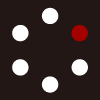
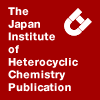
HETEROCYCLES
An International Journal for Reviews and Communications in Heterocyclic ChemistryWeb Edition ISSN: 1881-0942
Published online by The Japan Institute of Heterocyclic Chemistry
e-Journal
Full Text HTML
Received, 5th July, 2008, Accepted, 30th July, 2008, Published online, 31st July, 2008.
DOI: 10.3987/COM-08-S(F)40
■ Wittig Rearrangement of 3-Furylmethyl Ethers: Facile Synthesis of 3-Methyl-2-furylmethanols and 3-Furylethanols
Masayoshi Tsubuki,* Hiroyuki Okita, Kazunori Kaneko, Atsushi Shigihara, and Toshio Honda*
Faculty of Pharmaceutical Sciences, Hoshi University, 2-4-41 Ebara, Shinagawa-ku, Tokyo 142-8501, Japan
Abstract
Wittig rearrangement of 3-furylmethyl ethers 1a-i was investigated. Deprotonation of 3-furylmethyl ethers 1a-i with bases, such as BuLi and LDA, occurred preferentially at the allylic, propargylic, benzylic positions and α-position adjacent to carbonyl group giving the corresponding anions, which underwent 2,3- and 1,2-rearrangements to afford 3-methyl-2-furylmethanols 2a-i and 3-furylethanols 3a-f,h,i. Synthesis of naginata ketone and dendrolasin was achieved employing the Wittig rearrangement as a key step.INTRODUCTION
The synthesis of furan derivatives has attracted great interest because of the importance of natural and synthetic furans1 and the synthetic versatility of furans.2 Numerous efforts have been devoted to the preparation of furans and functionalization of the furan ring.3 As a part of our continuing work on the synthesis of biologically active natural compounds using furylmethanols, we were interested in developing a new method for the synthesis of furylmethanols. Previously, we have reported that the Wittig rearrangement of 2- and 3-furylmethyl ethers provides an efficient method for the preparation of 2-furylmethanol derivatives.4 Recently, we have successfully applied this rearrangement to the stereoselective synthesis of furanocyclic diterpene skeleton,5a steroidal side chain,5b and OSW-15c and its
thiazole analogue.5d In the present study we further investigate the Wittig rearrangement of 3-furylmethyl ethers and apply the rearrangement to the synthesis of naturally occurring terpenoids, naginata ketone and dendrolasin.
RESULTS AND DISCUSSION
The Wittig rearrangement of allyl 3-furylmethyl ether 1 could theoretically afford both 2,3-rearranged products 26 and 4 and 1,2-rearranged products 3 and 5 depending on the position, either α- or α’-position, of deprotonation (Scheme 1). We first evaluated the relative thermodynamic stabilities of anions 1α and 1α’ using ab initio calculations involving full optimizations with the GAUSSIAN 92 quantum mechanical package.7 The calculations show that the energy minimums of the 1a-d α anions were favored by 7.9-17.9 kJ/mol over the energy minimums of the 1a-d α’ anions at the RHF/6-31+G* level. This result suggests that the Wittig rearrangement of allyl 3-furylmethyl ethers 1a-d would proceed through deprotonation mainly at the α position, yielding 2a-d and 3a-d.
With this result in mind, the Wittig rearrangement of allyl 3-furylmethyl ether 1a was initially studied under standard conditions.8 Allyl 3-furylmethyl ethers 1a was prepared in 80% yield from by reaction of 3-furanmethanol with allyl bromide in DMF using 1.8 equiv. of NaH. The results of the rearrangement are shown in Table 1. Reaction of 1a with base brought about selective deprotonation at the expected α position to give 1α anion (R1=R2=R3=H), which went through sigmatropic rearrangement to afford α-ethenyl-3-methyl-2-furanmethanol 2a as a major product, together with α-ethenyl-3-furanethanol 3a. Treatment of 1a with 2 equiv. of n-BuLi in THF gave 2,3- and 1,2-rearranged products 2a and 3a in a ratio of ca. 2:1, and there remained 50% of starting material 1a (entry 1). In contrast, the reaction completed with 5 equiv. of n-BuLi (entry 2). The rearrangement proceeded at -30 to -20 °C when n-BuLi was employed as a base in THF. A large excess (10 equiv.) of LDA in THF gave a slightly higher ratio (ca. 2.6:1) than the ratio obtained by using n-BuLi (entry 4), whereas use of TMEDA (12 equiv.) as an additive in pentane-THF (v/v=9:1) resulted in the recovery of starting material again (entry 3). Treatment of 1a with s-BuLi (5 equiv.) in THF at -78 °C produced 2a and 3a in a ratio of ca. 2.3:1, which is similar to that obtained with LDA (entry 5). Fortunately, reaction of 1a with t-BuLi (4 equiv.) in THF at -78 °C furnished preferentially 2a (entry 6).
