 4,8-hydrogens are located in an open space left by the slanting aryl planes in 7a–c. As mentioned above, the azulene ring of 7d is non-planar but the deformation is much smaller than those of 7a–c. Besides, the pattern of the deformation in 7d is different from those in 7a–c; the hydrogens at the 4 and 8 positions spread to the side of furan oxygen atoms. CH···O distances are estimated to be 2.205 and 2.228 Å, which are sufficiently short for these atoms to be able to interact. Therefore, the attractive CH···O interaction may deform the azulene ring. Since the deformation in 7d is small, further discussion should be based on more precise data, such as those measured at a lower temperature. As shown in these X-ray structures, it is important to account the molecular shapes on the basis of various possible conformations of these molecules.
Conformational analysis of 1-aryl- and 1,3-diarylazulenes based on DFT calculations
In order to gain a further insight into the relationship of rotation of aryl groups and the planarity of the azulene ring in these molecules, we examined conformational analysis based on density functional theory (DFT) calculations at the B3LYP/6–31+G(d,p) level of theory.23 The azulene derivatives 7a–e have two rotary aryl groups at the 1 and 3 positions and, therefore, many rotational isomers can be expected for 7a–e. To simplify the analysis, we first studied inter-ring torsional strain for azulenes with one substituent, 1-arylazulenes 13a–d (Figure 8). Figures 9–12 show the energy profiles, which were obtained by calculating the energy for each conformer upon changing the inter-ring dihedral angle (C(2)=C(1)–C=CX) of 13a (X=CH), 13b (X=S), 13c (X=O), and 13d (X=NH) by 5°. From these results, the most stable conformer of 13a is found to be one with a dihedral angel of 42.0°. The most stable conformer of 13b is found to be the one with a dihedral angel of 38.6°, and the sulfur atom located at the side of the 2 position, and the second most stable conformer of 13b is the one with a dihedral angel of 41.4° and the sulfur atom located at the side of the 4(8) position. The most stable conformer of 13c is found to be one with a dihedral angel of 0.1° and the oxygen atom located at the side of the 4(8) position. The second most stable conformer of 13c is one with a dihedral angel of 24.9° and the oxygen atom located at the side of the 2 position. Because steric hindrance between the hydrogen at the 4(8) position and the furan oxygen in 13c is expected to be smaller compared to other similar interactions in 13a–b and 13d,24 the compound 13c can have the most stable conformer with a nearly coplanar furan ring to the azulene ring. The most stable conformer of 13d is the one with a dihedral angel of 35.4˚ and the nitrogen atom located at the side of the 2 position. The second most stable conformer of 13d is the one with a dihedral angel of 34.6° and the nitrogen atom located at the side of the 4(8) position. The rotational
barriers of 13a–c and 13d are estimated as 4.93, 2.63, 4.24, and 2.16 kcal mol–1, respectively. Thus, these aryl groups are suggested to rotate freely at room temperature.25 With these results, we next attempted to generate possible conformers for 1,3-diarylazulenes, 7b and 7d–f.
