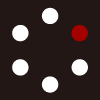
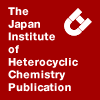
HETEROCYCLES
An International Journal for Reviews and Communications in Heterocyclic ChemistryWeb Edition ISSN: 1881-0942
Published online by The Japan Institute of Heterocyclic Chemistry
e-Journal
Full Text HTML
Received, 19th May, 2008, Accepted, 8th July, 2008, Published online, 10th July, 2008.
DOI: 10.3987/COM-08-S(F)18
■ Ring Contraction of α,β-Unsaturated Cyclic Amines with cis-Dihydroxylation at the α,β-Position
Samuel S. Libendi, Yosuke Demizu, Yoshihiro Matsumura, and Osamu Onomura*
Department of Pharmaceutical Sciences, Graduate School of Biomedical Sciences, Nagasaki University, 1-14 Bunkyo-machi, Nagasaki 852-8521, Japan
Abstract
α,β-Unsaturated cyclic amines are oxidized by OsO4 to afford α,β-cis-dihydroxylated compounds which are thermodynamically transformed into ring-opened keto-alcohols. The keto-alcohols are then cyclized to form synthetically useful ring-contracted cyclic amines.INTRODUCTION
Functionalized cyclic amines are versatile building blocks and intermediates for organic synthesis. There are several methods reported to date that achieve these.1 Ring contraction is one of these methods. Ever since Leonard et al found rearrangement of β-hydroxylated cyclic amines generated from β-oxo cyclic amines during the Clemmensen reduction,2 some methods for ring contraction via bicyclic aziridinium ion have been exploited.3 Recently, Sayre et al. reported acid catalyzed rearrangement of 1-benzyl-2-methyl-3-piperidone to 1-benzyl-2-acetylpyrrolidine, in which ring-opened keto-alcohol was proposed as a plausible intermediate.4 Now, we found that N-protected α,β-cis-dihydroxylated cyclic amines 2 which are formed by oxidation of the corresponding α,β-unsaturated compounds 1 with OsO4 are thermodynamically unstable and changed to ring-opened keto-alcohols 3. Acid catalyzed reaction of 3 afforded ring contracted products including functionalized cyclic imines (Scheme 1). Herein, we present the ring contraction of cyclic amines and subsequent formation of functionalized imines.
RESULTS AND DISCUSSION
Starting from readily commercially available cyclic amines 4a-f, we activated the α-position by electrochemical oxidation in methanol5 followed by acid catalyzed removal of methanol to afford α,β-unsaturated cyclic amines 1a-f.6,7 These results are summarized in Table 1.
Having successfully prepared 1a-f, we embarked on the task of functionalizing and subsequent ring opening of the product 2a-f. First, dihydroxylation of 1a-f using OsO4 followed by thermodynamically induced ring opening by use of elevated temperatures afforded 3a-f. The results are summarized in Table 2.
Analysis of the results obtained led us to conclude as follows: protecting groups influenced the ease of keto-alcohol formation, i.e. enamines protected by benzoyl group (Entries 1-4) required only 5 h to convert to keto-alcohols 3 compared to other protecting groups like phenoxycarbonyl and methoxycarbonyl which required 24 h to 48 h for complete reaction (Entries 5 and 6). Furthermore, ring stability played a role in the reaction. Five and seven membered amines (Entries 1, 4-6) were easily converted to keto-alcohols 3 with comparatively better yields to that of six membered amines (Entries 2 and 3).
Dihydroxylated products 2 formed by OsO4 have cis orientation. So, to find out if trans product can undergo this reaction we prepared trans product using electrochemical method.8 As shown in Scheme 2, the transformation of trans-2b to keto-alcohol 3b did not take place even at elevated temperatures of 70 oC for 48 h.
Based on these data, we propose that the mechanism for ring opening is as shown in Scheme 3. Under elevated temperatures, the cis-diols 2 are unstable and therefore tautomerize to more stable keto-alcohols 3 (Scheme 3).