We next examined the Wittig rearrangement of 3-furylmethyl ethers 1b-i as shown in Table 2. Compounds 1b-i were prepared by the same method as above 1a. Reactions of 1b-f,h were conducted using n-BuLi (5 equiv.), s-BuLi (5 equiv.), and t-BuLi (4 equiv.) as a base in THF. LDA was employed for substrates 1g,i, and products were isolated as the corresponding methyl esters. Methallyl ether 1b gave preferentially 2,3-rearranged product 2b, together with 1,2-rearranged product 3b (entries 1-3), while crotyl ether 1c and prenyl ether 1d produced mainly 1,2-rearranged products 3c,d, respectively (entries 4-8). The effect of methyl group at the terminal alkene was apparent from the decreased reactivity toward BuLis; starting material 1c,d were recovered (entries 6 and 7) and reaction of 1d with t-BuLi was sluggish (entry 9). Rising temperature from -78 to 0 °C in the case of entry 9 brought about the formation of complex mixtures. Propargyl ether 1e gave predominantly 2e using s- and t-BuLi (entries 11 and 12), although treatment with n-BuLi gave almost equal amounts of 2e and 3e (entry 10). Reaction of benzyl ether 1f with n- and s-BuLi gave mostly 3f (entries 13 and 14), whereas reaction with t-BuLi afforded 2f as a major product (entry 15). 3-Furylmethyloxyacetic acid 1g gave cleanly 2,3-rearranged methyl ester 3g as a sole product (entry 16), whereas the corresponding propionic acid 1i gave equal amounts of 2i and 3i (entry 20). Compound 1h gave predominantly 1,2-rearranged product 3h (entries 17-19), in contrast allyl ether 1a gave mainly 2,3-rearranged product 2a (Table 1). The threo/erythro stereochemistries were tentatively assigned based on Nakai’s observation,9 in which the 1H NMR signal ascribed to CHOH in threo isomer is observed further upfield than that of erythro isomer. The threo isomer, the major product 3h, shows a triplet due to CHOH at δ 4.04 (J= 7.1 Hz), while erythro isomer presents a distorted triplet due to CHOH at δ 4.17 (J= 5.9 Hz).
We further applied this rearrangement to the synthesis of naturally occurring terpenoids dendrolasin10 and naginata ketone.11 The synthesis of dendrolasin was achieved as follows (Scheme 2). Etherification of 3-furanmethanol 6 with geranyl bromide in the presence of NaH gave geranyl ether 7 in 70% yield.
Treatment of 7 with n-BuLi (5 equiv.) gave almost starting material together with trace amount of 1,2-rearranged product 8, indicating lower reactivity of 7 toward BuLis. Next, excess n-BuLi (20 equiv.) was used to produce the desired product 8 in 27% yield together with starting material. Treatment of 7 with t-BuLi (5 equiv.) in THF at -78 to -25 °C afforded 8 in 51% yield and starting material. Pleasingly, reaction of 7 with s-BuLi (5 equiv.) at -78 °C in THF afforded 8 in 82% yield, although the reasons for the improvement of the yield are unclear. Attempts to convert 8 into dendrolasin by deoxygenation under Barton’s method12 were unsuccessful. Thus, alcohol 8 was acetylated to 9 in 91% yield, which was treated with lithium in EtNH213 to give dendrolasin 10 in 39% yield. The spectroscopic data obtained were identical with those reported.10c
Nginata ketone 11 was also prepared by MnO2 oxidation of 2d in poor yield, 11%, due to its high volatility (eq. 1).
Thus, we have disclosed the Wittig rearrangement of 3-furylmethyl ethers leading to 3-methyl-2-furylmethanols and 3-furylethanols. Regarding the formation of [1,2] rearranged product, the use of n-BuLi is superior to the use of s- and t-BuLi, whereas the use of t-BuLi is suitable for the formation of [2,3] rearranged product rather than the use of n- and s-BuLi. Synthesis of dendrolasin and naginata ketone has been succeeded in employing the Wittig rearrangement of 3-furylmethyl ethers as a key step.
EXPERIMENTAL
IR spectra were obtained using a JASCO FT/IR-200 spectrophotometer. 1H- and 13C-NMR spectra were obtained on a JEOL JNM-LA270 (1H-NMR: 270 MHz, 13C-NMR: 67.8 MHz) instrument for solutions in CDCl3, and chemical shifts are reported on the δ scale using TMS as an internal standard of δ 0.00 for 1H NMR spectra and CDCl3 as an internal standard of δ 77.00 for 13C NMR spectra, respectively. MS spectra were measured with a JEOL JMS-600 spectrometer. Elemental analyses were performed on a Yanaco-MT5.
General procedure for etherification of 3-furanmethanol
To a solution of allylic halide (36.7 mmol) and 3-furanmethanol (2.00 g, 20.4 mmol) in DMF (50 mL) was added NaH (ca. 60 % purity, 1.47 g, ca. 36.7 mmol) at 0 °C and stirring was continued for 8 h at rt. The reaction was carefully quenched with sat. aq. NH4Cl solution in ice bath. The reaction mixture was extracted with Et2O and CH2Cl2 (v/v, 2:1), and the combined organic layers were washed with brine. The organic layer was dried over Na2SO4 and evaporated to give a residue, which was chromatographed on silica gel (80 g, n-hexane/Et2O=95:5) to afford 3-furylnmethyl ether as a colorless oil.