DFT calculations generate several possible conformers for 7b and 7d–f 23; there are six conformers (A–F) for 7b and 7d–e and two conformers (A and D) for 7f (Figures 13–16). Conformers A–C have two aryl substituents slanting in the same way and conformers D–F have the two substituents slanting in different directions. Twist angles are listed showing the out-of-plane deformation for the conformers and the total energy relative to the most stable conformer in Table 3. First, we discuss conformation and planarity of 7b and 7e–f without 7d, since the reason of the deformation for 7d is different from those of others. Conformer 7fD is more stable and its structure is more similar to the X-ray structure of 7a than 7fA. 7bD
is the most stable conformer whose structure is similar to the X-ray structure of 7b. However, the crystal structure of 7e is not similar to the most stable conformer 7eD but to the third most stable conformer of 7eA. Further, the crystal structure of 7c is not similar to the most stable conformer 7bD but to the third most stable conformer of 7bF. The reason why these two crystal structures of 7c and 7e are not the most stable conformer based on calculations can be ascribed to their intermolecular interactions in their crystal cell (vide ánte). An important feature of conformers calculated is as follows: the optimized conformers D–F of 7b and 7e–f show clear out-of-plane deformation of their azulene rings, while the conformers
A–C show negligible twist angles and can be thought to have almost planar azulene rings. Results of these calculations support the experimental data not only in the pattern of the deformation of 7a–c but also in the planarity of the crystal structure of 7e. Therefore, it is best assumed that the conformers D–F have a the vacant space, where the H4 and H8 hydrogen atoms are able to spread their bonds, up and down against their mean azulene plane. The aryl groups can rotate around their affixing C–C single bonds in either solution or gas phase at ambient temperature, because the rotational barriers of 1-arylazulenes are estimated to be less than 5 kcal mol–1 based on results of DFT calculations (vide ánte).25 Thus, one may imagine that the molecules can evade the deformation by rotating the aryl rings. However, these calculations predict that the molecules can relieve the strain by slight out-of-plane deformation for themselves. In other words, we have become aware that an azulene ring can basically tolerate such a slight out-of-plane deformation and thus deform even under such circumstances without losing appreciable aromatic character.
Next, we discuss the theoretical and crystal structures of 7d. Among conformers 7dA–F, 7dE is the most stable one whose structure is not the same but most similar to the X-ray one of 7d. Energy differences between these conformers are smaller than those calculated for 7b, and 7e–f. The reason for these differences can be ascribed to less repulsive interactions between the furan oxygen and the hydrogen atoms at the 2, 4, and 8 positions, relative to the interaction between the C-H groups of the phenyl, the thienyl, and pyrrolyl substituents, the sulfur atom of the thienyl, or the N-H group of the pyrrolyl substituent and the hydrogen atoms. Different from cases of 7b and 7e–f, conformers A–B and E have a planar azulene ring, conformers C and F have a slightly deformed azulene ring, and conformer D shows clear out-of-plane deformation of the azulene ring in the case of 7d. Although the calculated structure of 7dE has a planar azulene ring, the X-ray structure of 7d shows slight out-of-plane deformation of the azulene ring along its short molecular axis. The deformation of the crystal structure of 7d is caused by an attractive interaction between the oxygen atoms of aryl substituents at the 1,3-positions and the hydrogen atoms at the 4,8-positions and, therefore, is fundamentally different from those of 7a–c, which are derived from a repulsive interaction. Besides planarity of the azulene ring, there are some differences between the most stable calculated and crystal structures; a torsion angle through C(2)–C(1)–C(2’)–O(1’) (13.8°) for the most stable calculated structure is greater than that (8.4°) for the crystal structure and its CH···O distance (2.240 Å) is slightly longer than those (2.205 and 2.228 Å) of the crystal structure. Thus, it is clear that there is discrepancy between the theoretical and crystal structures of 7d, probably because the attractive CH···O interaction, seen in the crystal structures of 7d, may be energetically underestimated in the calculations at the level applied.26
Out-of-plane deformation of an azulene derivative collected from an X-ray crystal structure database and conformational analysis of 1,3-bis(substituted methy)azulenes based on DFT calculations.