Next, transforming 3a-f to synthetically useful intermediates or products was examined. We envisioned that in acidic conditions, the carbonyl group on 3f could be activated leading to an attack by the lone pairs of electrons on the nitrogen group thus forming α-hydroxyl-α-hydroxylmethylpiperidine 5 that has a quaternary carbon at the α-position which might be transformed to pharmaceutically important compounds (Scheme 4).9
To test this method, keto-alcohol 3f was dissolved in CH2Cl2 and methanesulfonic acid (1 equiv) was added to it dropwise and left to stir for 12 h. After workup, product 5 was obtained in 92% yield which was determined by NMR analysis. However, when 3b was subjected to similar reaction conditions, imine 6b was formed with almost 50% recovery of keto-alcohol 3b. Therefore, to drive the reaction to completion, MgSO4 was added to remove H2O. As shown in Table 3, benzoyl group migrated to the terminal hydroxyl group.
α-Benzoyloxymethylated cyclic imine 6b was obtained in good yield from 3b (Entry 1). Methoxycarbonyl substituent on keto-alcohol 3c was well tolerated (Entry 2). Moreover, 6-membered cyclic imine 6d was synthesized starting from 3d with good yield (Entry 3). Interestingly, when 3a was subjected to these reaction conditions, dimer 7 instead of a 4-membered cyclic imine was exclusively obtained in good yield (Scheme 5). Attempts to vary reaction conditions so as to attain an imine were futile.
Ketoalcohol 3e formed bicyclic compound 8 when subjected to acid catalyzed condensation reaction. MgSO4 did not affect the reaction (Scheme 6).
To demonstrate how the imines can be utilized in organic synthesis,9 6b and 6d were allylated using allyltrimethylsilane in the presence of π-allylpalladium chloride dimer10 and cyanated by trimethylsilyl cyanide catalyzed by β-cyclodextrin (Scheme 7).11
In conclusion, starting from simple cyclic amines, we have achieved ring contraction of 5-, 6- and 7- membered ring systems to functionalized 4-, 5- and 6-membered ones respectively through electrochemical and OsO4 oxidation. Finally, we have demonstrated the use of the imine products by allylation and cyanation.
EXPERIMENTAL
Electrochemical reactions were carried out using DC Power Supply (GP 050-2) of Takasago Seisakusho, Inc. 1H NMR spectra were measured on a Varian Gemini 300 and 400 spectrometer with TMS as an internal standard. 13C NMR spectra were measured on a Varian Gemini 400 spectrometer with TMS as an internal standard. IR spectra were obtained on a Shimadzu FTIR-8100A. Elemental analyses were carried out at the Center for Instrumental Analysis, Nagasaki University. Mass spectra were obtained on a JEOL JMS-DX 303 instrument.
All solvents were used as supplied without further purification.
General procedure for preparation of enecarbamates 1a-f
To a 200 mL beaker containing a stirring bar, 4 (100 mmol), MeOH (200 mL) and platinum electrodes was added Et4NBF4 (10 mmol, 2.18 g). The beaker type cell was then placed at 0 oC and current passed through as the reaction was monitored by TLC and NMR. Upon completion of reaction, MeOH was removed under vacuo and the residue dissolved in AcOEt (100 mL). H2O (100 mL) was added to the mixture and the organic layer separated, the aqueous layer was extracted by AcOEt (2 x 100 mL) and the organic layer combined, dried by anhyd. MgSO4, filtered and solvent removed under reduced pressure. The residue was then subjected to flash chromatography to afford methoxylated product. This product was then transferred to 100 mL flask containing a stirring bar and NH4Cl (10 mmol, 0.535 g). The flask was then transferred to an oil bath already preheated at 100 oC to generate MeOH as a side product which was removed under reduced pressure. On completion of reaction as determined by TLC and NMR, the residue was passed through a silica gel column to afford product 1 as oil.
Compounds 1a,12 1b,13 1c,6 and 1f14 are known compounds.
