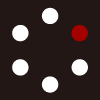
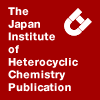
HETEROCYCLES
An International Journal for Reviews and Communications in Heterocyclic ChemistryWeb Edition ISSN: 1881-0942
Published online by The Japan Institute of Heterocyclic Chemistry
e-Journal
Full Text HTML
Received, 7th July, 2008, Accepted, 22nd August, 2008, Published online, 25th August, 2008.
DOI: 10.3987/COM-08-S(F)41
■ Formation of O-Glycosidic Linkages from 1-Hydroxy Sugars by Bismuth(III) Triflate-catalyzed Dehydrative Glycosidation
Takashi Yamanoi,* Ryo Inoue, Sho Matsuda, Kazuya Iwao, Yoshiki Oda, Akihiro Yoshida, and Keita Hamasaki
The Noguchi Institute, 1-8-1, Kaga, Itabashi-ku, Tokyo 173-0003, Japan
Abstract
This paper describes the direct formation of various O-glycosidic linkages from 1-hydroxy sugars by bismuth(III) triflate-catalyzed dehydrative glycosidation. The condensation reactions of 1-hydroxy sugars with some primary alcohols in the presence of only 5 mol% bismuth(III) triflate at reflux temperature for 15 min in dichloromethane afforded O-glycosides in good yields. An 1,6-anhydro-β-D-glucopyranosidic linkage was formed by the intramolecular condensation of the corresponding 1-hydroxy sugar performed with similar reaction conditions using 5 mol% bismuth(III) triflate. A reaction using 10 mol% bismuth(III) triflate at room temperature in dichloromethane promoted the self- or cross-condensations of 1-hydroxy sugars to produce several kinds of 1,1’-disaccharides. This paper reports some important properties of bismuth(III) triflate catalyzed dehydrative glycosidation using 1-hydroxy sugars to form various O-glycosidic linkages.INTRODUCTION
The development of an efficient preparation of complex glycosides is a major focus in synthetic carbohydrate chemistry.1 Dehydrative glycosidation of 1-hydroxy sugars, which are easy to prepare and handle, can be a simple and versatile method for glycosidic bond formations.2 Due to the generally poor leaving ability of the anomeric hydroxyl group of 1-hydroxy sugars, most of the conventional dehydrative O-glycosidation methods using 1-hydroxy sugars require the formation of a highly reactive glycosyl
donor in the reaction system by in situ introduction of a transient leaving group at its anomeric hydroxyl group.3
Dehydrative glycosidation by an acid catalyst, which can directly activate the hemiacetal hydroxyl group of 1-hydroxy sugars, is considered to be a more practical approach because it does not require a transient leaving group. However, there have been only a few reports on such dehydrative glycosidation approaches using an acid catalyst.4 This type of dehydrative glycosidation, called “Fischer glycosidation”, requires the use of large excesses of acid (activator) and alcohol (acceptor) in order to increase the reactivity.5 It is problematic that the reaction system using the stoichiometric amounts of an alcohol produces 1,1’-disaccharide (a non-reducing disaccharide) as a byproduct through self-condensation of 1-hydroxy sugars.6 Therefore, it is important to develop an efficient dehydrative O-glycosidation method that does not require excess amounts of acid and alcohol in order to prevent the formation of the 1,1′-disaccharides. On the other hand, there is currently a significant emphasis on the study of the biologically novel functions of 1,1’-disaccharide derivatives, which are structurally-classified as trehalose analogs.7 Therefore, the establishment of an efficient method for preparing 1,1’-disaccharides directly from 1-hydroxy sugars also becomes one of the most noteworthy subjects in dehydrative glycosidation studies.8
Our previous work showed that the O-ketopyranosidation9 via the dehydrative condensation of artificial ketopyranoses, i.e., 1-C-alkylated sugar derivatives, proceeded easily when using catalytic bismuth(III) triflate (Bi(OTf)3) as the activator.10 This finding prompted us to test the applicability of Bi(OTf)3 in dehydrative O-aldosylation using 1-hydroxy sugars.11 We then investigated the direct formation of various O-glycosidic linkages from 1-hydroxy sugars by dehydrative glycosidation using Bi(OTf)3. Part of the study, as reported in our preliminary letter, showed that the condensation of 1-hydroxy sugars with several primary alcohols in the presence of only 5 mol% Bi(OTf)3 at reflux temperature for 15 min produced O-aldopyranosides and also controlled the production of undesirable 1,1’-disaccharides.12 Our other studies of glycosidation using Bi(OTf)3 revealed the following significant findings. The O-D-arabinofuranosidic and 1,6-anhydro-β-D-glucopyranosidic linkages were formed from the corresponding 1-hydroxy sugars under the similar glycosidation systems using 5 mol% Bi(OTf)3. In addition, various 1,1’-disaccharides were produced in good yields by self- or cross-condensation with 1-hydroxy sugars in the presence of 10 mol% Bi(OTf)3 at room temperature.
This paper describes the direct formation of various O-glycosidic linkages from 1-hydroxy sugars by Bi(OTf)3-catalyzed dehydrative glycosidation.
RESULTS AND DISCUSSION
The 1-hydroxy sugars and alcohols used in this study are shown in Figures 1 and 2. The typical
O-glycosides and 1,1’-disaccharides produced are shown in Figures 3 and 4.
1. Dehydrative glycosidation for producing typical O-glycosides (Scheme 1, Table 1)
We first investigated the glycosidation of 2,3,4,6-tetra-O-benzyl-D-glucopyranose (1) with an equimolar amount of 2-phenylethyl alcohol (7). The amount of Bi(OTf)3 was varied in CH2Cl2 for 2 h at room temperature in the presence of a drying agent, anhydrous calcium sulfate (CaSO4). These reaction conditions were similar to those previously reported.13 The reaction using 5 mol% Bi(OTf)3 afforded the corresponding glycoside 13 in only 35% yield. The use of 10 mol% Bi(OTf)3 effectively promoted the glycosidation to produce 13 in a respectable yield of 85% with an α/β ratio of 71/29. It is noteworthy that under these reaction conditions the production of 1,1’-disaccharide 26 decreased only to a 7% yield. The use of 20 mol% Bi(OTf)3 slightly reduced the yield of 13 to 68% with an increase in the yield of 26 to 13%. When the reaction temperature was raised to reflux temperature, the glycosidation using only 5 mol% Bi(OTf)3 in CH2Cl2 for 15 min easily proceeded to produce 13 in a good yield of 87% (α/β = 69/31) with the formation of 26 in only 9% yield. A similar reaction using a mixed CH2Cl2/MeCN (v/v = 1/2) solvent at reflux temperature gave 13 in 72% yield (α/β = 34/66). The effect of other metal triflates was examined. Similar reaction conditions employing 5 mol% scandium(III) triflate (Sc(OTf)3) and ytterbium(III) triflate (Yb(OTf)3) afforded 13 in 82% yield (α/β = 65/35) and 14% yield (α/β = 44/56), respectively. Sc(OTf)3 was fairly effective in dehydrative glycosidation. Thus, a reaction using only 5 mol% Bi(OTf)3 at reflux temperature in CH2Cl2 for 15 min effectively promoted the glycosidation between 1 and 7 (1 equivalent) to produce the desired glycoside 13 with only a slight production of 26.