Allyl 3-furylmethyl ether (1a)
80% yield; bp 70 °C (18 mmHg). IR 2860, 1500 cm-1; 1H-NMR δ 4.00 (2H, dt, J= 1.5 and 5.6 Hz), 4.39 (2H, d, J= 0.3 Hz), 5.20 (1H, dd, J= 1.5 and 10.4 Hz), 5.28 (1H, dd, J= 1.5 and 17.3 Hz), 5.93 (1H, ddt, J= 1.5, 10.4, and 17.3 Hz), 6.42 (1H, brs), 7.39-7.41 (2H, m); 13C-NMR δ 63.7, 71.3, 110.8, 117.6, 122.7, 135.1, 141.1, 143.7; MS (EI): 138 (M+); HRMS (EI) calcd for C8H10O2: 138.0681. Found: 138.0682. Anal. Calcd for C8H10O2: C, 69.54; H, 7.30. Found: C, 69.29; H, 7.39.
Methallyl 3-furylmethyl ether (1b)
88% yield; bp 75 °C (18 mmHg). IR 2850, 1500 cm-1; 1H-NMR δ 1.75 (3H, s), 3.90 (2H, s), 4.36 (2H, s), 4.91 and 4.98 (each 1H, each brs), 6.42 (1H, brs), 7.40 (2H, brd, J= 1.8 Hz); 13C-NMR δ 19.4, 63.0, 73.7, 110.2, 112.3, 122.3, 140.5, 142.0, 143.2; MS (EI): 152 (M+); HRMS (EI) calcd for C9H12O2: 152.0837. Found: 152.0854. Anal. Calcd for C9H12O2: C, 71.02; H, 7.95. Found: C, 71.32; H, 7.95.
Crotyl 3-furylmethyl ether (E/Z=82:18) (1c)
52% yield; bp 50 °C (16 mmHg). IR 2850, 1500 cm-1; 1H-NMR (E isomer) δ 1.72 (3H, dd, J= 1.2 and 6.1 Hz), 3.92 (2H, dd, J= 1.2 and 6.1 Hz), 4.36 (2H, brs), 5.54-5.79 (2H, m), 6.42 (1H, brs), 7.39-7.40 (2H, m); 13C-NMR (E isomer) δ 17.6, 62.9, 70.5, 110.3, 123.3, 127.4, 129.5, 140.5, 143.1; MS (EI): 152 (M+); HRMS (EI) calcd for C9H12O2: 152.0837. Found: 152.0842. Anal. Calcd for C9H12O2: C, 71.02; H, 7.95. Found: C, 71.35; H, 7.98.
Prenyl 3-furylmethyl ether (1d)
87% yield; bp 90 °C (15 mmHg). IR 2850, 1500 cm-1; 1H-NMR δ 1.66 and 1.75 (each 3H, each brs), 3.97 (2H, d, J= 6.7 Hz), 4.37 (2H, s), 5.34-5.40 (1H, m), 6.43 (1H, brs), 7.38-7.41 (2H, m); 13C-NMR δ 18.0, 25.7, 63.1, 66.3, 110.4, 120.9, 122.5, 137.2, 140.6, 143.2; MS (EI): 166 (M+); HRMS (EI) calcd for C10H14O2: 166.0993. Found: 166.0988. Anal. Calcd for C10H14O2: C, 72.26; H, 8.49. Found: C, 72.36; H, 8.56.
Propargyl 3-furylmethyl ether (1e)14
76% yield.
Benzyl 3-furylmethyl ether (1f)15
97% yield.
3-Furylmethoxyacetic acid (1g)
85% yield; bp 190 °C (9 mmHg). IR 3450, 3150, 1735 cm-1; 1H-NMR δ 4.13 (2H, s), 4.54 (2H, s), 6.45 (1H, d, J= 1.2 Hz), 7.42 (1H, d, J= 1.2 Hz), 7.45 (1H, brs); 13C-NMR δ 64.3, 65.9, 110.3, 120.7, 141.2, 143.6, 175.4; MS (EI): 152 (M+); HRMS (EI) calcd for C7H8O4: 152.0837. Found: 152.0854. Anal. Calcd for C7H8O4·0.2H2O: C, 52.73; H, 5.31. Found: C, 52.69; H, 5.27.