N-Benzoyl-2,3,4,5-tetrahydroazepin (1d)
1H NMR (300MHz, CDCl3) δ 7.59-7.34 (m, 5H), 6.80-6.66 and 6.28-6.10 (m, 1H), 5.38-5.20 and 5.13-4.98 (m, 1H), 4.10-3.50 (m, 2H), 2.35-2.20 (m, 2H), 2.00-1.70 (m, 4H). 13C NMR (100Hz, CDCl3) δ 169.58 (1C), 135.86 (1C), 132.69 (1C), 129.96 (1C), 128.26 (1C), 127.86 (2C), 116.56 (1C), 45.95 (1C), 27.71 (1C), 26.99 (1C), 24.58 (2C). IR ν cm-1 (neat): 2930, 1636, 1447, 1406, 1387, 1364. High Resolution Mass Spectrum [FAB(+)]: m/z calcd for C13H16NO [M+H]+ 202.1232, found: 202.1243.
N-Phenoxycarbonyl-2,3,4,5-tetrahydroazepin (1e)
1H NMR (300MHz, CDCl3) δ 7.41-32 (m, 2H), 7.24-7.08 (m, 3H), 6.71-6.57 (m, 1H), 5.23-5.10 (m, 1H), 3.93-3.74 (m, 2H), 2.40-2.20 (m, 2H), 1.95-1.65 (m, 4H). IR ν cm-1 (neat): 2928, 1719, 1701, 1497, 1420, 1377, 1196. High Resolution Mass Spectrum [FAB(+)]: m/z calcd for C13H16NO2 [M+H]+ 218.1181, found: 218.1162.
General procedure for preparation of keto-alcohols 3
To 1 (1 mmol) in MeCN (2 mL) and 50% N-methylmorpholine N-oxide in H2O (1.5 mmol) was added 4% OsO4 in H2O (0.01 mmol) and the mixture stirred at rt monitored by TLC. On completion of the reaction, the mixture was transferred to an oil bath set at 55 oC. The reaction progress was then monitored by TLC and upon completion; H2O (5 mL) was added and the resulting mixture extracted with AcOEt (3 x 10 mL). The combined organic layer was dried by MgSO4, filtered and solvent removed in vacuo.
Recrystallization from AcOEt and n-hexane gave white crystalline compounds 3a,b,d,e. Oily compounds 3c and 3f were purified by silica gel column chromatography (n-hexane:AcOEt = 1:3).
N-(4-Hydroxy-3-oxobutyl)benzamide (3a)
Mp 82 oC; 1H NMR (300MHz, CDCl3) δ 7.78-7.68 (m, 2H), 7.52-7.38 (m, 3H), 6.88-6.75 (br s, 1H), 4.27 (s, 2H), 3.80-3.70 (m, 2H), 3.21-2.98 (br s, 1H), 2.80 (t, J=6.3Hz, 2H). 13C NMR (100Hz, CDCl3) δ 209.68 (1C), 167.68 (1C), 134.00 (1C), 131.62 (2C), 128.54 (1C), 126.87 (2C), 68.30 (1C), 37.88 (1C), 34.45 (1C). IR ν cm-1 (neat): 3422, 2900, 1728, 1653, 1603, 1578. Anal. Calcd for C11H13NO3: C, 63.76; H, 6.32; N, 6.76. Found: C, 63.78; H, 6.37; N, 6.70.
N-(5-Hydroxy-4-oxopentyl)benzamide (3b)
Mp 53oC; 1H NMR (400MHz, CDCl3) δ 7.79-7.72 (m, 2H), 7.56-7.40 (m, 3H), 6.45-6.31 (br s, 1H), 4.27 (d, J=6.6Hz, 2H), 3.51 (q, J=8.4Hz, 2H), 3.03 (t, J=6.6Hz, 1H), 2.57 (t, J=9.2Hz, 2H), 2.03-1.94 (m, 2H). IR ν cm-1 (neat): 3422, 2934, 1719, 1638, 1578, 1541, 1491. Anal. Calcd for C12H15NO3: C, 65.14; H, 6.83; N, 6.33. Found: C, 65.51; H, 6.67; N, 6.25.