Next, we examined the glycosidation of 1 with various alcohols (1 equivalent) using 5 mol% Bi(OTf)3 at reflux temperature in CH2Cl2 for 15 min. A reaction using n-octanol (8) produced the glycoside 18 in a good yield of 85% (α/β = 62/38) with the formation of 26 in only 9% yield. The glycosylation of 1 to methyl 2,3,4-tri-O-benzyl-α-D-glucopyranoside (9) afforded 20 in a moderate yield of 52% (α/β = 75/25) with the production of 26 in 11% yield. Even reactions using less reactive acceptors such as phenol (10) and 1-adamantanol (11) produced the corresponding glycosides 21 and 22 in 40% (α/β = 80/20) and 58% (α/β = 65/35) yields with the formation of 26 in 21% and 17% yields, respectively. In addition, during the reaction using 10, the benzyl glucoside 23 was obtained as a new byproduct in 31% yield. It seemed that the benzyl alcohol was formed along with the degradation of 1 under the given reaction conditions using 10. The glycosidation of 1 with some primary alcohols afforded the desired glycosides in good yields. In contrast, for the reactions using less reactive alcohols, the yields of the desired glycosides decreased due to increase in the yield of 26 and the appearance of a new byproduct 23.
Similarly, 2,3,4,6-tetra-O-benzyl-D-mannopyranose (2), 2,3,4,6-tetra-O-benzyl-D-galactopyranose (3), 2-azido-3,4,6-tri-O-benzyl-2-deoxy-D-glucopyranose (4) and 2,3,5-tri-O-benzyl-D-arabinofuranose (6) were used as 1-hydroxy sugars. The reaction of 2 with 7 or 8 under the reaction conditions using 5 mol% Bi(OTf)3 at reflux temperature for 15 min stereoselectively afforded the corresponding glycosides 14 or 19 in 73% yield (α only) or 81% yield (α/β = 91/9) and the production of the 1,1’-disaccharide 27 in 16% yield in both cases. Similar reaction conditions using 3, 4, or 6 with 7 gave the glycosides 15, 16 or 17 in 70% yield (α/β = 77/23), 51% yield (α/β = 52/48) or 81% yield (α/β = 85/15) with the production of the 1,1’-disaccharide 28, 29 or 30 in 10%, 15% or 5% yield, respectively. The reaction of 1 with 8 using 5 mol% Bi(N(SO2-n-C8F17)2)314 instead of Bi(OTf)3 afforded 18 in 71% yield (α/β = 60/40) with the production of 26 in 16% yield.
The formation of an 1,6-anhydro-β-glucopyranosidic linkage from 2,3,4-tri-O-benzyl-D-glucopyranose (5) was examined. The reaction conditions using 5 mol% Bi(OTf)3 at reflux temperature for 15 min in CH2Cl2 easily promoted intramolecular condensation of 5 to give 1,6-anhydro-2,3,4-tri-O-benzyl-β-D-glucopyranose (24) in 73% yield. A reaction using 5 and allyl alcohol (10 equivalent) in the presence of 20 mol% Bi(OTf)3 produced the allyl O-glycoside 25 in 78% yield (α/β = 65/35) as a main product and 24 was given in only 16% yield. This result indicated that under theses reaction conditions the intermolecular condensation of 5 with allyl alcohol preceded the intramolecular condensation of 5.
2. Dehydrative glycosidation for producing 1,1’-disaccharides (Scheme 2, Table 2)
We next, investigated the formation of the symmetrical 1,1’-disaccharide 26 by the self-condensation of 1 in detail. The reactions using 5 mol% and 10 mol% Bi(OTf)3 at reflux temperature for 15 min promoted the self-condensation of 1 to produce 26 in 77% and 71% yields. At the same time, however, the benzyl glucoside 23 was obtained as a byproduct in 19% and 14% yields, respectively. The reaction using 5 mol% Bi(OTf)3 at room temperature for 15 h afforded 26 in only 12% yield with no production of 23. The use of 10 mol% Bi(OTf)3 at room temperature increased the yield of 26 to 85% with an αα/αβ/ββ isomer ratio of 53/33/14. The use of 50 mol% Bi(OTf)3 decreased the yield of 26 to 59% and increased the yield of 23 to 35%. The increase of Bi(OTf)3 seemed to promote the degradation of 1.
The effect of other metal triflates was also examined using Sc(OTf)3 and Yb(OTf)3. Sc(OTf)3 was a fairly effective activator for the production of 26 in 67% yield, while Yb(OTf)3 was completely ineffective. This result roughly corresponded to that of entries 6 and 7 in Table 1.
Subsequently, the synthesis of various symmetrical 1,1’-disaccharides 27, 28, 29 and 30 by the self-condensation of the 1-hydroxy sugars 2-4 and 6 was examined. Similar reaction conditions using 10 mol% Bi(OTf)3 in CH2Cl2 at room temperature for 3 to 15 h successfully gave 27, 28 and 30 in good yields of 85% (αα only), 70% (αα/αβ/ββ= 71/29/0) and 93% (αα/αβ/ββ= 85/15/0) from 2, 3 and 6. However, the same reaction conditions were not applicable to self-condensation using 4 at all. The reaction conditions using 100 mol% Bi(OTf)3 increased the yield of 29 up to 53% (αα/αβ/ββ= 35/32/33).
We observed that the reactivity of 6 was higher than that of 1. Next, the synthesis of the unsymmetrical structural 1,1’-disaccharide 31 by the cross-condensation using 6 and 1 was examined. The reaction of 6 with 1 (1.5 equivalent) using 10 mol% Bi(OTf)3 for 3.5 h afforded the desired 31 in a good yield of 74% with a mixture of αα/αβ/βα/ββ isomers = 31/31/31/7. Under these reaction conditions 30 was obtained in 11% yield and 26 was scarcely produced.
CONCLUSIONS
The direct formation of various O-glycosidic linkages from 1-hydroxy sugars by Bi(OTf)3-catalyzed dehydrative glycosidation was demonstrated. The reactions of several benzylated 1-hydroxy sugars with certain primary alcohols using only 5 mol% Bi(OTf)3 at reflux temperature in dichloromethane for 15 min successfully afforded the desired O-glycosides in good yields. These reaction conditions controlled the formation of unwanted 1,1’-disaccharides. The application of a similar reaction system effectively led to the formation of an 1,6-anhydro-β-D-glucopyranosidic linkage from the corresponding 1-hydroxy sugar. Self- or cross-condensation with 1-hydroxy sugars was promoted by 10 mol% Bi(OTf)3 in CH2Cl2 at room temperature and produced various symmetrical or unsymmetrical 1,1’-disaccharides. Therefore, we believe that our dehydrative glycosidation procedure can contribute to the convenient production of certain chemically or biologically useful glycosides.