Various crystal structures of azulene derivatives were surveyed in our new finding of the out-of-plane deformation of the ring in simply substituted azulene derivatives.27 Using the ConQuest software containing crystallographic structure database, a crystal structure of 1,3-bis(trimethylammonio- methyl)azulene (8, diiodide salt) containing a guest molecule was found to show deformation depending on its conformation. The original paper was reported by Vögtle et al. in 1984 according to which the various onium salts with conformational flexibility can include low molecular weight guests in the host crystal lattice.28 They indicated that salt 8 in inclusion crystals could have two syn- and anti-conformation regarding a stereochemical relationship of two trimethylammonio groups. We found that the anti-conformer of 8 shows out-of-plane deformation along the short molecular axis, whereas the azulene ring of the syn-conformer is nearly planar. Twist angles of the two forms are listed in Table 4. Since the guest molecule is far from the azulene rings in these inclusion crystals, the phenomenon was thought to be intrinsic to the conformation of 8. Non-bonded distances between one of the hydrogens of the methylene group and the hydrogen at the 4(8) position is estimated to be 2.178 Å for anti-conformer and 2.046 Å for syn-conformer. These are sufficiently short for the hydrogen atoms to experience steric hindrance. In the structure of the anti-conformer, one pair of the methylene hydrogens is located on one side of the azulene plane and other pair is located on other side, whereas a pair of the methylene hydrogens is located on the same side of the azulene plane in the structure of the syn-conformer. Thus, the hydrogens at the 4(8) position of the anti-conformer are oriented up and down while the azulene plane distorts, similar to the cases of 7a–c. Thus, the anti-conformer avoids the steric hindrance by out-of
plane deformation along its short molecular axis. On the other hand, how does the syn-conformer avoid the steric hindrance? Two methylene carbon atoms at the 1,3-positions are displaced out-of-plane by 0.15 Å in the same direction. Further, the torsion angle evidences this displacement; the angle through C(3a)–C(8a)–C(1)–C(CH2) for the syn-conformer is 174.1°, though the angle for anti-conformer is 176.9°. Thus, the syn-conformer relieves the strain maintaining the aromatic ring by displacement of the substituents, whereas the anti-conformer relieves the strain by displacement of the aromatic ring.29 We also studied theoretical structures of the syn- and anti-conformers for 8 and structurally related compounds 9–11. Optimized syn- and anti-structures were illustrated in Figures 17–8. Twist angles and total energy of calculated conformers were listed in Table 5. Each anti-conformer has a lower total energy than syn-conformer in all cases of 8–11. Differences of energy between the syn- and anti-conformers of 10 and 11 are smaller than those of 8 and 9. The anti-conformers show clear out-of-plane deformation of the azulene ring along the short molecular axis and the syn-conformers show out-of-plane deformation of the substituents, partly supporting the crystal structural data for 8; two methylene carbon atoms at the 1,3-positions are displaced out-of-plane by 0.2 Å in the same direction and the angle through
C(3a)–C(8a)–C(1)–C(CH2) for the syn-conformer is 170.8°, though the angle for anti-conformer is 176.7°. Thus, it should be note that the syn-and anti-conformers differently relieve their strain and the way of anti-conformers to lower the energy is more effective than that of the syn-conformers.
CONCLUDING REMARKS
In summary, we have determined crystal structures of simply substituted azulene derivatives 7a–d and described new examples of out-of-plane deformation along its short molecular axis. Despite the inconsiderable forced strain, 7a–c relieve the strain by out-of-plane deformation for themselves without losing appreciable aromatic character. On the other hand, 7d deforms similarly but by a small amount by attractive interaction between the furan oxygen and the hydrogen atoms at the 4 and 8 positions, respectively. We have also demonstrated that DFT calculations approximately predict the deformation of 1,3-diarylazulenes, depending on their conformations, supporting the experimental result except the case of 7d. It is also described that a similar distortion depending on conformation was also found in previously disclosed X-ray structure of the derivative 8, collected from an X-ray structural database. Furthermore, we have predicted planarity of compounds 9–11, which are structurally related to 8.