Methyl 2-(N-benzoylamino)-6-hydroxy-5-oxohexanoate (3c)
Oil; 1H NMR (300MHz, CDCl3) δ 7.80 (d, J=7.2Hz, 2H), 7.58-7.39 (m, 3H), 7.20-7.12 (m, 1H), 4.86-4.74 (m, 1H), 4.24 (d, J=2.4Hz, 2H), 3.77 (s, 3H), 3.56-3.15 (br s, 1H), 2.71-2.48 (m, 2H), 2.41-2.30 (m, 1H), 2.18-2.03 (m, 1H). IR ν cm-1 (neat): 3422, 3063, 2953, 2361, 1747, 1653, 1541, 1491. Anal. Calcd for C14H17NO5: C, 60.21; H, 6.14; N, 5.02. Found: C, 60.51; H, 6.26; N, 4.71.
N-(6-Hydroxy-5-oxohexyl)benzamide (3d)
Mp 102oC; 1H NMR (300MHz, CDCl3) δ 7.78 (d, J=6.9Hz, 2H), 7.52-7.39 (m, 3H), 6.41-6.22 (br s, 1H), 4.26 (d, J=4.8Hz, 2H), 3.47 (q, J=6.6Hz, 2H), 3.13 (t, J=4.8Hz, 1H), 2.50 (t, J=7.2Hz, 2H), 1.78-1.57 (m, 4H). 13C NMR (100Hz, CDCl3) δ 209.45 (1C), 167.50 (1C), 134.51 (1C), 131.35 (2C), 128.49 (1C), 126.75 (2C), 68.17 (1C), 39.42 (1C), 37.67 (1C), 29.14 (1C), 20.65 (1C). IR ν cm-1 (neat): 3422, 2936, 2869, 2357, 1723, 1717, 1682. High Resolution Mass Spectrum [FAB(+)]: m/z calcd for C13H18NO3 [M+H]+ 236.1287, found: 236.1276.
6-Hydroxy-5-oxo-N-phenoxycarbonylhexylamine (3e)
Mp 53-54oC; 1H NMR (400MHz, CDCl3) δ 7.35 (t, J=7.6Hz, 2H), 7.19 (t, J=7.6Hz, 1H), 7.11 (d, J=7.2Hz, 2H), 5.39-5.31 (br s, 1H), 4.22 (s, 2H), 3.38-3.25 (br s, 1H), 3.23 (q, J=6.4Hz, 2H), 2.43 (t, J=7.2Hz, 2H), 1.80-1.50 (m, 4H). 13C NMR (100Hz, CDCl3) δ 154.68 (1C), 150.93 (1C), 129.18 (2C), 125.19 (2C), 121.59 (1C), 121.48 (1C), 68.04 (1C), 40.52 (1C), 37.52 (1C), 29.10 (1C), 20.35 (1C). IR ν cm-1 (neat): 3328, 3046, 2938, 1744, 1705, 1595. Anal. Calcd for C13H17NO4: C, 62.14; H, 6.82; N, 5.57. Found: C, 61.89; H, 6.53; N, 5.39.
6-Hydroxy-N-methoxycarbonyl-5-oxohexylamine (3f)
1H NMR (300MHz, CDCl3) δ 4.80-4.61 (br s, 1H), 4.25 (d, J=4.5Hz, 2H), 3.66 (s, 3H), 3.25-3.11 (m, 2H), 3.08 (t, J=3.6Hz, 1H), 2.46 (t, J=7.2Hz, 2H), 1.74-1.44 (m, 4H). 13C NMR (100Hz, CDCl3) δ 209.45 (1C), 157.12 (1C), 68.11 (1C), 52.04 (1C), 40.41 (1C), 37.66 (1C), 29.42 (1C), 20.45 (1C). IR ν cm-1 (neat): 3430, 3330, 2959, 2884, 1717, 1655, 1539, 1410. High Resolution Mass Spectrum [FAB(+)]: m/z calcd for C8H16NO4 [M+H]+ 190.1080, found: 190.1078.
General procedure for cyclization of keto-alcohols 3.