EXPERIMENTAL
1H NMR (600 MHz) and 13C NMR (150 MHz) spectra were recorded on a JEOL ECA-600 spectrometer in CDCl3 using TMS as an internal standard. Optical rotations were recorded on a JASCO DIP-360 digital polarimeter. HRMS were obtained on a Mariner spectrometer (PerSeptive Biosystems Inc.). Preparative TLC was performed using Merck silica gel 60GF254. Column chromatography was conducted using silica gel 60 N (40~50 µm, Kanto Chemical Co., INC.). Bi(OTf)3 was purchased from Sigma-Aldrich. All anhydrous solvents were purified according to standard methods.
2-Phenylethyl 2,3,4,6-Tetra-O-benzyl-D-glucopyranoside (13) (Table 1, Entry 4): To a stirred suspension of Bi(OTf)3 (5.4 mg, 0.0082 mmol) and 7 (20.3 mg, 0.17 mmol) in CH2Cl2 (3 mL) was added 1 (89.2 mg, 0.17 mmol) in the presence of anhydrous CaSO4 (ca. 100 mg) under an Ar atmosphere. The resulting mixture was stirred at reflux temperature for 15 min. The reaction was then quenched by the addition of a saturated aqueous NaHCO3 solution (5 mL). The reaction mixture was extracted with EtOAc, and the organic layer was washed with water and a saturated aqueous NaCl solution. After the organic layer was dried over Na2SO4, the solvent was evaporated under reduced pressure. The crude product was purified using a preparative silica gel TLC (PTLC; EtOAc/hexane = 1/3) to give the desired glycoside 13 (92.4 mg, 87%, a mixture of α and β anomers) as a colorless oil and 1,1’-disaccharide 26 (8.3 mg, 9%) as a colorless oil. 1H NMR δ 2.92-2.99 (m, CH2CH2Phαβ), 3.99 (dd, J = 8.2 Hz, J = 8.9 Hz, H-3α), 4.19-4.23 (m, CHaHbCH2Phβ), 4.41 (d, J = 7.6 Hz, H-1β), 4.77 (d, J = 3.4 Hz, H-1α); 13C NMR δ 35.9, 36.2, 68.4, 68.7, 68.9, 70.1, 70.6, 73.1, 73.36, 73.41,74.6, 74.80, 74.82, 74.9, 75.59, 75.60, 77.6, 77.8, 80.0, 81.9, 82.2, 84.6, 96.8 (C-1α), 103.6 (C-1β); HRMS (ESI, α/β mixture) m/z calcd for 667.3030 (C42H44O6+Na+), found 667.2993.
Octyl 2,3,4,6-Tetra-O-benzyl-D-glucopyranoside (18) (Table 1, Entry 8): The same procedure used for the preparation of 13 using Bi(OTf)3 (5.1 mg, 0.0078 mmol), 8 (20.2 mg, 0.16 mmol), and 1 (84.1 mg, 0.16 mmol) in CH2Cl2 (3 mL) gave the desired glycoside 18 (PTLC; EtOAc/hexane = 1/3, 85.6 mg, 85%, a mixture of α and β anomers) as a colorless oil and 26 (7.1 mg, 9%). (Table 1, Entry 9): The same procedure using Bi(N(SO2-n-C8F17)2)3 (32.2 mg, 0.01 mmol), 8 (27.0 mg, 0.2 mmol), and 1 (111.9 mg, 0.21 mmol) in CH2Cl2 (3 mL) gave the desired glycoside 18 (92.1 mg, 71%, a mixture of α and β anomers) and 26 (11.5 mg, 10%). 1H NMR δ 0.86-0.89 (m, CH3), 1.27-1.70 (m, (CH2)6), 3.40-3.47 (m, H-2β, H-5β, CHaHb(CH2)6CH3α), 3.51-3.69 (m, H-2α, H-4α, Ha-6α, CHaHb(CH2)6CH3α, H-3β, H-4β, Ha-6β, CHaHb(CH2)6CH3β), 3.72 (dd, J = 3.4 Hz, J = 10.3 Hz, Hb-6α), 3.73-3.75 (m, Hb-6β), 3.77-3.80 (m, H-5α), 3.95-3.99 (m, CHaHb(CH2)6CH3β), 3.99 (dd, J = 8.9 Hz, J = 9.6 Hz, H-3α), 4.39 (d, J = 7.6 Hz, H-1β), 4.76 (d, J = 4.1 Hz, H-1α); 13C NMR δ 14.1, 22.6, 26.11, 26.14, 29.18, 29.21, 29.3, 29.3, 29.4, 29.7, 31.8, 31.8, 68.2, 68.5, 69.0, 70.0, 70.1, 73.1, 73.4, 73.4, 74.7, 74.8, 74.9, 75.0, 75.6, 75.6, 77.7, 77.9, 80.1, 82.1, 82.2, 84.7, 96.8 (C-1α), 103.6 (C-1β); HRMS (ESI, α/β mixture) m/z calcd for 675.3656 (C42H52O6+Na+), found 675.3680. (Lit.,15 α anomer: 1H NMR δ 4.76 (J = 2.1 Hz, H-1); 13C NMR δ 96.8 (C-1); β anomer: 1H NMR δ 4.39 (J = 7.6 Hz, H-1); 13C NMR δ 103.6 (C-1)).
Methyl 6-O-(2,3,4,6-Tetra-O-benzyl-D-glucopyranosyl)-2,3,4-tri-O-benzyl-α-D-glucopyranoside (20) (Table 1, Entry 10): The same procedure used for the preparation of 13 using Bi(OTf)3 (5.0 mg, 0.0076 mmol), 9 (70.8 mg, 0.15 mmol), and 1 (82.4 mg, 0.15 mmol) in CH2Cl2 (3 mL) gave the desired glycoside 20 (PTLC; EtOAc/hexane = 1/3, 77.7 mg, 52%, a mixture of α and β anomers) as a colorless oil and 26 (9.2 mg, 11%). 1H NMR δ 3.25 (s, CH3β), 3.35 (s, CH3 α), 3.43 (dd, J = 3.4 Hz, J = 8.9 Hz, H-2’α), 3.48-3.57 (m, H-2α, H-2’β), 3.94-4.01 (m, H-3’α, H-3α), 4.35 (d, J = 8.2 Hz, H-1’β), 4.50-4.62 (m, H-1α, H-1β), 4.98 (d, J = 4.1 Hz, H-1’α); 13C NMR δ 97.2 (C-1’αα), 97.9 (C-1αα), 98.0 (C-1βα), 103.7 (C-1’βα); HRMS (ESI, α/β mixture) m/z calcd for 1009.4497 (C62H66O11+Na+), found 1009.4479. (Lit.,16 α anomer: 1H NMR δ 4.98 (J = 3.7 Hz, H-1’); 13C NMR δ 97.9 (C-1’); β anomer: 1H NMR δ 4.34 (J = 7.8 Hz, H-1’); 13C NMR δ 103.7 (C-1’)).