Allyl 1-(3-furyl)ethyl ether (1h)
68% yield; bp 98 °C (8 mmHg). IR 2860, 1500 cm-1; 1H-NMR δ 1.44 (3H, d, J= 6.1 Hz), 3.85 and 3.95 (each 1H, each ddt, J=1.2, 5.5, and 12.8 Hz), 4.47 (1H, q, J= 6.1 Hz), 5.15 (1H, dq, J= 1.2 and 10.4 Hz), 5.25 (1H, dq, J= 1.2 and 17.1 Hz), 5.90 (1H, ddt, J= 5.5, 10.4, and 17.1 Hz), 6.40 (1H, d, J= 1.8 Hz), 7.36 (1H, brs), 7.39 (1H, t, J= 1.8 Hz); 13C-NMR δ 22.2, 68.8, 68.9, 108.5, 116.6, 127.5, 135.0,139.4, 143.2; MS (EI): 152 (M+); HRMS (EI) calcd for C9H12O2: 152.0837. Found: 152.0839. Anal. Calcd for C9H12O2: C, 71.02; H, 7.95. Found: C, 70.23; H, 7.83.
2-(3-Furylmethoxy)propionic acid (1i)
76% yield; bp 180 °C (5 mmHg). IR 3460, 3150, 1730 cm-1; 1H-NMR δ 1.49 (3H, d, J= 7.5 Hz), 4.10 (1H, q, J= 7.5 Hz), 4.42 and 4.58 (each 1H, each d, J= 12.5 Hz), 6.45 (1H, brs), 7.40 (1H, brs), 7.45 (1H, brs), 9.58 (1H, brs); 13C-NMR δ 18.3, 63.2, 72.9, 110.3, 121.2, 141.0, 143.5, 178.5; MS (EI): 170 (M+); HRMS (EI) calcd for C8H10O4: 170.0579. Found: 170.0585. Anal. Calcd for C8H10O4: C, 56.46; H, 5.92. Found: C, 56.24; H, 5.96.
Geranyl 3-furylmethyl ether (7)
70% yield; bp 182 °C (6 mmHg). IR 2820, 1060 cm-1; 1H-NMR δ 1.60 and 1.65 (each 3H, each brs), 1.68 (3H, d, J= 1.2 Hz), 1.95-2.15 (4H, m), 4.00 (2H, d, J= 6.7 Hz), 4.37 (2H, s), 5.09 (1H, tt, J= 1.2 and 6.7 Hz), 5.37 (1H, dt, J= 1.2 and 6.7 Hz), 6.42 (1H, d, J= 1.8 Hz), 7.39 (2H, brd, J= 1.8 Hz); 13C-NMR δ 16.3, 17.5, 25.6, 26.2, 39.5, 62.9, 66.2, 110.3, 120.6, 122.4, 123.9, 131.5, 140.3, 140.5, 143.1; MS (EI): 234 (M+); HRMS (EI) calcd for C15H22O2: 234.1619. Found: 234.1619. Anal. Calcd for C15H22O2: C, 76.88; H, 9.46. Found: C, 76.63; H, 9.53.
General procedure for Wittig rearrangement of 3-furylmethyl ether
To a solution of 3-furylmethyl ether (1a-i, 7) (1.0 mmol) in THF (10 mL) was added dropwise a base (n-BuLi 1.6 M in hexane, 3.12 mL, 5.0 mmol; s-BuLi 1 M in cyclohexane, 5.0 mL, 5.0 mmol; t-BuLi 1.6 M in pentane, 2.5 mL, 4.0 mmol; LDA 4.0 mmol) at -78 °C under Ar. After stirring for 1 h (the reaction mixture was allowed to warm to 0 °C in the cases of n-BuLi), the reaction mixture was quenched with sat. aq. NH4Cl solution, and the solvent was removed under vacuum. The residue was extracted with pentane-Et2O (1:1, v/v). The extract was washed with brine and dried over Na2SO4. Evaporation of the solvent gave a residue, which was chromatographed on silica gel (50 g, n-hexane/AcOEt=95:5) to afford rearrangement products, respectively. Yields and the ratio of product distribution are shown in Tables 1 and 2. All the rearranged products were isolated by either careful column chromatography or derivatization.
α-Ethenyl-3-methyl-2-furanmethanol (2a)
Colorless oil; IR 3400, 2940, 990 cm-1; 1H-NMR δ 2.06 (3H, s), 5.20-5.23 (1H, m), 5.27 (1H, d, J= 12.2 Hz), 5.35 (1H, d, J= 17.1 Hz), 6.06-6.18 (1H, m), 6.20 (1H, d, J= 1.8 Hz), 7.28 (1H, d, J= 1.8 Hz); 13C-NMR δ 9.6, 67.1, 113.2, 115.6, 116.7, 137.1, 141.2, 149.1; MS (EI): 138 (M+); HRMS (EI) calcd for C8H10O2: 138.0681. Found; 138.0671.
α-Ethenyl-3-furanethanol (3a)
Colorless oil; IR 3380, 2930, 1020 cm-1; 1H-NMR δ 2.63 and 2.71 (each 1H, each dd, J= 7.3 and 14.6 Hz), 4.28 (1H, brd, J= 5.5 Hz), 5.15 (1H, dt, J= 10.4 Hz), 5.28 (2H, d, J= 17.1 Hz), 5.93 (1H, ddd, J=6.7, 10.4, and 17.1 Hz), 7.31 (1H, brs), 7.38 (1H, d, J=1.8 Hz); 13C-NMR δ 32.8, 72.4, 111.5, 115.2, 120.4, 140.1, 140.3, 143.0; MS (EI): 138 (M+); HRMS (EI) calcd for C8H10O2: 138.0681. Found; 138.0683.