EXPERIMENTAL
Melting points were measured on a Yanaco MP-3. IR spectra were recorded on a Perkin-Elmer Spectrum RX I spectrometer. UV spectra were measured on a Shimadzu UV-1600 spectrometer. 1H and 13C-NMR were recorded with tetramethylsilane as internal standard on a JEOL α400 NMR instrument. Mass spectra were measured on a JMS-700 mass spectrometer. Column chromatography was done with Wako activated alumina. THF was purified from benzophenone-sodium ketyl radical by distillation under a nitrogen atmosphere. Furan and an n-butyllitum hexane solution were purchased from Kanto Chem., Inc. and tetrakis(triphenylphosphine)palladium was purchased from Aldrich Co. A THF solution of 2-furylzinc chloride was prepared according to a literature procedure.30
Synthesis of 1,3-di(2-furyl)azulene (7d)
A mixture of 320 mg (1.12 mmol) of 1,3-dibromoazulene and 110 mg (0.089 mmol) of Pd(PPh3)4 was evacuated and replaced with argon gas (five times). To this mixture was added 15 mL (7.5 mmol) of 2-furylzinc chloride in THF solution (ca. 0.5 M) and this mixture was stirred at 60 °C under argon atmosphere. The reaction was monitored by TLC and the resulted dark reaction mixture was poured into 70 mL of 10% EDTA-NaHCO3 solution and extracted three times with 50 mL of Et2O. The combined organic layer was washed with brine and was dried with MgSO4. The solvent was removed and the residue was purified by alumina chromatography to give 163 mg of 7d as dark green solids. Mp 125-126 ˚C. 1H NMR (CDCl3) δ = 8.83 (d, J = 9.6 Hz, 2H, azulene H-4,8), 8.32 (s, 1H, azulene H-2), 7.58 (d, J = 1.6 Hz, 2H, furyl H-5’), 7.53 (t, J = 9.6 Hz, 1H, azulene H-6), 7.11 (t, J = 10.0 Hz, 2H, azulene H-5,7), 6.66 (d, J = 3.2 Hz, 2H, furyl H-3’), 6.54-6.55 (m, 2H, furyl H-4’) ppm; 13C NMR (CDCl3) δ= 151.9, 141.4, 139.6, 136.8, 135.7, 133.1, 124.4, 119.6, 111.4, 106.0 ppm; IR (KBr) νmax = 3447m, 3139w, 2369m, 2343m, 1846w, 1794w, 1774w, 1751w, 1735w, 1718m, 1702m, 1655m, 1637s, 1563s, 1499s, 1476w, 1458w, 1436m, 1387s, 1342m, 1234m, 1152m, 1012s, 970w, 882m, 855s cm-1; UV-Vis (CH2Cl2) λmax = 288 (log ε = 4.59), 314sh (4.42), 352 (4.19), 412 (3.92), 645 (2.47); MS (70eV) m/z (rel int) 261 (M++1, 20), 260 (M+, 100), 231 (22), 203 (17), 202 (33), 201 (8), 200 (9), 176 (5), 149 (5), 130 (7), 101 (6). Anal. Calcd for C18H12O2: C, 83.06; H, 4.65%. Found: C, 83.15; H, 4.87%.
X-Ray crystallographic analysis of 1,3-diarylazulenes
Measurements of 7a and 7d were performed by a Rigaku AFC7R diffractometer with graphite monochromated Mo-Ka radiation and a rotating anode generator. Those of 7b were carried out by a Rigaku R-AXIS IV imaging plate area detector and those of 7e were by a Rigaku RAXIS RAPID imaging plate area detector. All structures were solved by direct method and expand using Fourier techniques. Crystallographic data excluding structures have been deposited with the Cambridge Crystallographic Data Centre as supplementary publication numbers CCDC 288903 for 7a, CCDC 289169 for 7b, CCDC 671989 for 7c and CCDC 671990 for 7d, respectively. A copy of the data can be obtained free of charge from CCDC, 12 Union road, Cambridge CB2 1EZ. UK [DIRECT LINE: +44 1223 762910, Fax: +44 (0) 1223-336033 or e-mail: linstead@ccdc.cam.ac.uk; deposit@ccdc.cam.ac.uk. Unusual bond length and angle were not observed in crystal structures of 7a–d. No short contact between the intermolecular atoms was found for 7a and 7d. There is a short contact between one of the sulfur atoms and the hydrogen at 3' on the thienyl ring of another molecule with a distance of 2.997 Å in 7b, assumed to be a CH–S interaction. Also there is a short contact in 7c, assumed to be an S···C interaction, as described in a text. There is no disorder derived from crystal packing. Therefore, these deformations can only be ascribed to the intramolecular steric hindrance and the attractive interaction.