Phenyl 2,3,4,6-Tetra-O-benzyl-D-glucopyranoside (21) (Table 1, Entry 11): The same procedure used for the preparation of 13 using Bi(OTf)3 (5.4 mg, 0.0082 mmol), 10 (15.6 mg, 0.16 mmol), and 1 (89.0 mg, 0.16 mmol) in CH2Cl2 (3 mL) gave 21 (PTLC; toluene/EtOAc = 10/1, 40.3 mg, 40%, a mixture of α and β anomers) as a colorless oil, 26 (18.5 mg, 21%) as a colorless oil and 2317 (32.2 mg, 31%) as a colorless oil. 1H NMR δ 3.56 (dd, J = 2.1 Hz, J = 10.3 Hz, Ha-6α), 3.60-3.80 (m, H-2α, Hb-6α, H-2β, H-3β, H-4β, H-5β, Ha-6β, Hb-6β), 3.79 (dd, J = 8.9 Hz, J = 10.3 Hz, H-4α), 3.87-3.89 (m, H-5α), 4.21 (dd, J = 8.9 Hz, J = 9.4 Hz, H-3α), 5.01 (d, J = 7.6 Hz, H-1β), 5.48 (d, J = 3.4 Hz, H-1α); 13C NMR δ 68.2, 68.8, 70.8, 73.3, 73.4, 73.5, 75.02, 75.05, 75.11, 75.77, 75.78, 77.4, 77.7, 79.7, 82.0, 84.7, 95.4 (C-1α), 101.6 (C-1β); HRMS (ESI, α/β mixture) m/z calcd for 639.2717 (C40H40O6+Na+), found 639.2693. (Lit.,16 α anomer: 1H NMR δ 5.48 (J = 3.4 Hz, H-1); 13C NMR δ 95.4 (C-1); β anomer: 1H NMR δ 5.01 (J = 7.6 Hz, H-1); 13C NMR δ 101.6 (C-1)).
1-Adamantyl 2,3,4,6-Tetra-O-benzyl-D-glucopyranoside (22) (Table 1, Entry 12): The same procedure used for the preparation of 13 using Bi(OTf)3 (5.6 mg, 0.0085 mmol), 11 (27.1 mg, 0.18 mmol), and 1 (89.9 mg, 0.17 mmol) in CH2Cl2 (3 mL) gave the desired glycoside 22 (PTLC; EtOAc/hexane = 1/3, 65.2 mg, 58%, a mixture of α and β anomers) as a colorless oil and 26 (15.0 mg, 17%). 1H NMR δ 1.56-2.17 (30H, m, 1-adamantyl), 3.42-3.50 (m, H-2β, H-4β, H-5β), 3.53 (dd, J = 4.1 Hz, J = 9.6 Hz, H-2α), 3.60-3.67 (m, Ha-6α, H-4α, Ha-6β, H-3β), 3.73 (dd, J = 2.1 Hz, J = 11.0 Hz, Hb-6β), 3.76 (dd, J = 3.4 Hz, J = 10.3 Hz, Hb-6α), 4.00-4.03 (m, H-5α), 4.01 (dd, J = 8.9 Hz, J = 9.6 Hz, H-3α), 4.70 (d, J = 7.6 Hz, H-1β), 5.28 (d, J = 3.4 Hz, H-1α); 13C NMR δ 30.6, 30.7, 36.3, 36.3, 42.4, 42.8, 68.8, 69.5, 69.6, 72.8, 73.3, 73.4, 74.51, 74.54, 74.9, 75.1, 75.3, 75.5, 75.7, 78.1, 78.2, 80.1, 82.1, 82.3, 85.1, 89.8 (C-1α), 96.2 (C-1β); HRMS (ESI, α/β mixture) m/z calcd for 697.3500 (C44H50O6+Na+), found 697.3538. (Lit.,18 β anomer: 1H NMR δ 4.69 (J = 7.6 Hz, H-1); 13C NMR δ 96.2 (C-1)).
2-Phenylethyl 2,3,4,6-Tetra-O-benzyl-α-D-mannopyranoside (14) (Table 1, Entry 13): The same procedure used for the preparation of 13 using Bi(OTf)3 (5.7 mg, 0.0087 mmol), 7 (21.3 mg, 0.17 mmol), and 2 (94.1 mg, 0.17 mmol) in CH2Cl2 (3 mL) gave the desired glycoside 14 (PTLC; EtOAc/hexane = 1/3, 82.1 mg, 73%) as a colorless oil and 27 (14.7 mg, 16%) as a colorless oil. [α]D22 +30.5° (c 4.1, CHCl3); 1H NMR δ 2.82 (2H, t, J = 6.9 Hz, CH2CH2Ph), 3.57-3.63 (2H, m, CHaHbCH2Ph, H-5), 3.67 (1H, dd, J = 2.1 Hz, J = 11.0 Hz, Ha-6), 3.71-3.74 (2H, m, H-2, Hb-6), 3.84-3.88 (2H, m, CHaHbCH2Ph, H-3), 3.95 (1H, t, J = 9.6 Hz, H-4), 4.84 (1H, d, J = 2.1 Hz, H-1); 13C NMR δ 36.0, 68.1, 69.3, 71.8, 72.1, 72.5, 73.3, 74.7, 74.9, 74.9, 80.0, 97.7 (C-1, JC1-H1 = 168.3 Hz); HRMS (ESI) m/z calcd for 667.3030 (C42H44O6+Na+), found 667.3073.
Octyl 2,3,4,6-Tetra-O-benzyl-D-mannopyranoside (19) (Table 1, Entry 14): The same procedure used for the preparation of 13 using Bi(OTf)3 (5.3 mg, 0.0081 mmol), 8 (21.1 mg, 0.16 mmol), and 2 (87.3 mg, 0.16 mmol) in CH2Cl2 (3 mL) gave the desired glycoside 19 (PTLC; EtOAc/hexane = 1/3, 84.9 mg, 81%, a mixture of α and β anomers) as a colorless oil and 27 (13.5 mg, 16%). 1H NMR δ 0.88 (t, J = 6.9 Hz, CH3α), 1.26-1.52 (m, CH2(CH2)6CH3), 3.35 (ddd, J = 2.8 Hz, J = 6.9 Hz, J = 9.6 Hz, CHaHb(CH2)6CH3α), 3.65 (ddd, J = 2.8 Hz, J = 6.9 Hz, J = 9.6 Hz, CHaHb(CH2)6CH3α), 3.72-3.80 (m, H-2α, H-5α, Ha-6α, Hb-6α), 3.91 (dd, J = 3.4 Hz, J = 9.6 Hz, H-3α), 3.99 (t, J = 9.6 Hz, H-4α), 4.37 (d, J = 0.7 Hz, H-1β), 4.86 (d, J = 1.4 Hz, H-1α); 13C NMR δ 14.1, 14.5, 22.61, 22.63, 26.08, 26.12, 29.16, 29.24, 29.3, 29.4, 29.7, 31.8, 67.6, 69.3, 69.7, 70.0, 71.3, 71.7, 72.1, 72.5, 73.3, 73.4, 73.5, 73.6, 74.8, 74.9, 75.0, 75.1, 75.9, 80.3, 82.3, 97.8 (C-1α, JC1-H1 = 165.7 Hz), 101.7 (C-1β, JC1-H1 = 152.6 Hz); HRMS (ESI, α/β mixture) m/z calcd for 675.3656 (C42H52O6+Na+), found 675.3663. (Lit.,19 α anomer: 1H NMR δ 4.86 (J = 2.1 Hz, H-1); 13C NMR δ 97.8 (C-1)).