3-Methyl-α-(1-methylethenyl)-2-furanmethanol (2b)
Colorless oil; IR 3410, 2950, 1010 cm-1; 1H-NMR δ 1.69 (3H, s), 2.06 (3H, s), 4.99 (1H, q, J=1.2 Hz), 5.14 and 5.18 (each 1H, each s), 6.20 (1H, d, J=1.8 Hz), 7.28 (1H, d, J= 1.8 Hz); 13C-NMR δ 9.6, 18.9, 69.4, 110.7, 113.1, 117.0, 141.1, 144.6, 148.7; MS (EI): 152 (M+); HRMS (EI) calcd for C9H12O2: 152.0837. Found: 152.0844.
α-(1-Methylethenyl)-3-furanethanol (3b) 16
Colorless oil; IR 3450, 2940, 1020 cm-1; 1H-NMR δ 1.79 (3H, s), 2.64 (1H, dd, J= 7.9 and 14.6 Hz), 2.73 (1H, dd, J= 4.9 and 14.6 Hz), 4.21 (1H, dd, J= 4.9 and 7.9 Hz), 4.88 (1H, brs), 4.98 (1H, d, J= 1.8 Hz), 6.33 (1H, brs), 7.32 (1H, brs), 7.38 (1H, d, J=1.8 Hz); 13C-NMR δ 18.2, 31.3, 75.3, 111.4, 111.5, 121.0, 140.3, 143.1, 146.7; MS (EI): 152 (M+); HRMS (EI) calcd for C9H12O2: 152.0837. Found: 152.0831.
3-Methyl-α-1-propenyl-2-furanmethanol (E/Z=82:18) (2c)
Colorless oil; IR 3380, 2920, 1020 cm-1; 1H-NMR (E isomer) δ 1.73 (3H, d, J= 6.2 Hz), 2.05 (3H, s), 5.17 (1H, d, J=6.5 Hz,), 5.74 (1H, dq, J=6.2 and 15.3 Hz), 5.83 (1H, dd, J= 6.5 and 15.3 Hz), 6.19 (1H, d, J=1.8 Hz), 7.27 (1H, d, J= 1.8 Hz); 13C-NMR (E isomer) δ 9.6, 17.8, 67.0, 113.1, 116.1, 127.8, 130.3, 141.0, 149.8; MS (EI): 152 (M+); MS (EI): 152 (M+); HRMS (EI) calcd for C9H12O2: 152.0837. Found: 152.0837.
α-1-Propenyl-3-furanethanol (E/Z=82:18) (3c)
Colorless oil; IR 3430, 2860, 960 cm-1; 1H-NMR (E isomer) δ 1.70 (3H, d, J= 6.1 Hz), 2.60 (1H, dd, J= 6.7 and 14.6 Hz), 2.66 (1H, dd, J= 6.1 and 14.6 Hz), 4.21 (1H, q, J= 6.7 Hz), 5.53 (1H, ddd, J= 1.2, 6.7, and 15.3 Hz), 5.70 (1H, dq, J= 6.1 and 15.3 Hz), 6.31 (1H, brs), 7.30 (1H, brs), 7.37 (1H, d, J=1.8 Hz); 13C-NMR (E isomer) δ 17.7, 33.1, 72.4, 111.5, 120.7, 127.3, 133.1, 140.2, 142.9; MS (EI): 152 (M+); HRMS (EI) calcd for C9H12O2: 152.0837. Found: 152.0842.
3-Methyl-α-(2-methyl-1-propenyl)-2-furanmethanol (2d)
Colorless oil; IR 3360, 2920, 1020 cm-1; 1H-NMR δ 1.73 and 1.75 (each 3H, each brs), 2.05 (3H, s), 5.43 (1H, d, J=8.7 Hz,), 5.64 (1H, d, J=8.7 Hz), 6.16 (1H, d, J=1.6 Hz), 7.27 (1H, d, J= 1.6 Hz); 13C-NMR δ 9.6, 18.6, 25.8, 62.8, 113.0, 115.6, 124.1, 135.7, 140.9, 150.4; MS (EI): 166 (M+); HRMS (EI) calcd for C10H14O2: 166.0993. Found: 166.0991.