ACKNOWLEDGEMENTS
This research was carried out in part under financial support from the Faculty of Science at Shinshu University. We thank Dr. Ryuta Miyatake at the University of Toyama for his kind assistance in X-ray crystallographic analyses of 7a and 7d and also thank Dr. Mikio Yamasaki and Shin-ichi Sato at Rigaku Inc. for the X-ray crystallographic analysis of 7c.
References
1. For reviews on the azulenes, see; M. Gordon, Chem. Rev., 1952, 52, 127; CrossRef K. Hafner, Angew. Chem., 1958, 70, 419; CrossRef T. Nozoe and T. Asao, ‘Comprehensive Organic Chemistry (Dai-Yuki Kagaku),’ ed. by M. Kotake, ch. 16, pp. 439-464 and ch 17, pp. 465-686, Asakura Shoten, Tokyo, 1959; V. B. Mochali and Y. N. Porshnev, Russ. Chem. Rev., 1977, 46, 530; CrossRef D. Lloyd, ‘Non-benzenoid Conjugated Carbocyclic Compounds,’ pp. 352-377, Elsevier, Amsterdam, 1984; K.-P. Zeller, ‘Houben-Weyl; Methoden der Organischen Chemie,’ 4th ed. Vol. 5, Part 2C, ed. by Kropf, H., pp. 127-418, Georg Thieme, Stuttgart, 1985.
2. J. Michl and E. W. Thulstrup, Tetrahedron, 1976, 32, 205; CrossRef D. M. Lemal and G. D. Goldman, J. Chem. Educ., 1988, 65, 923; CrossRef R. S. H. Lui, J. Chem. Educ., 2002, 79, 183. CrossRef
3. R. S. H. Lui and A. E. Asato, J. Photochem. Photobiol., C, 2003, 4, 179. CrossRef
4. J. M. Robertson and H. M. M. Shearer, Nature, 1956, 177, 885; CrossRef J. D. Bernal, Nature, 1956, 178, 40; CrossRef Y. Takeuchi and R. Pepinsky, Science, 1956, 124, 126; CrossRef J. M. Robertson, H. M. M. Shearer, G. A. Sim, and D. G. Watson, Acta Crystallogr., 1962, 15, 1; CrossRef O. Bastiansen and J. L. Derissen, Acta Chim. Scand., 1966, 20, 1319. CrossRef
5. R. C. Haddon and K. Raghavachari, J. Am. Chem. Soc., 1981, 104, 3516; CrossRef R. C. Haddon and K. Raghavachari, J. Chem. Phys., 1983, 79, 1093; CrossRef J. M. L. Martin, J. El-Yazal, and J.-P. Francois, J. Phys. Chem., 1996, 100, 15358; CrossRef I. Bandyopadhyay, THEOCHEM. 2002, 618, 59. CrossRef
6. a) V. Balasubramaniyan, Chem. Rev., 1966, 66, 567; CrossRef b) A. Greenberg and J. F. Liebman, ‘Strained Oraganic Molecules,’ Academic Press: New York, 1978; Chapter 3, 3.H.2, pp. 148-170; c) J. F. Blount, F. Cozzi, J. R. Damewood, Jr., L. D. Iroff, U. Stöstrand, and K. Mislow, J. Am. Chem. Soc., 1980, 102, 99; CrossRef d) R. A. Pascal, Jr., W. D. McMillan, and D. Van Engen, J. Am. Chem. Soc., 1986, 108, 5652; CrossRef e) R. A. Pascal, Jr., W. D. McMillan, and D. Van Engen, J. Am. Chem. Soc., 1987, 109, 4660; CrossRef f) P. J. Fagan, M. D. Ward, J. V. Caspar, J. C. Calabrese, and P. J. Krusic, J. Am. Chem. Soc., 1988, 110, 2981. CrossRef