2-Phenylethyl 2,3,4,6-Tetra-O-benzyl-D-galacutopyranoside (15) (Table 1, Entry 15): The same procedure used for the preparation of 13 using Bi(OTf)3 (4.6 mg, 0.007 mmol), 7 (17.1 mg, 0.14 mmol), and 3 (75.9 mg, 0.14 mmol) in CH2Cl2 (3 mL) gave the desired glycoside 15 (PTLC; EtOAc/hexane = 1/3, 63.6 mg, 70%, a mixture of α and β anomers) as a colorless oil and 28 (7.6 mg, 10%) as a colorless oil. 1H NMR δ 2.91-2.97 (m, CH2CH2Ph), 3.92 (dd, J = 2.8 Hz, J = 9.6 Hz, H-3α), 4.03 (dd, J = 3.4 Hz, J = 9.6 Hz, H-2α), 4.15-4.19 (m, CHaHbCH2Phβ), 4.37 (d, J = 7.6 Hz, H-1β), 4.83 (d, J = 3.4 Hz, H-1α); 13C NMR δ 36.0, 36.2, 68.6, 68.8, 68.9, 69.2, 70.5, 73.0, 73.1, 73.2, 73.30, 73.36, 73.39, 73.5, 74.4, 74.7, 75.0, 75.1, 76.5, 78.8, 79.5, 82.1, 97.4 (C-1α), 103.9 (C-1β); HRMS (ESI, α/β mixture) m/z calcd for 667.3030 (C42H44O6+Na+), found 667.3011.
2-Phenylethyl 2-Azido-3,4,6-tri-O-benzyl-2-deoxy-D-glucopyranoside (16) (Table 1, Entry 16): The same procedure used for the preparation of 13 using Bi(OTf)3 (6.8 mg, 0.01 mmol), 7 (24 µL, 0.2 mmol), and 4 (95.2 mg, 0.2 mmol) in CH2Cl2 (3 mL) gave the desired glycoside 16 (PTLC; EtOAc/hexane = 1/3, 59.5 mg, 51%, a mixture of α and β anomers) as a colorless oil and 29 (31.2 mg, 33%) as a colorless oil. 1H NMR δ 2.92-2.99 (4H, m, CH2CH2Ph), 3.34 (dd, J = 3.4 Hz, J = 10.3 Hz, H-2α), 3.84-3.88 (m, CHaHbCH2Phα), 3.95 (t, J = 9.6 Hz, H-3α), 4.12-4.16 (m, CHaHbCH2Phβ), 4.27 (d, J = 7.6 Hz, H-1β), 4.93 (d, J = 3.4 Hz, H-1α); 13C NMR δ 36.0, 36.2, 63.3, 66.4, 68.2, 68.6, 68.9, 70.6, 70.9, 73.4, 73.5, 74.8, 75.0, 75.3, 75.5, 77.7, 78.2, 80.1, 83.1, 97.6 (C-1α), 102.1 (C-1β); HRMS (ESI, α/β mixture) m/z calcd for 602.2625 (C35H37O5N3+Na+), found 602.2668.
2-Phenylethyl 2,3,5-Tri-O-benzyl-D-arabinofuranoside (17) (Table 1, Entry 17): The same procedure used for the preparation of 13 using Bi(OTf)3 (7.9 mg, 0.012 mmol), 7 (29.3 mg, 0.24 mmol), and 6 (101.2 mg, 0.24 mmol) in CH2Cl2 (3 mL) gave the desired glycoside 17 (PTLC; EtOAc/hexane = 1/3, 102.6 mg, 81%, a mixture of α and β anomers) as a colorless oil and 30 (4.5 mg, 5%) as a colorless oil. 1H NMR δ 2.86 (t, J = 6.9 Hz, CH2CH2Phβ), 2.90 (t, J = 6.9 Hz, CH2CH2Phα), 3.42 (d, J = 6.2 Hz, Ha-5β, Hb-5β), 3.57 (dd, J = 5.5 Hz, J = 11.0 Hz, Ha-5α), 3.57 (ddd, J = 2.8 Hz, J = 6.9 Hz, J = 10.3 Hz, CHaHbCH2Phβ), 3.61-3.66 (m, CHaHbCH2Phα, Hb-5α), 3.87 (ddd, J = 2.8 Hz, J = 6.9 Hz, J = 9.6 Hz, CHaHbCH2Phβ), 3.91 (dd, J = 2.8 Hz, J = 6.9 Hz, H-3α), 3.97 (ddd, J = 2.8 Hz, J = 6.9 Hz, J = 9.6 Hz, CHaHbCH2Phα), 4.00 (d, J = 2.1 Hz, H-2α), 4.03 (dd, J = 4.1, 6.9 Hz, H-2β), 4.07-4.11 (m, H-3β, H-4β), 4.13-4.16 (m, H-4α), 4.86 (d, J = 4.1 Hz, H-1β), 5.02 (s, H-1α); 13C NMR δ 36.0, 36.1, 68.3, 68.4, 69.6, 71.8, 72.0, 72.25, 72.28, 72.5, 73.2, 73.3, 80.2, 80.4, 83.3, 83.4, 84.2, 88.3, 100.4 (C-1β), 106.2 (C-1α); HRMS (ESI, α/β mixture) m/z calcd for 547.2455 (C34H36O5+Na+), found 547.2446. A part of α anomer was isolated. α Anomer: [α] D25 +46.6° (c 4.1, CHCl3).
1,6-Anhydro-2,3,4-tri-O-benzyl-β-D-glucopyranose (24) (Table 1, Entry 18): The same procedure used for the preparation of 13 using Bi(OTf)3 (6.8 mg, 0.01 mmol) and 5 (93.4 mg, 0.21 mmol) in CH2Cl2 (3 mL) gave 24 (PTLC; EtOAc/hexane = 1/2, 65.5 mg, 73%) as white crystals. [α] D22 -30.6° (c 1, CHCl3); 1H NMR δ 3.34 (1H, s, H-2 or H-3), 3.35 (1H, s, H-2 or H-3), 3.59 (1H, s, H-4), 3.67 (1H, dd, J = 6.2 Hz, J = 6.9 Hz, Ha-6), 3.90 (1H, d, J = 6.9 Hz, Hb-6), 4.54-4.60 (1H, m, H-5), 5.46 (1H, s, H-1); 13C NMR δ 65.3, 71.1, 71.7, 71.9, 74.3, 76.0, 76.1, 76.8, 100.6 (C-1); HRMS (ESI) m/z calcd for 455.1829 (C27H28O5+Na+), found 455.1858. (Lit.,20 [α] D20 -29.5° (c 1, CHCl3); Lit.,21 1H NMR δ 5.45 (bs, H-1)).