α-(2-Methyl-1-propenyl)-3-furanethanol (3d)
Colorless oil; IR 3430, 2860, 1020 cm-1; 1H-NMR δ 1.66 and 1.73 (each 3H, each d, J= 1.2 Hz), 2.59 (1H, dd, J= 5.5 and 14.0 Hz), 2.65 (1H, dd, J= 6.7 and 14.0 Hz), 4.50 (1H, dd, J= 6.1 and 6.7 Hz), 5.22 (1H, dm, J= 8.6 Hz), 6.32 (1H, brs), 7.29 (1H, brs), 7.37 (1H, d, J=1.8 Hz); 13C-NMR δ 18.2, 25.7, 33.2, 68.4, 111.5, 120.8, 127.1, 135.8, 140.2, 142.8; MS (EI): 166 (M+); HRMS (EI) calcd for C10H14O2: 166.0993. Found: 166.0991.
α-Ethynyl-3-methyl-2-furanmethanol (2e)
Colorless oil; IR 3290, 2920, 990 cm-1; 1H-NMR δ 2.10 (3H, s), 2.62 (1H, s), 5.47 (1H, brs), 6.21 (1H, d, J= 1.6 Hz), 7.31 (1H, d, J= 1.8 Hz); 13C-NMR δ 9.7, 56.2, 74.0, 81.1, 113.5, 117.7, 141.7, 146.6; MS (EI): 136 (M+); HRMS (EI) calcd for C8H8O2: 136.0524. Found; 136.0515.
α-Ethynyl-3-furanethanol (3e)
Colorless oil; IR 3290, 2950, 2120 cm-1; 1H-NMR δ 2.48 (1H, d, J= 2.2 Hz), 2.82 and 2.88 (each 1H, each dd, J= 4.2 and 14.6 Hz), 4.51 (1H, t, J= 4.2 Hz), 6.40 (1H, brs), 7.38 (2H, brs); 13C-NMR δ 33.2, 61.9, 73.4, 84.2, 111.6, 119.2, 140.7, 142.9; MS (EI): 136 (M+); HRMS (EI) calcd for C8H8O2: 136.0524. Found; 136.0502.
3-Methyl-α-phenyl-2-furanmethanol (2f)
Colorless oil; IR 3400, 2920, 1450, 1020 cm-1; 1H-NMR δ 2.00 (3H, s), 5.84 (1H, brs), 6.18 (1H, d, J= 1.8 Hz), 7.24-7.42 (6H, m); 13C-NMR δ 9.7, 68.1, 113.2, 116.9, 126.2, 127.6, 128.3, 14.3, 141.4, 149.7; MS (EI): 188 (M+); HRMS (EI) calcd for C12H12O2: 188.0837. Found; 188.0828.
α-Phenyl-3-furanethanol (3f)
Colorless oil; IR 3400, 2920, 1450, 1020 cm-1; 1H-NMR δ 2.83 (2H, d, J= 6.6 Hz), 4.79 (1H, t, J= 6.6 Hz), 6.20 (1H, brs), 7.23 (1H, brs), 7.23-7.40 (6H, m); 13C-NMR δ 34.9, 74.0, 111.3, 120.7, 125.8, 127.6, 128.3, 140.3, 142.9, 143.7; MS (EI): 188 (M+); HRMS (EI) calcd for C12H12O2: 188.0837. Found; 188.0818.
Methyl 2-hydroxy-2-(3-methyl-2-furyl)acetate (2g)
Colorless oil; IR 3520, 2940, 1740, 1060 cm-1; 1H-NMR δ 2.08 (3H, s), 3.80 (3H, s), 5.21 (1H, d, J= 4.9 Hz), 6.22 (1H, d, J= 1.8 Hz), 7.28 (1H, d, J= 1.8 Hz); 13C-NMR δ 9.5, 53.1, 64.9, 113.2, 118.9, 141.9, 145.7, 172.3; MS (EI): 170 (M+); HRMS (EI) calcd for C8H10O4: 170.0579. Found; 170.0590.
α-Ethenyl-3-ethyl-2-furanmethanol (2h)
Colorless oil; IR 3430, 2930, 1030 cm-1; 1H-NMR δ 1.16 (3H, t, J= 7.2 Hz), 2.47 (2H, q, J= 7.2 Hz), 5.20-5.23 (1H, m), 5.25-5.40 (2H, m), 6.05-6.20 (1H, m), 6.26 (1H, d, J= 1.8 Hz), 7.28 (1H, d, J= 1.8 Hz); 13C-NMR δ 14.1, 17.8, 77.4, 111.9, 115.6, 116.7, 140.9, 141.3, 149.7; MS (EI): 152 (M+); HRMS (EI) calcd for C9H12O2: 152.0837. Found: 152.0810.
α-Ethenyl-2-methyl-3-furanethanol (3h)
Colorless oil; IR 3410, 2930, 1030 cm-1; 1H-NMR (threo) δ 1.21 (3H, d, J= 7.1 Hz), 2.78 (1H, quint, J= 7.1), 4.04 (1H, t, J= 7.1 Hz), 5.10-5.30 (2H, m), 5.75-5.92 (1H, m), 6.34 (1H, brs), 7.31 (1H, brs), 7.39 (1H, brs); 13C-NMR δ 16.9, 36.6, 76.9, 109.9, 116.5, 126.1, 138.8, 139.6, 143.0; MS (EI): 152 (M+); HRMS (EI) calcd for C9H12O2: 152.0837. Found: 152.0823.