7. A. Greenberg and J. F. Liebman, ‘Strained Organic Molecules,’ Academic Press, New York, 1978, Chapter 3, 3.H.3, pp. 153-170; Y. Tobe, K. Ueda, K. Kakiuchi, and Y. Odaira, Tetrahedron, 1986, 42, 1851; CrossRef F. Bickelhaupt and W. H. d. Wolf, Recl. Trav. Chim. Pays-Bas, 1988, 107, 459; F. Bickelhaupt, Pure & Appl. Chem., 1990, 62, 373; CrossRef F. Bickelhaupt and W. H. d. Wolf, ‘Advances in Strain in Organic Chemistry,’ JAI Press, 1993, Vol. 3, pp. 185-227; V. V. Kane, W. H. d. Wolf, and F. Bickelhaupt, Tetrahedron, 1994, 50, 4575. CrossRef
8. R. A. Martin, Angew. Chem., Int. Ed. Engl., 1974, 13, 649; CrossRef K. P. Meurer and F. Vögtle, Top. Curr. Chem., 1985, 127, 1; CrossRef For most recent structure analysis of a helicene, see; D. J. Morrison, T. K. Trefz, W. E. Piers, R. McDonald, and M. Parvez, J. Org. Chem., 2005, 70, 5309. CrossRef
9. Y. Fukazawa, M. Aoyagi, and S. Itô, Tetrahedron Lett., 1978, 19, 1067; CrossRef Y. Fukazawa, M. Aoyagi, and S. Itô, Tetrahedron Lett., 1979, 20, 1055; CrossRef Y. Fukazawa, J. Tsuchiya, M. Sobukawa, and S. Itô, Tetrahedron Lett., 1985, 26, 5473; CrossRef Y. Fukazawa, M. Kodama, J. Tsuchiya, Y. Fujise, and S. Itô, Tetrahedron Lett., 1986, 27, 1929. CrossRef
10. N. Brodherr, P. Narayanan, K. Zechmeister, and W. Hoppe, Liebigs Ann. Chem., 1971, 750, 53. CrossRef
11. K. Yamamura, S. Kawabata, T. Kimura, K. Eda, and M. Hashimoto, J. Org. Chem., 2005, 70, 8902; CrossRef For other example of a non-planar azulene structure in helicene, see; S. Ito, A. Nomura, N. Morita, C. Kabuto, H. Kobayashi, S. Maejima, K. Fujmori, and M. Yasunami, J. Org. Chem., 2002, 67, 7295. CrossRef
12. A prelimnary account of this work has been appeared; A. Ohta, N. C. Thanh, K. Terasawa, K. Fijimori, S. Kuroda, and M. Oda, Tetrahedron Lett., 2006, 47, 2815; CrossRef We recently also reported that 1,2,3-trisubstituted azulene deforms similarly; S. Kuroda, Y. Obata, T. N. Chung, R. Miyatake, Y. Horino, and M. Oda, Tetrahedron Lett., 2008, 49, 552. CrossRef
13. F. Vögtle, H.-G. Löhr, H. Puff, and W. Schuh, Angew. Chem., Int. Ed. Engl., 1983, 22, 409; CrossRef H.-G. Löhr, A. Engel, H.-P. Josel, F. Vögtle, W. Schuh, and H. Puff, J. Org. Chem., 1984, 49, 1621. CrossRef