To 3 (1 mmol) in CH2Cl2 (15 mL) and anhyd. MgSO4 (1.5 mmol) stirring at rt, was added dropwise MeSO3H (2 mmol) and the mixture left to stir for 9 h. The reaction was then quenched using sat. aq. NaHCO3 (10 mL) and extracted by AcOEt (3 x 10 mL). The combined organic layer was dried using MgSO4, filtered and solvent removed in vacuo. The resulting product was purified by silica gel column chromatography (n-hexane:AcOEt = 1:2) to afford products 6-8.
2-Hydroxy-2-hydroxymethyl-N-methoxycarbonylpiperidine (5)
On silica gel 5 is unstable thus decomposes. So, the crude sample was analysed.
1H NMR (300MHz, CDCl3) δ 4.59 (s, 2H), 3.85-3.71 (m, 1H), 3.81 (s, 3H), 3.68-3.57 (m, 3H), 2.20-2.09 (m, 2H), 1.81-1.50 (m, 4H). 13C NMR (100Hz, CDCl3) δ 164.88 (1C), 155.44 (1C), 70.78 (1C), 54.81 (1C), 48.90 (1C), 25.97 (1C), 21.63 (1C), 18.65 (1C). IR νcm-1 (neat): 3596, 3430, 2990, 2857, 1800, 1709, 1667. High Resolution Mass Spectrum [EI(+)]: m/z calcd for C8H15NO4 [M]+ 189.1001, found 189.0989.
2-Benzoyloxymethyl-1,2-didehydropyrrolidine (6b)
Oil; 1H NMR (400MHz, CDCl3) δ 8.09 (d, J=7.3Hz, 2H), 7.59 (t, J=7.3Hz, 1H), 7.46 (t, J=7.3Hz, 2H), 5.07 (s, 2H), 3.93 (t, J=7.8Hz, 2H), 2.63 (t, J=8.3Hz, 2H), 2.04-1.91 (m, 2H). IR ν cm-1 (neat): 3063, 3032, 2953, 2349, 1918, 1728, 1662. Anal. Calcd for C12H13NO2: C, 70.92; H, 6.45; N, 6.89. Found: C, 70.67; H, 6.57; N, 6.75.
2-Benzoyloxymethyl-1,2-didehydro-5-methoxycarbonylpyrrolidine (6c)
Oil; 1H NMR (400MHz, CDCl3) δ 8.08 (d, J=7.3Hz, 2H), 7.59 (t, J=7.8Hz, 1H), 7.46 (t, J=7.3Hz, 2H), 5.14 (s, 2H), 4.80 (t, J=6.8Hz, 1H), 3.78 (s, 3H), 2.90-2.80 (m, 1H), 2.75-2.65 (m, 1H), 2.33-2.24 (m, 1H), 2.21-2.11 (m, 1H). 13C NMR (100Hz, CDCl3) δ 176.97 (1C), 165.96 (1C), 133.37 (1C), 129.80 (2C), 129.40 (1C), 128.48 (2C), 74.34 (1C), 63.92 (1C), 52.32 (1C), 36.00 (1C), 29.67 (1C), 25.78 (1C). IR ν cm-1 (neat): 2955, 2851, 1730, 1653, 1601, 1451, 1435, 1316, 1271. High Resolution Mass Spectrum [FAB(+)]: m/z calcd for C14H16NO4 [M+H]+ 262.1079, found: 262.1103.
2-Benzoyloxymethyl-1,2-didehydropiperidine (6d)
Oil; 1H NMR (300MHz, CDCl3) δ 8.09 (d, J=6.9Hz, 2H), 7.60-7.38 (m, 3H), 4.81 (s, 2H), 3.70-3.59 (m, 2H), 2.29-2.19 (m, 2H), 1.81-1.57 (m, 4H). IR νcm-1 (neat): 3063, 3032, 2953, 2349, 1918, 1728, 1662. Anal. Calcd for C13H15NO2: C, 71.87; H, 6.96; N, 6.45. Found: C, 72.01; H, 6.75; N, 6.38.