Methyl 2-hydroxy-2-(3-methyl-2-furyl)propionate (2i)
Colorless oil; IR 3520, 2940, 1740, 1060 cm-1; 1H-NMR δ 1.81 (3H, s), 2.05 (3H, s), 3.79 (3H, s), 6.18 (1H, d, J= 1.8 Hz), 7.24 (1H, d, J= 1.8 Hz); 13C-NMR δ 10.2, 24.1, 53.2, 72.6, 114.4, 116.7, 140.4, 147.9, 174.9; MS (EI): 184 (M+); HRMS (EI) calcd for C9H12O4: 184.0735. Found; 184.0755.
Methyl 3-(3-furyl)-2-hydroxy-2-methylpropionate (3i)
Colorless oil; IR 3410, 2920, 1740, 1020 cm-1; 1H-NMR δ 1.47 (3H, s), 2.74 and 2.92 (each 1H, each d, J= 14.3 Hz), 3.75 (3H, s), 6.26 (1H, brs), 7.33 (2H, brs); 13C-NMR δ 25.7, 35.8, 52.7, 74.8, 111.8, 118.9, 140.7, 142.7, 176.7; MS (EI): 184 (M+); HRMS (EI) calcd for C9H12O4: 184.0735. Found; 184.0755.
(E)-α-(2.6-Dimethyl-1,5-heptadienyl)-3-furanethanol (8)
Treatment of 7 with sec-BuLi afforded the corresponding geraniol 8 in 82% yield. Colorless oil; IR 3450, 2900, 1010 cm-1; 1H-NMR δ 1.60 and 1.68 (each 3H, each brs), 1.64 (3H, d, J= 1.2 Hz), 1.95-2.15 (4H, m), 2.59 (1H, dd, J= 5.5 and 14.7 Hz), 2.66 (1H, dd, J= 6.7 and 14.7 Hz), 4.51 (1H, dq, J= 1.8 and 6.7 Hz), 5.00-5.15 (1H, m), 5.22 (1H, dq, J= 1.2 and 8.5 Hz), 6.32 (1H, brs), 7.28 (1H, brs), 7.36 (1H, t, J=1.8 Hz); 13C-NMR δ 16.6, 17.6, 25.6, 26.3, 33.1, 39.5, 68.4, 111.5, 120.8, 123.8, 126.8, 131.7, 139.0, 140.2, 142.8; MS (EI): 234 (M+); HRMS (EI) calcd for C15H22O2: 234.1618. Found: 234.1603.
(E)-α-(2.6-Dimethyl-1,5-heptadienyl)-3-furanethanol acetate (9)
A solution of alcohol 8 (27 mg, 0.115 mmol), Ac2O (150 mg, 1.47 mmol), and pyridine (200 mg, 2.53 mmol) in CH2Cl2 was stirred for 3 h at 0 °C. The reaction mixture was poured into water and the product was extracted with Et2O-hexane (v/v=1:1). The organic layer was washed successively with saturated aqueous KHSO4, brine, sat. aq. NaHCO3, and brine, and dried over Na2SO4. Evaporation of the solvent gave a residue, which was purified by silica gel column chromatography using Et2O-hexane (v/v=1:49) to give acetate 9 (29 mg) in 91% yield. Colorless oil; IR 2880, 1710, 1020 cm-1; 1H-NMR δ 1.59 and 1.68 (each 3H, each brs), 1.64 (3H, d, J= 1.2 Hz), 2.02 (3H, s), 1.95-2.15 (4H, m), 2.62 and 2.76 (each 1H, each dd, J= 6.7 and 14.7 Hz), 5.04 (1H, brt, J= 6.7 Hz), 5.13 (1H, dd, J= 1.2 and 9.1 Hz), 5.62 (1H, dt, J= 6.7 and 9.1 z), 6.28 (1H, d, J= 1.2 Hz), 7.23 (1H, brs), 7.33 (1H, t, J=1.8 Hz); MS (EI): 216 (M+-AcOH); HRMS (EI) calcd for C17H24O3 - AcOH: 216.1514. Found: 216.1514.
Dendrolasin (10)
To a stirred solution of acetate 9 (18 mg, 0.065 mmol) in EtNH2 (3 mL) was added portionwisely Li metal (9 mg, 1.3 mmol) at 2 °C and the reaction mixture was stirred for 4 h at the same temperature. After filtration of excess Li metal, filtrate was condensed to give a residue, which was purified by silica gel column chromatography using hexane to give dendrolasin 10 (5 mg) in 39% yield. The spectroscopic data obtained were identical with those reported.10c
Naginata ketone (11)
A mixture of alcohols 2d and 3d (231 mg, 2d/3d=21:79, 1.39 mmol), obtained by treatment of 1d with s-BuLi, and MnO2 (4.8 g, 55.2 mmol) in CH2Cl2 (50 mL) was stirred for 8 h at rt. After filtration of inorganic material, filtrate was condensed to give a residue, which was purified by silica gel column chromatography using Et2O-pentane (v/v=1:49) to give naginata ketone 11 (5.4 mg) in 11% yield based on 2d. The spectroscopic data obtained were identical with those reported.11b
ACKNOWLEDGEMENTS
This work was supported in part by a Grant-in-Aid for Scientific Research from the Ministry of Education, Culture, Sports, Science and Technology of Japan, and by the Open Research Center Project.