Allyl 2,3,4-Tri-O-benzyl-D-glucopyranoside (25) (Table 1, Entry 19): The same procedure used for the preparation of 13 using Bi(OTf)3 (22.5 mg, 0.034 mmol), 12 (117.1 µL, 1.7 mmol), and 5 (77.4 mg, 0.17 mmol) in CH2Cl2 (3 mL) gave 25α (PTLC; EtOAc/hexane = 1/2, 43.1 mg, 51%) as a colorless oil, 25β (22.9 mg, 27%) as a colorless oil, and 24 (12.2 mg, 16%) as a colorless oil. Compound 25α: [α] D25 +46.6° (c 4.1, CHCl3); 1H NMR δ 3.51 (1H, dd, J = 3.4 Hz, J = 9.6 Hz, H-2), 3.54 (1H, dd, J = 8.9 Hz, J = 9.6 Hz, H-4), 3.67-3.72 (2H, m, H-5, Ha-6), 3.74-3.77 (1H, m, Hb-6), 3.97-4.00 (1H, m, CHaHbCH=CH2), 4.04 (1H, dd, J = 8.9 Hz, J = 9.6 Hz, H-3), 4.14 (1H, dd, J = 5.5 Hz, J = 13.6 Hz, CHaHbCH=CH2), 4.77 (1H, d, J = 3.4 Hz, H-1), 5.22 (1H, dd, J = 1.4 Hz, J = 10.3 Hz, CH2CH=CHaHb), 5.31 (1H, dd, J = 1.4 Hz, J = 17.2 Hz, CH2CH=CHaHb), 5.92 (1H, m, CH2CH=CH2); 13C NMR δ 61.8, 68.2, 70.8, 73.2, 75.0, 75.7, 77.4, 80.0, 81.9, 95.6 (C-1), 118.2, 133.6; HRMS (ESI) m/z calcd for 513.2248 (C30H34O6+Na+), found 513.2293. Compound 25β: [α] D22 +4.5° (c 1.2, CHCl3); 1H NMR δ 3.35-3.37 (1H, m, H-5), 3.45 (1H, dd, J = 8.3 Hz, J = 8.9 Hz, H-2), 3.57 (1H, t, J = 9.6 Hz, H-4), 3.67 (1H, dd, J = 8.9 Hz, J = 9.6 Hz, H-3), 3.70-3.73 (1H, m, Ha-6), 3.85-3.88 (1H, m, Hb-6), 4.16 (1H, dd, J = 6.2 Hz, J = 13.1 Hz, CHaHbCH=CH2), 4.40 (1H, dd, J = 5.5 Hz, J = 13.1 Hz, CHaHbCH=CH2), 4.50 (1H, d, J = 7.6 Hz, H-1), 5.22 (1H, dd, J = 1.4 Hz, J = 10.3 Hz, CH2CH=CHaHb), 5.35 (1H, dd, J = 2.1 Hz, J = 17.1 Hz, CH2CH=CHaHb), 5.96 (1H, m, CH2CH=CH2); 13C NMR δ 62.0, 70.7, 74.97, 75.01, 75.1, 75.7, 77.5, 82.3, 84.5, 102.8 (C-1), 117.5, 133.87; HRMS (ESI) m/z calcd for 513.2248 (C30H34O6+Na+), found 513.2253.
2,3,4,6-Tetra-O-benzyl-D-glucopyranosyl 2,3,4,6-Tetra-O-benzyl-D-glucopyranoside (26) (Table 2, Entry 4): To a stirred solution of Bi(OTf)3 (8.3 mg, 0.013 mmol) in CH2Cl2 (3 mL) was added 1 (68.4 mg, 0.13 mmol) in the presence of anhydrous CaSO4 (ca. 100 mg) under an Ar atmosphere. The resulting mixture was stirred at rt for 1 d. The same procedure used for the preparation of 13 was followed. The crude product was purified using a preparative silica gel TLC (EtOAc/hexane = 1/3) to provide the desired 1,1’-disaccharide 26 (57.0 mg, 85%, a mixture of αα, αβ, and ββ isomers) as a colorless oil. 1H NMR δ 3.36-3.38 (m, Ha-6αα), 3.48-3.54 (m, Hb-6αα, H-2αβ-β, H-5ββ), 3.53 (dd, J = 7.6 Hz, J = 8.2 Hz, H-2ββ), 3.57-3.65 (2H, m, H-2αα, H-2αβ-α), 3.68 (1H, t, J = 9.6 Hz, H-4αα), 3.76 (dd, J = 7.6 Hz, J = 10.3 Hz, H-4αβ-α), 4.02 (1H, dd, J = 8.9 Hz, J = 9.6 Hz, H-3αα-α), 4.09 (1H, t, J = 9.6 Hz, H-3αβ-α), 4.15-4.17 (1H, m, H-5αα-α), 4.58 (1H, d, J = 7.6 Hz, H-1αβ-β), 4.90 (d, J = 8.2 Hz, H-1ββ), 5.16 (1H, d, J = 3.4 Hz, H-1αβ-α), 5.23 (1H, d, J = 3.4 Hz, H-1αα-α); 13C NMR δ 68.1, 68.9, 70.6, 73.5, 74.6, 74.97, 75.03, 75.6, 77.6, 77.7, 79.3, 81.7, 81.9, 82.2, 84.6, 94.4 (C-1αα), 99.3 (C-1ββ), 99.4 (C-1αβ-α), 104.1 (C-1αβ-β); HRMS (ESI, isomer mixture) m/z calcd for 1085.4810 (C68H70O11+Na+), found 1085.4798. A part of ββ isomer was isolated. ββ Isomer: [α] D20 +15.9° (c 0.42, CHCl3). (Lit.,22 αα isomer: 13C NMR δ 94.26 (C-1); αβ isomer: 13C NMR δ 99.54 (C-1α), 104.27 (C-1β)).
2,3,4,6-Tetra-O-benzyl-α-D-mannopyranosyl 2,3,4,6-Tetra-O-benzyl-α-D-mannopyranoside (27) (Table 2, Entry 9): A similar procedure as employed for the preparation of 26 by stirring both Bi(OTf)3 (12.5 mg, 0.019 mmol) and 2 (103.2 mg, 0.19 mmol) in CH2Cl2 (3 mL) at rt for 1 h gave the desired 1,1’-disaccharide 27 (PTLC; EtOAc/hexane = 1/3, 86.0 mg, 85%) as a colorless oil. [α] D25 +30.2° (c 0.88, CHCl3); 1H NMR δ 3.53-3.56 (2H, m, H-5), 3.60 (2H, dd, J = 2.1 Hz, J = 3.4 Hz, H-2), 3.63 (2H, dd, J = 2.1 Hz, J = 11.0 Hz, Ha-6), 3.69-3.72 (4H, m, Hb-6, H-3), 3.97 (2H, dd, J = 9.6 Hz, J = 10.3 Hz, H-4), 5.20 (2H, d, J = 2.1 Hz, H-1); 13C NMR δ 69.0, 72.1, 72.4, 72.6, 73.5, 74.0, 74.6, 75.3, 79.5, 93.3 (C-1, JC1-H1 = 167.5 Hz); HRMS (ESI) m/z calcd for 1085.4810 (C68H70O11+Na+), found 1085.4823. (Lit.,22 [α] D20 +40° (c 0.85, CHCl3); 13C NMR δ 93.25 (C-1)).