1,10-Diaza-N,N-dibenzoyl-6,12-dioxodispiro[3.2.3.2]dodecane (7)
Mp 128-129 oC; 1H NMR (300MHz, CDCl3) δ 8.00 (d, J=6.3Hz, 4H), 7.50-7.33 (m, 6H), 4.11 (d, J=12Hz, 2H), 3.92-3.61 (m, 4H), 3.79 (d, J=11.7Hz, 2H), 2.00-1.91 (m, 2H), 1.80-1.71 (m, 2H). 13C NMR (100Hz, CDCl3) δ 152.84 (2C), 133.35 (2C), 130.63 (2C), 128.17 (4C), 126.91 (4C), 92.75 (2C), 66.17 (2C), 38.83 (2C), 27.02 (2C). IR ν cm-1 (neat): 3306, 2926, 1661, 1443, 1364, 1277, 1186. High Resolution Mass Spectrum [EI(+)]: m/z calcd for C22H22N2O4 [M]+ 378.1579, found 378.1570.
1-Aza-6-hydroxy-8-oxa-9-oxo-[4.3.0]bicyclononane (8)
Mp 115 oC; 1H NMR (300MHz, CDCl3) δ 4.29 (d, J=9.6Hz, 1H), 4.12 (d, J=9.6Hz, 1H), 4.18-3.91 (m, 1H), 3.65 (dd, J=9 and 3.9Hz, 1H), 3.16 (td, J=9.9 and 3.3Hz, 1H), 2.12-2.02 (m, 1H), 1.90-1.62 (m, 3H), 1.55-1.36 (m, 2H). 13C NMR (100Hz, CDCl3) δ 156.18 (1C), 84.59 (1C), 50.76 (1C), 37.60 (1C), 34.70 (1C), 24.01 (1C), 19.00 (1C). IR ν cm-1 (neat): 3370, 2950, 1765, 1597, 1367, 1285, 1242. Anal. Calcd for C7H11NO3: C, 53.49; H, 7.05; N, 8.91. Found: C, 53.78; H, 6.88; N, 8.51.
General procedure for allylation of imines10
To a solution of 6b or 6d (0.5 mmol) and π-allyl PdCl2 dimer (0.025 mmol) in n-hexane (2 mL) was added allyltrimethylsilane (1.0 mmol). The resulting mixture was stirred for about half an hour, and then TBAF (0.25 mmol, 1.0M solution in THF) and THF (0.25 mL) were added. The reaction mixture became two phases: the upper phase was a homogenous n-hexane-THF solution and the bottom phase contained a TBAF solution. The mixture was stirred for 24 h at rt. The reaction progress was monitored by TLC. After imine was consumed completely, the reaction was quenched with water. The reaction mixture was extracted with AcOEt. The organic layer was dried over anhydrous MgSO4 and concentrated. The crude product was then dissolved in CH2Cl2 (2 mL), Et3N (1.2 mmol) was added and the resulting mixture was stirred at room temperature as ClCO2Me (1.0 mmol) was added dropwise, stirring continued for 1 h as reaction progress was checked by TLC. On completion of reaction, H2O (3 mL) was added and the mixture extracted using AcOEt. The organic layer was dried over MgSO4, concentrated and then purified over silica gel column chromatography (n-hexane : AcOEt = 5 : 1) to afford an oil.
2-Allyl-2-benzoyloxymethyl -N-methoxycarbonylpyrrolidine (9b)
Oil; 1H NMR (400MHz, CDCl3) δ 8.01 (d, J=7.3Hz, 2H), 7.57 (t, J=7.3Hz, 1H), 7.45 (t, J=7.3Hz, 2H), 5.92-5.67 (m, 1H), 5.25-5.00 (m , 2H), 4.72-4.20 (m, 2H), 3.53 and 3.51 (s, 3H), 3.61-3.40 (m, 2H), 2.95-2.70 (m, 1H), 2.53-2.23 (m, 1H), 2.18-1.98 (m, 2H), 1.91-1.73 (m, 2H). IR ν cm-1 (neat), 2959, 2878, 1721, 1698, 1640, 1601, 1449, 1375, 1271. High Resolution Mass Spectrum [FAB(+)]: m/z calcd for C17H22NO4 [M+H]+ 304.1548, found: 304.1548.