Dedicated to Dr. Keiichiro Fukumoto, Professor Emeritus of Tohoku University, on the occasion of his 75th birthday.
References
1. (a) C. Djerassi, in ‘Dictionary of Natural Porducts,’ Chapman & Hall, London, 1994; (b) M. Regitz, in ‘Science of Synthesis‘,Vol. 9, ed. by G. Maas, Thieme, Stuttgart, 2001.
2. (a) B. H. Lipshutz, Chem. Rev., 1986, 86, 795; CrossRef (b) T. Hurst, in ‘Rodd’s Chemistry of Carbon Compounds, 2nd Ed.’, 2nd suppl., Vol. IVA, chap. 2, ed. by M. Sainsbury, Elsevier, Amsterdam, 1977..
3. (a) C. B. Bird and G. W. H. Cheesman, in ‘Comprehensive Heterocyclic Chemistry’, Vol. 4, p. 89, ed. by A. R. Katritzky and C. W. Rees, Pergamon Press, Oxford, 1984., 1977, 3, 215; (b) R. Vieser and W. Eberbach, Tetrahedron., 1995, 36, 4405 and references cited therein.. CrossRef
4. (a) M. Tsubuki, H. Okita, and T. Honda, J. Chem. Soc., Chem. Commun., 1995, 2135; CrossRef (b) M. Tsubuki, T. Katama, H. Okita, M. Arai, A. Shigihara, and T. Honda, Chem. Commun, 1999, 2263. CrossRef
5. (a) M. Tsubuki, K. Takahashi, and T. Honda, J. Org. Chem., 2003, 68, 10183; CrossRef (b) M. Tsubuki, A. Ohinata, T. Tanaka, K. Takahashi, and T. Honda, Tetrahedron, 2005, 61, 1095; CrossRef (c) M. Tsubuki, S. Matsuo, and T. Honda, Tetrahedron Lett., 2008, 49, 229; CrossRef (d) M. Tsubuki, S. Matsuo, and T. Honda, Heterocycles, 2008, 76, 257. CrossRef
6. B. Cazes and S. Julia, Synth. Commun., 1977, 113. CrossRef
7. MO calculations were performed using the IBM RS/6000 version of the GAUSSIAN 92 suite of programs.
8. For recent reviews, see (a) J. A. Marshall, ‘Comprehensive Organic Synthesis;’ Vol. 3, ed. by B. M. Trost and I. Fleming, Pergamon Press: New York, 1991, pp. 975-1014; (b) T. Nakai and K. Mikami, Org. React. 1994, 46, 105; (c) K. Tomooka, ‘The Chemistry of Organolithium Compounds;’ ed. by Z. Rappoport and I. Marek, Wiley, New York, 2004, pp. 749-828. CrossRef
9. K. Mikami, Y. Kimura, N. Kishi, and T. Nakai, J. Org. Chem., 1983, 48, 279. CrossRef
10. For the isolation of dendrolasin, see (a) A. Quilico, F. Piozzi, and M. Pavan, Tetrahedron, 1957, 1, 177; CrossRef (b) T. Sakai, K. Nishimura, and Y. Hirose, Bull. Chem. Soc. Jpn., 1965, 38, 381; CrossRef For the synthesis of dendrolasin, see (c) A. Chakraborty, G. K. Kar, and J. K. Ray, Tetrahedron, 1997, 53, 8513 and references cited therein. CrossRef
11. For the isolation of naginata ketone, see (a) Y. Fujita and T. Ueda, Chem. & Ind., 1960, 236; For the synthesis of naginata ketone, see (b) G. Büchi, E. Kovats, P. Enggist, and G. Uhde, J. Org. Chem., 1968, 33, 1227; CrossRef (c) G. Cahiez, P. Y. Chavant, and E. Metais, Tetrahedron Lett., 1992, 33, 5245 and references cited therein. CrossRef
12. D. H. R. Barton and S. W. McCombie, J. Chem. Soc., Perkin Trans. 1, 1975, 157.
13. W. G. Dauben and D. J. Hart, J. Am. Chem. Soc., 1977, 99, 7307. CrossRef
14. R. Boese, D. F. Harvey, M. J. Malaska, and K. P. C. Vollhardt, J. Am. Chem. Soc., 1994, 116, 11153. CrossRef
15. R. Noyori, T. Sato, and H. Kobayashi, Bull. Chem. Soc. Jpn., 1983, 56, 2661. CrossRef
16. S. P. Tanis and P. M. Herrinton, J. Org. Chem., 1983, 48, 4572 CrossRef