2,3,4,6-Tetra-O-benzyl-D-galactopyranosyl 2,3,4,6-Tetra-O-benzyl-D-galactopyranoside (28) (Table 2, Entry 10): A similar procedure as employed for the preparation of 26 by stirring both Bi(OTf)3 (6.4 mg, 0.0098 mmol) and 3 (52.9 mg, 0.098 mmol) in CH2Cl2 (3 mL) at 0 oC for 1 d gave the desired 1,1’-disaccharide 28 (PTLC; EtOAc/hexane = 1/3, 36.3 mg, 70%, a mixture of αα and αβ isomers) as a colorless oil. 1H NMR δ 4.54 (1H, d, J = 7.6 Hz, H-1αβ-β), 5.20 (1H, d, J = 3.4 Hz, H-1αβ-α), 5.28 (2H, d, J = 4.1 Hz, H-1αα); 13C NMR δ 93.5 (C-1αα), 99.8 (C-1αβ-α), 103.7 (C-1αβ-β); HRMS (ESI, isomer mixture) m/z calcd for 1085.4810 (C68H70O11+Na+), found 1085.4816. A part of αα isomer was isolated. αα Isomer: [α] D21 +74.5° (c 0.81, CHCl3). (Lit.,22 αα isomer: [α] D20 +147.7° (c 0.65, CHCl3); 13C NMR δ 93.54 (C-1); αβ isomer: 13C NMR δ 99.86 (C-1α), 103.82 (C-1β)).
2-Azido-3,4,6-tri-O-benzyl-2-deoxy-D-glucopyranosyl 2-Azido-3,4,6-tri-O-benzyl-2-deoxy-D-glucopyranoside (29) (Table 2, Entry 13): A similar procedure as employed for the preparation of 26 by stirring both Bi(OTf)3 (142.5 mg, 0.22 mmol) and 4 (103.8 mg, 0.22 mmol) in CH2Cl2 (3 mL) at rt for 1 d gave the desired 1,1’-disaccharide 29 (PTLC; EtOAc/hexane = 1/3, 53.8 mg, 53%, a mixture of αα, αβ, and ββ isomers) as a colorless oil. 1H NMR δ 4.33 (1H, d, J = 7.56 Hz, H-1αβ-β), 4.72 (1H, d, J = 8.25 Hz, H-1ββ), 5.17 (1H, d, J = 3.44 Hz, H-1αβ-α), 5.21 (1H, d, J = 3.44 Hz, H-1αα); 13C NMR δ 95.4 (C-1αα), 97.6 (C-1αβ-α), 101.3 (C-1αβ-β), 103.5 (C-1ββ); HRMS (ESI, isomer mixture) m/z calcd for 955.4001 (C54H56O9N6+Na+), found 955.4026.
2,3,5-Tri-O-benzyl-D-arabinofuranosyl 2,3,5-Tri-O-benzyl-D-arabinofuranoside (30) (Table 2, Entry 14): A similar procedure as employed for the preparation of 26 by stirring both Bi(OTf)3 (15.8 mg, 0.024 mmol) and 5 (101.2 mg, 0.24 mmol) in CH2Cl2 (3 mL) at 0 oC for 3.5 h gave the desired 1,1’-disaccharide 30αα (PTLC; EtOAc/hexane = 1/2, 78.2 mg, 79%) as a colorless oil and 30αβ (13.7 mg, 14%) as a colorless oil. αα Isomer: [α] D22 +54.9° (c 3.9, CHCl3); 1H NMR δ 3.61-3.64 (2H, m, Ha-5), 3.65-3.68 (2H, m, Hb-5), 3.94-3.96 (2H, m, H-3), 4.10-4.11 (2H, m, H-2), 4.24-4.27 (2H, m, H-4), 5.50 (2H, s, H-1); 13C NMR δ 69.7, 71.8, 72.1, 73.4, 81.2, 83.7, 87.9, 102.1 (C-1). HRMS (ESI) m/z calcd for 845.3660 (C52H54O9+Na+), found 845.3686. (Lit.,23 αα isomer: 1H NMR δ 5.51 (s)). αβ Isomer: [α] D25 +18.5° (c 0.69, CHCl3); 1H NMR δ 3.56-3.63 (3H, m, Ha-5α, Hb-5α, Ha-5β), 3.67 (1H, dd, J = 6.2 Hz, J = 10.3 Hz, Hb-5β), 3.99 (1H, dd, J = 2.8 Hz, J = 6.2 Hz, H-3α), 4.10-4.15 (4H, m, H-2α, H-2β, H-3β, H-4β), 4.30-4.32 (1H, m, H-4α), 5.18 (1H, d, J = 3.4 Hz, H-1β), 5.20 (1H, s, H-1α); 13C NMR δ 69.5 (C-5α), 71.7 (CH2Ph), 72.1 (CH2Ph), 72.2 (CH2Ph), 72.6 (CH2Ph), 72.7 (C-5β), 73.1 (CH2Ph), 73.3 (CH2Ph), 80.8 (C-4β), 81.4 (C-4α), 83.58 (C-3α), 83.62 (C-2β or C-3β), 84.56 (C-2β or C-3β), 87.8 (C-2α), 99.5 (C-1β), 106.1 (C-1α); HRMS (ESI) m/z calcd for 845.3660 (C52H54O9+Na+), found 845.3659.
2,3,5-Tri-O-benzyl-D-arabinofuranosyl 2,3,4,6-Tetra-O-benzyl-D-glucopyranoside (31) (Table 2, Entry 15): A similar procedure as employed for the preparation of 26 by stirring Bi(OTf)3 (11.0 mg, 0.017 mmol), 1 (135.7 mg, 0.25 mmol), and 6 (70.5 mg, 0.17 mmol) in CH2Cl2 (3 mL) at 0 oC for 3.5 h gave the desired 1,1’-disaccharide 31 (PTLC; EtOAc/hexane = 1/4, 117.3 mg, 74%, a mixture of αα, αβ, βα, and ββ (Glc/Ara linkages: 31/31/31/7) isomers) as a colorless oil. 1H NMR δ 4.62 (d, J = 8.2 Hz, H-1βα(Glc)), 4.78-4.80 (m, H-1ββ(Glc)), 5.20 (d, J = 3.4 Hz, H-1αβ(Glc)), 5.29 (d, J = 4.2 Hz, H-1’αβ(Ara)), 5.40 (1H, d, J = 3.4 Hz, H-1αα(Glc)), 5.42(1H, s, H-1’αα(Ara)), 5.43 (s, H-1’βα(Ara)), 5.60(d, J = 3.4 Hz, H-1’ββ(Ara));13C NMR δ 92.7 (C-1αα(Glc)), 96.1 (C-1’ββ(Ara)), 96.2 (C-1αβ(Glc)), 97.5 (C-1ββ(Glc)), 100.6 (C-1’αβ(Ara)), 101.4 (C-1βα(Glc)), 102.0 (C-1’αα(Ara)), 106.6 (C-1’βα(Ara)); HRMS (ESI, isomer mixture) m/z calcd for 965.4235 (C60H62O10+Na+), found 965.4237.