2-Allyl-2-benzoyloxymethyl -N-methoxycarbonylpiperidine (9d)
Oil; 1H NMR (400MHz, CDCl3) δ 7.99 (d, J=3.6Hz, 2H), 7.52 (t, J=7.2Hz, 1H), 7.41 (t, J=7.6Hz, 2H), 5.88-5.70 (m, 1H), 5.18-5.08 (m, 2H), 4.74 (d, J=11.2Hz, 1H), 4.53 (d, J=11.2Hz, 1H), 3.62 (s, 3H), 3.68-3.58 (m, 1H), 3.50-3.40 (m, 1H), 2.99 (dd, J=7.2 and 6.8Hz, 1H), 2.49 (dd, J=8 and 5.6Hz, 1H), 1.88-1.73 (m, 2H), 1.68-1.58 (m, 4H). 13C NMR (100Hz, CDCl3) δ 166.06 (1C), 156.50 (1C), 133.16 (1C), 132.80 (1C), 130.14 (1C), 129.44 (2C), 128.27 (2C), 118.50 (1C), 67.50 (1C), 59.07 (1C), 52.17 (1C), 41.97 (1C), 39.81 (1C), 29.74 (1C), 23.07 (1C), 17.47 (1C). IR ν cm-1 (neat), 2951, 1725, 1698, 1603, 1441, 1383, 1275, 1192, 1117. High Resolution Mass Spectrum [FAB(+)]: m/z calcd for C18H24NO4 [M+H]+ 318.1706, found: 318.1700.
General procedure for cyanation11
To β-cyclodextrin (0.1 mmol) dissolved in water (10 mL) was added 6b or 6d (1.0 mmol) in MeOH (1 mL) followed by trimethylsilyl cyanide (1.0 mmol) and the mixture stirred at rt until the reaction was complete (2 h). The organic material was extracted with AcOEt, dried and concentrated under reduced pressure, and the resulting product, though seen as single compound by TLC, was further purified by passing over a column of silica gel. After extraction with AcOEt, the aqueous phase was lyophilized to get back β-cyclodextrin.
2-Benzoyloxymethyl-2-cyanopyrrolidine (10b)
Oil; 1H NMR (400MHz, CDCl3) δ 8.08 (d, J=7.3Hz, 2H), 7.60 (t, J=7.3Hz, 1H), 7.46 (t, J=7.8Hz, 2H), 4.46 (d, J=10.7Hz, 1H), 4.35 (d, J=11.2Hz, 1H), 3.31-3.11 (m, 2H), 2.89-2.60 (m, 1H), 2.40-2.22 (m, 1H), 2.13-1.84 (m, 3H). 13C NMR (100Hz, CDCl3) δ 165.77 (1C), 133.44 (2C), 129.74 (2C), 129.13 (1C), 128.46 (1C), 121.58 (1C), 68.37 (1C), 59.74 (1C), 45.63 (1C), 34.38 (1C), 23.43 (1C). IR ν cm-1 (neat), 3352, 3067, 2953, 2226, 1725, 1638, 1601, 1451, 1269. High Resolution Mass Spectrum [FAB(+)]: m/z calcd for C13H15N2O2 [M+H]+ 231.1133, found: 231.1128.
2-Benzoyloxymethyl-2-cyanopiperidine (10d)
Mp 83-85 oC; 1H NMR (400MHz, CDCl3) δ 8.07 (d, J=7.3Hz, 2H), 7.60 (t, J=7.8Hz, 1H), 7.47 (t, J=7.8Hz, 2H), 4.44 (d, J=10.7Hz, 1H), 4.28 (d, J=10.8Hz, 1H), 3.12-2.95 (m, 2H), 2.31-2.12 (br s, 1H), 2.15-1.45 (m, 6H). 13C NMR (100Hz, CDCl3) δ 165.69 (1C), 133.50 (2C), 129.78 (2C), 129.12 (1C), 128.53 (1C), 119.51 (1C), 69.65 (1C), 56.69 (1C), 43.11 (1C), 31.77 (1C), 24.75 (1C), 20.99 (1C). IR ν cm-1 (neat), 3333, 3065, 2946, 2863, 2222, 1736, 1601, 1586, 1451, 1379, 1285. High Resolution Mass Spectrum [FAB(+)]: m/z calcd for C14H17N2O2 [M+H]+ 245.1290, found: 245.1283.
