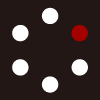
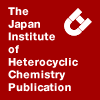
HETEROCYCLES
An International Journal for Reviews and Communications in Heterocyclic ChemistryWeb Edition ISSN: 1881-0942
Published online by The Japan Institute of Heterocyclic Chemistry
e-Journal
Full Text HTML
Received, 2nd July, 2008, Accepted, 6th August, 2008, Published online, 7th August, 2008.
DOI: 10.3987/COM-08-S(F)36
■ Pd(II)-Catalyzed Cyclization to Ether and Its Application to the Synthesis of the trans-Fused Polyether Core
Hajime Yokoyama, Satoshi Nakayama, Masayuki Murase, Masahiro Miyazawa, Seiji Yamaguchi, and Yoshiro Hirai*
Department of Chemistry, Graduate School of Science and Engineering, Toyama University, Gofuku 3190, Toyama 930-8555, Japan
Abstract
We employed an iterative Pd(II)-catalyzed cyclization reaction to ether from tri-O-acetyl-D-glucal to synthesize trans-fused polyethers. This approach should be applicable to synthesize the core structure of marine ladder toxins.Recently, many natural polyethers (eg.yessotoxin, Figure 1) were isolated from marine origin and these structures were determined by extensive endeavors.1 Especially the marine ladder toxins are very interesting, due to specific structure and biological activity.2-4 We have studied Pd(II)-catalyzed cyclization and its application to the synthesis of natural products.5,6 Herein we report the synthetic study of the trans-fused polyether core (polytetrahydropyran ring system (12)) by Pd(II)-catalyzed cyclization method.
This paper is dedicated to Professor Emeritus Keiichiro Fukumoto on the occasion of his 75th birthday.
The hydroboration of the alcohol (1), which was readily obtained by using a previously reported procedure from tri-O-acetyl-D-glucal (7steps),7 followed by Swern oxidation of the resulting alcohol afforded the aldehyde (2) (Scheme1). The coupling reaction of the aldehyde (2) and the propargyl alcohol derivative gave the alcohol (3) in 69% yield (2steps) as a mixture of stereoisomers (1:1). Deprotection of the TBS group of the alcohol (3) followed by hydrogenation of alkyne moiety with Lindlar catalyst gave the alcohol (4) in 70% yield from 3. The alcohol (4) was treated with a catalytic amount of PdCl2(MeCN)2 and benzoquinone in THF as a solvent at rt under an argon atmosphere to afford the alcohol (5) in 74% yield.8,5d Oxidation of the alcohol (5) followed by the reduction of the resulting ketone with NaBH4 afforded the alcohol (6a)9 and (6b) in 62% yield (2steps) (dr 89:11). The protection of the alcohol group of 6a with TBSCl in CH2Cl2 by using Et3N as a base gave the alcohol (7) in quantitative yield.
Next, the conversion of 7 into 12 was performed by a similar procedure(1.hydroboration of 7, 2.Swern oxidation of the resulting alcohol, 3. coupling of 8 with the propargyl alcohol derivative, 4.deprotection of TBS group, 5.hydrogenation of 9 with lindlar catalyst, 6.Pd(II)-catalyzed cyclization of 10, 7.oxidation of 11 with DMP, 8. reduction with NaBH4) (Scheme 2). After this conversion, polytetrahydropyran ring system (12) (the trans-fused polyether core) was obtained in 59% yield (2steps) as a 92:8 ratio. The stereochemistry of 12 was determined by extensive NMR experiment.10 Further studies are ongoing in our laboratory to synthesize the ABCD ring of yessotoxin.
In summary, we planed the iterative strategy of Pd(II)-catalyzed cyclization. To demonstrate this synthetic strategy, we tried the synthesis of the trans-fused polyether core. The optical starting material was treated by palladium-catalyzed cyclization method to give the tricyclic fused polyether core with high stereoselectivity. Finally we attained the synthesis of the trans-fused polyether core. The approach described could be easily extended towards synthesis of various the marine ladder toxin of potential biomedical application.
ACKNOWLEDGEMENTS
This work was supported by Grant-in Aid for Scientific Research on Priority Areas (18032032 & 20590101) from The Ministry of Education, Culture, Sports, Science and Technology (MEXT).
Reference 8
This paper is dedicated to Professor Emeritus Keiichiro Fukumoto on the occasion of his 75th birthday.