This paper is dedicated to Professor Emeritus Keiichiro Fukumoto on the occasion of his 75th birthday.
References
1. ‘Comprehensive Glycoscience. From Chemistry to Systems Biology,’ Vol. 1. ed. by J. P. Kamerling, G.-J. Boons, Y. C. Lee, A. Suzuki, N. Taniguchi, and A. G. J. Voragen, Elsevier Ltd., Oxford, 2007.
2. (a) K. Toshima and K. Tatuta, Chem. Rev., 1993, 93, 1503; CrossRef (b) A. V. Demchenko, Synlett, 2003, 1225. CrossRef
3. D. Gin, J. Carbohydr. Chem., 2002, 21, 645. CrossRef
4. (a) B. Wagner, M. Heneghan, G. Schnabel, and B. Ernst, Synlett, 2003, 1303; CrossRef (b) K. Toshima, H. Nagai, and S. Matsumura, Synlett, 1999, 1420; CrossRef (c) N. Aoyama and S. Kobayashi, Chem. Lett., 2006, 35, 238. Recent references cited therein. CrossRef
5. E. Fischer, Chem. Ber., 1893, 26, 2400.
6. L. Panza and L. Lay, ‘In Carbohydrates in Chemistry and Biology,’ Vol. 1, ed. by B. Ernst, G. W. Hart, and P. Sinay, Wiley-VCH, Weinheim, 2000, pp. 195-237. CrossRef
7. (a) K. Hiruma, T. Kajimoto, G. Weitz-Schmidt, I. Ollmann, and C.-H. Wong, J. Am. Chem. Soc., 1996, 118, 9265; CrossRef (b) H. Dohi, Y. Nishida, Y. Furuta, H. Uzawa, S.-I. Yokoyama, S. Ito, H. Mori, and K. Kobayashi, Org. Lett., 2002, 4, 355; CrossRef (c) Y. Hui and C.-H. T. Chang, Org. Lett., 2002, 4, 2245. CrossRef
8. (a) G. H. Posner and D. S. Bull, Tetrahedron Lett., 1996, 37, 6279; CrossRef (b) M. Nishizawa, D. M. Garcia, Y. Noguchi, K. Komatsu, S. Hatakeyama, and H. Yamada, Chem. Pharm. Bull., 1994, 42, 2400.
9. (a) Y. Oda and T. Yamanoi, Synthesis, 2007, 3021; CrossRef (b) T. Yamanoi, Y. Oda, S. Matsuda, I. Yamazaki, K. Matsumura, K. Katsuraya, M. Watanabe, and T. Inazu, Tetrahedron, 2006, 62, 10383; CrossRef (c) T. Yamanoi, S. Matsuda, I. Yamazaki, R. Inoue, K. Hamasaki, and M. Watanabe, Heterocycles, 2006, 68, 673; CrossRef (d) T. Yamanoi, K. Matsumura, S. Matsuda, and Y. Oda, Synlett, 2005, 2973; CrossRef (e) T. Yamanoi, Y. Oda, I. Yamazaki, M. Shinbara, K. Morimoto, and S. Matsuda, Lett. Org. Chem., 2005, 2, 242. CrossRef
10. T. Yamanoi, R. Inoue, S. Matsuda, K. Katsuraya, and K. Hamasaki, Tetrahedron: Asymmetry, 2006, 17, 2914. CrossRef
11. There were several glycosidation reports using Bi(OTf)3 as follows. (a) K. Ikeda, Y. Ueno, S. Kitani, R. Nishino, and M. Sato, Synlett, 2008, 1027; CrossRef (b) S. Valerio, A. Iadonisi, M. Adinolfi, and A. Ravida, J. Org. Chem., 2007, 72, 6097; CrossRef (c) M. Adinolfi, A. Iadonisi, A. Ravida, and S. Valerio, Tetrahedron Lett., 2006, 47, 2595; CrossRef (d) J. Babu, A. Khare, and Y. Vankar, Molecules, 2005, 10, 884; CrossRef (e) J. S. Yadav, B. V. S. Reddy, R. K. Srinivasa, L. Chandraiah, and V. Sunitha, Synthesis, 2004, 2523; CrossRef (f) K. Ikeda, Y. Torisawa, T. Nishi, J. Minamikawa, K. Tanaka, and M. Sato, Bioorg. Med. Chem., 2003, 11, 3073. CrossRef
12. T. Yamanoi, R. Inoue, S. Matsuda and K. Hamasaki, Lett. Org. Chem., 2008, 5, 30. CrossRef
13. Anhydrous calcium sulfate was used as a scavenger for water. We previously investigated the glycosidation catalyzed by a Lewis acid in presence of several anhydrous salts. T. Yamanoi, S. Nagamaya, H.-K. Ishida, J. Nishikido, and T. Inazu, Synth. Commun., 2001, 31, 899. CrossRef
14. X. Hao, A. Yoshida, and J. Nishikido, Green Chem., 2004, 6, 566. CrossRef
15. H. Nagai, K. Sasaki, S. Matsumura, and K. Toshima, Carbohydr. Res., 2005, 340, 337. CrossRef
16. B. A. Garcia and D. Y. Gin, J. Am. Chem. Soc., 2000, 122, 4269. CrossRef
17. E. Decoster, J.-M. Lacombe, J.-L. Strebler, B. Ferrari, and A. A. Pavia, J. Carbohydr. Chem., 1983, 2, 329. CrossRef
18. D. Crich and S. Sun, Tetrahedron, 1998, 54, 8321. CrossRef
19. K. Toshima, H. Nagai, K. Kusumi, K. Kawahara, and S. Matsumura, Tetrahedron, 2004, 60, 5331. CrossRef
20. F. Micheel, O. E. Brodde, and K. Reinking, Justus Leibigs Ann. Chem., 1974, 124.
21. H. Hori, Y. Nishida, H. Ohrui, and H. Meguro, J. Org. Chem., 1989, 54, 1346. CrossRef
22. A. A. Pavia, J.-M. Rocheville, and S. N. Ung, Carbohydr. Res., 1980, 79, 79. CrossRef
23. V. Dourtoglou and B. Gross, J. Carbohydr. Chem., 1983, 2, 57 CrossRef