ACKNOWLEDGEMENTS
This work was supported by a Grant-in-Aid for Scientific Research (C) (19550109) from Japan Society for the Promotion of Science and a Grant-in-Aid for Young Scientists (B) (19790017) from the Ministry of Education, Science, Sports and Culture, Japan.
References
1. J. C. Hubert, J. B. P. A. Wijinberg, and W. N. Speckamp, Tetrahedron, 1975, 31, 1437; CrossRef T. Shono, Y. Matsumura, and K. Tsubata, J. Am. Chem. Soc., 1981, 103, 1172; CrossRef W. N. Speckamp and M. J. Moolenaar, Tetrahedron, 2000, 56, 3817; CrossRef S. S. Libendi, Y. Demizu, Y. Matsumura, and O. Onomura, Tetrahedron, 2008, 64, 3935. CrossRef
2. N. J. Leonard and W. C. Wildman, J. Am. Chem. Soc., 1949, 71, 3089. CrossRef
3. L. Duhamel and J.-M. Poirier, J. Org. Chem., 1979, 44, 3576; CrossRef K. A. Tehrani, K. V. Syngel, M. Boelens, J. Contreras, N. De. Kimpe, and D. W. Knight, Tetrahedron Lett., 2000, 41, 2507; CrossRef D. Trancard, J.-B. Tout, T. Giard, I. Chichaoui, D. Cahard, and J.-C. Plaquevent, Tetrahedron Lett., 2000, 41, 3843; CrossRef H. Tanaka, H. Sakagami, and K. Ogasawara, Tetrahedron Lett., 2002, 43, 93; CrossRef T. Mino, A. Saito, Y. Tanaka, S. Hasegawa, Y. Sato, M. Sakamoto, and T. Fujita, J. Org. Chem., 2005, 70, 1937. CrossRef
4. L. M. Sayre, V. D. Nadkarni, H. Jeon, and S. Zhao, Tetrahedron, 2006, 62, 6361. CrossRef
5. T. Shono, H. Hamaguchi, and Y. Matsumura, J. Am. Chem. Soc., 1975, 97, 4264. CrossRef
6. T. Shono, Y. Matsumura, K. Tsubata, Y. Sugihara, A. Yamane, T. Kanazawa, and T. Aoki, J. Am. Chem. Soc., 1982, 104, 6697. CrossRef
7. O. Onomura, Y. Kanda, M. Imai, and Y. Matsumura, Electrochim. Acta, 2005, 50, 4926. CrossRef
8. S. S. Libendi, T. Ogino, O. Onomura, and Y. Matsumura, J. Electrochem. Soc., 2007, 154, E31.. CrossRef
9. D. Xiao, J. B. lavey, A. Palani, C. Wang, G. R. Aslanian, A. J. Kozlowski, N. Shin, T. A. Mcphil, G. P. Randolph, E. J. Lachowicz, and A. R. Duffy, Tetrahedron Lett., 2005, 46, 7653. CrossRef
10. K. Nakamura, H. Nakamura, and Y. Yamamoto, J. Org. Chem., 1999, 64, 2614. CrossRef
11. K. Surendra, N. S. Krishnaveni, A. Mahesh and K. R. Rao, J. Org. Chem., 2006, 71, 2532. CrossRef
12. Y. Matsumura, J. Terauchi, T. Yamamoto, T. Konno, and T. Shono, Tetrahedron, 1993, 49, 8503. CrossRef
13. S. Furukubo, N. Moriyama, O. Onomura, and Y. Matsumura, Tetrahedron Lett., 2004, 45, 8177. CrossRef
14. T. Shono, Y. Matsumura, O. Onomura, M. Ogaki, and T. Kanazawa, J. Org. Chem., 1987, 52, 536 CrossRef