References
1. a) J. W. Blunt, B. R. Copp, M. H. G. Murro, P. T. Northcote, and M. R. Prinsep, Nat. Prod. Rep., 2004, 21, 1-49; CrossRef b) D. J. Faulkner, Nat. Prod. Rep., 2002, 19, 1-49. CrossRef
2. a) T. Yasumoto, Chem. Rec., 2001, 1, 228; CrossRef b) P. J. Scheuer, Tetrahedron, 1994, 50, 3. CrossRef
3. a) I. Kadota and Y. Yamamoto, Acc. Chem. Res., 2005, 38, 423; CrossRef b) T. Nakata, Chem. Rev., 2005, 105, 4314; CrossRef c) M. Inoue, Chem. Rev., 2005, 105, 4379. CrossRef
4. Selected iterative synthesis: a) B. M. Trost and Y. H. Rhee, Org. Lett., 2004, 6, 431; CrossRef b) Y. Mori, K. Yaegashi, and H. Furukawa, J. Am. Chem. Soc., 1996, 118, 8158; CrossRef c) N. Hori, H. Matsukura, G. Matsuo, and T. Nakata, Tetrahedron Lett., 1999, 40, 2811. CrossRef
5. a) P. J. Harirington, L. S. Hegedus, and K. F. McDaniel, J. Am. Chem. Soc., 1987, 109, 4335; CrossRef b) S. Saito, T. Hara, N. Takahashi, M. Hirai, and T. Moriwake, Synlett, 1992, 237; CrossRef c) H. Makabe, L. K. Kong, and M. Hirota, Org. Lett., 2003, 5, 27; CrossRef d) J. Uenishi, M. Ohmi, and A. Ueda, Tetrahedron: Asymmetry, 2005, 16, 1299. CrossRef
6. a) Y. Hirai, T. Terada, Y. Amemiya, and T. Momose, Tetrahedron Lett., 1992, 33, 7893; CrossRef b) Y. Hirai and M. Nagatsu, Chem. Lett., 1994, 21; CrossRef c) Y. Hirai, K. Shibuya, Y. Fukuda, H. Yokoyama, and S. Yamaguchi, Chem. Lett., 1997, 22; d) Y. Hirai, J. Watanabe, T. Nozaki, H. Yokoyama, and S. Yamaguchi, J. Org. Chem., 1997, 62, 776; CrossRef e) H. Yokoyama, K. Otaya, S. Yamaguchi, and Y. Hirai, Tetrahedron Lett., 1998, 39, 5971; CrossRef f) H. Yokoyama, K. Otaya, H. Kobayashi, M. Miyazawa, S. Yamaguchi, and Y. Hirai, Org. Lett., 2000, 2, 2427; CrossRef g) M. Miyazawa, Y. Hirose, M. Narantsetseg, H. Yokoyama, S. Yamaguchi, and Y. Hirai, Tetrahedron Lett., 2004, 45, 2883; CrossRef h) M. Miyazawa, M. Narantsetseg, H. Yokoyama, S. Yamaguchi, and Y. Hirai, Heterocycles, 2004, 63, 1017. CrossRef
7. K. C. Nicolaou, C.-K. Hwang, B. E. Marron, S. A. Defrees, E. A. Couladouros, Y. Abe, P. J. Carroll, and J. P. Snyder, J. Am. Chem. Soc., 1990, 112, 3040. CrossRef
8. We concluded the Pd(II)-catalyzed cyclization proceeded with high stereoselectivity because the alcohol (5) was oxidized by IBX to give the ketones as a >82 : 18 ratio(by NMR analysis). These good stereoselectivities can be explained by cyclic transition model as shown below (Figure 2). It is likely that two chair-form transition states are involved. The transition state A, leading to the major product, would be more stable than the transition state B, which is subject to steric repulsion between the π-allyloxy palladium complex and the axial proton on another ring.
9. The selected data for 6a: Rf = 0.60 (AcOEt); IR: 3600-3200, 1649cm-1; 1HNMR (600MHz, CDCl3) δ 5.84 (ddd, J=17.2, 10.6, 7.3 Hz, 1H), 5.43 (dt, J=17.2, 0.7Hz, 1H), 5.36 (dt, J=10.6, 0.7 Hz, 1H), 3.93-3.91 (m, 1H), 3.59-3.56 (m, 1H), 3.45 (ddd, J=11.4, 9.2, 4.4Hz, 1H), 3.39 (dt, J=11.4, 3.7Hz, 1H), 3.09-3.02 (m, 2H), 2.41 (ddd, J=11.4, 4.4, 4.4Hz, 1H), 2.11-2.07 (m, 1H), 1.74-1.71 (m, 2H), 1.58 (br s, 1H), 1.55-1.50 (m, 1H), 1.43 (ddd, J=23.1, 11.7, 5.5Hz, 1H).
10. The selected data for 12: Rf = 0.39 (AcOEt); IR: 3600-3200, 1647cm-1; 1HNMR (600MHz, CDCl3) δ 5.84 (ddd, J=17.6, 10.6, 7.3 Hz, 1H), 5.44 (dt, J=17.6, 1.5 Hz, 1H), 5.37 (dt, J=10.6, 1.5 Hz, 1H), 3.92 (ddt, J=1l.4, 4.0, 1.8 Hz, lH), 3.57 (dd, J=7.3Hz, 1H), 3.45 (ddd, J=11.0, 9.2, 4.8 Hz, lH), 3.39 (dt, J=11.4, 4.0 Hz, 1H), 3.17-3.11 (m, 2H), 3.09-3.03 (m, 2H), 2.45 (ddd, J=11.4, 4.0, 4.0 Hz, 1H), 2.34 (ddd, J=11.4, 4.0, 4.0 Hz, 1H), 2.10-2.06 (m, 2H), 1.75-1.72 (m, 3H), 1.55-1.51 (m, 2H).