14. Structural search was done over database of the Cambridge Crystallographic Data Centre using the ConQuest interface.
15. F. Wang, M.-Y. Han, K. Y.; Mya, Y. Wang, and Y.-H. Lai, J. Am. Chem. Soc., 2005, 127, 10350. CrossRef
16. H. Salman, Y. Abraham, S. Tal, S. Meltzman, M. Kapon, N. Tessler, S. Speiser, and Y. Eichen, Eur. J. Org. Chem., 2005, 2207. CrossRef
17. M. Oda, N. C. Thanh, M. Ikai, T. Kajioka, H. Fujikawa, Y. Taga, S. Ogawa, H. Shimada, and S. Kuroda, Chem. Lett., 2005, 34, 754; CrossRef N. C. Thanh, M. Ikai, T. Kajioka, H. Fujikawa, Y. Taga, Y. Zhang, S. Ogawa, H. Shimada, Y. Miyahara, S. Kuroda, and M. Oda, Tetrahedron, 2006, 62, 11227. CrossRef
18. M. Oda, T. Kajioka, K, Haramoto, R. Miyatake, and S. Kuroda, Synthesis, 1999, 1349. CrossRef
19. M. Oda, N. C. Thanh, M. Ikai, H. Fujikawa, K. Nakajima, and S. Kuroda, Tetrahedron, 2007, 63, 10608. CrossRef
20. (a) A. G. Anderson, Jr., J. A. Nelson, and J. J. Tazuma, J. Am. Chem. Soc., 1953, 75, 4980; CrossRef (b) K. Hafner, H. Patzelt, and H. Kaiser, Liebigs Ann. Chem., 1962, 656, 24. CrossRef
21. These values were obtained as a dihedral angle between the mean planes of the azulene five-membered ring and the mean substituted aryl plane; 34.94° and 36.95° for 7a, 35.01° and 41.61° for 7b. Average torsion angles are 35.6° for 7a (36.48° for C2-C1-C1’-C2’ and 34.62° for C2-C3-C1"-C2"), 37.0° (40.05° for C2-C1-C2’-S1’ and 34.01° for C2-C3-C2"-S1") for 7b, 27.8° for 7c (38.5° for C2-C1-C2’-S1’ and 17.05° for C2-C3-C2"-C5"), and 9.65° for 7d (9.62° for C2-C1-C2’-C1’ and 9.67° for C2-C3-C2"-C1").
22. The twist angles were obtained by the way reported in the following reference; N. Symth, D. Van Eugen, and R. A. Pascal, Jr., J. Org. Chem., 1990, 55, 1937. CrossRef
23. We previously reported conformational analysis of 1,3-diarylazulene based on DFT calculations (ref. 12), which were performed using the Mulliken (ver. 2.0.0, IBM Inc.) program. All possible conformers were not necessarily generated in the previous study. DFT calculations of 1-aryl- and 1,3-diarylazulene in this paper were conducted by using the Gaussian 03, Revision D.01 program (Gaussian, Inc., Pittsburgh PA, 2003) to generate all possible conformers. Structures were optimized at B3LYP/6-31+G(d,p) level of theory and the energies were obtained at B3LYP/6-311+G(2d,p) level by one-point calculations.
24. The interaction between the hydrogen at the 4(8) position and the furan oxygen may rather be attractive as discussed on the crystal structure of 7d.
25. The free rotation is also supported by NMR experiments; the 1H-NMR spectra of 7a and 7b in CD2Cl2 at low temperature of -90°C did not show any intrinsic signal broadening based on the rotational process.
26. Calculations of 7dE at the MP2/6-31+G(d,p) level of theory predict so far an almost planar azulene ring, similarly shown in Table 3. Calculations at a higher level of theory are under progress.
27. The ConQuest program (version 1.9 [Build 4]. Copyright CCDC 2007) was used for the search.
28. H.-G. Löhr, H.-P. Josel, A. Engel, F. Vögtle, W. Schuh, and H. Puff, Chem. Ber., 1984, 117, 1487. CrossRef
29. Similar deformations were reported in substituted naphthalenes. See ref 6a.
30. A. Pelter, M. Rowlands, and G. Clements, Synthesis, 1987, 51 CrossRef