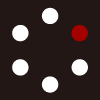
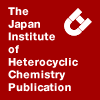
HETEROCYCLES
An International Journal for Reviews and Communications in Heterocyclic ChemistryWeb Edition ISSN: 1881-0942
Published online by The Japan Institute of Heterocyclic Chemistry
e-Journal
Full Text HTML
Received, 24th June, 2008, Accepted, 15th August, 2008, Published online, 18th August, 2008.
DOI: 10.3987/COM-08-S(F)31
■ Reaction of 1-Azabicyclo[1.1.0]butanes with 2,3-Dicyanofumarates; Interception of the Intermediate Zwitterions with Methanol
Grzegorz Mloston,* Malgorzata Celeda, Anthony Linden, and Heinz Heimgartner*
Institute of Organic Chemistry, University of Zürich, Winterthurerstrasse 190, CH-8057 Zürich, Switzerland
Abstract
The reaction of 3-phenyl-1-azabicyclo[1.1.0]butane (1c) with 2,3-dicyanofumarates ((E)-5) in dichloromethane at room temperature yields mixtures of cis- and trans-2,3-dicyano-4-phenyl-1-azabicyclo[2.1.1]hexane- 2,3-dicarboxylates (cis,trans-4). The proposed two-step reaction mechanism via a zwitterionic intermediate of type (6) is supported by trapping experiments with methanol: when the reactions of 1-azabicyclo[1.1.0]butanes (1) with dimethyl 2,3-dicyanofumarate ((E)-5a) are carried out in methanol, dimethyl (E)-2-(azetidin-1-yl)-3-cyanobut-2-enedioates (7) are formed as the only products.INTRODUCTION
The smallest bicyclic nitrogen compounds are azabicyclobutanes, and 1-azabicyclo[1.1.0]butanes (1) are known as relatively stable substances.1 The parent compound (1a) was prepared by the two-fold intramolecular substitution of either 1,3-dibromopropan-2-amine2 or the isomeric 2,3-dibromopropan-1-amine.3 The cyclizations were performed by using KOH or BuLi as a base. On the other hand, 3-aryl substituted 1-azabicyclo[1.1.0]butanes (1) are conveniently available by the reaction of 3-aryl-2H-azirines with dimethylsulfonium methanide.4,5 The strained bicyclic system (1) easily undergoes addition reactions with HX along the N(1),C(3) bond yielding diverse azetidine derivatives (2).6 Electrophilic agents such as azido- or chloroformates add in a similar manner to 1 yielding azetidine-1-carboxylates (3).7 Analogous reactions with chlorodithioformates led to hitherto unknown azetidine-1-dithiocarboxylates,8 and sulfanyl as well as sulfinyl chlorides add easily to 1c across the N(1),C(3) bond to give sulfenyl- and sulfinylamides, respectively.9 In a very recent paper, the unsubstituted 1a was shown to undergo a smooth 1,3-addition with the thiol form of azaheterocyclic thiones to give products of type 2 with X = S-Hetaryl.10 This type of azetidine derivative is also an attractive building block for the preparation of biologically active quinolone carboxylic acids bearing an azetidine residue. In the same paper, the reactions of 1a with nucleophilic secondary amines in the presence of Mg(ClO4)2 affording 3-aminoazetidines (2) (R = H, X = R2N) are described.
The first ring enlargement of 1c to give a 1-azabicyclo[2.1.1]hexane (4a) was achieved by the treatment of 1c with the extremely electron deficient dimethyl 2,3-dicyanofumarate ((E)-5a).11 The analysis of the crude product showed that the reaction led to a mixture of cis- and trans-4a in a ratio of 1:3 (Scheme 2). The same ratio of the ring-enlarged products was obtained starting with 1c and dimethyl 2,3-dicyanomaleate ((Z)-5a).
In the previous paper, we proposed that the reactions occur stepwise via the zwitterionic intermediate 6c, which lives long enough to undergo rotation about the C(2),C(3)-bond of the former olefinic component. The subsequent 1,5-ring closure results in the formation of the same mixture of cis- and trans-4a starting either from (E)- or (Z)-5a.
To get more insight into the mechanism of this unprecedented ring enlargement, reactions of differently substituted 1-azabicyclo[1.1.0]butanes (1) with (E)-5a in the presence of methanol as a trapping reagent were studied.
RESULTS AND DISCUSSION
For the present study, three different 1-azabicyclo[1.1.0]butanes were used, i.e., 1b, 1c, and 2,2-dimethyl-3-phenyl-1-azabicyclo[1.1.0]butane (1d). Along with the dimethyl ester (E)-5a, the corresponding diethyl and diisopropyl esters ((E)-5b) and ((E)-5c) were also applied.
Analogously to 5a, the reactions of 1c with (E)-5b and (E)-5c were carried out in CH2Cl2 at room temperature. After ca. 15 h, using equimolar amounts of reagents, complete conversion of 1c was confirmed by 1H-NMR spectroscopy. Chromatographic separation of the crude mixtures gave the expected stereoisomers of 1-azabicyclo[2.1.1]hexane 4b and 4c, respectively, in fair yields (Scheme 3). In both cases, the ratio of cis- to trans-adduct was established as ca. 2:3 after chromatographic workup.
In the case of 4c, the structure of the crystalline product isolated from the less polar fraction (minor isomer), was established by X-ray crystallography as cis-4c (Figure 1).
For comparison, the reactions of (E)-5a with 1b and 1d were carried out with the aim of testing the influence of the substitution pattern of 1. In both cases, the 1H-NMR spectra of the crude products confirmed the absence of starting compounds 1b or 1d, and the formation of a complex mixture of polymeric products was very likely. Attempted chromatographic separations of the products, using preparative plates coated with silica, failed.
In order to trap the postulated zwitterion, which is formed from 1c and (E)-5a, the reaction was performed in methanol, leading to a new product. The 1H-NMR spectrum of the crude mixture revealed the presence of three MeO signals located at 3.05, 3.74, and 3.92 ppm. After isolation and purification of the sole product, the MeO signals in the 1H-NMR were unchanged. In addition, the 13C-NMR spectrum showed the presence of a single C≡N absorption at 116.7 ppm as well as three signals at 158.5, 161.8, and 165.0 ppm. Whereas two of these signals belong to the ester C=O groups, the third one is attributed to the strongly deshielded C(2)-atom. The C(3)-atom absorbs at higher field, and the corresponding signal appears at 70.8 ppm. Based on this observation and supported by the mass spectrum and the elemental analysis, the structure of 2-(azetidin-1-yl)butenedioate (7b) is proposed (Scheme 4). Neither spectroscopically nor during the workup could the presence of the previously described bicyclic products (cis/trans-4a) be detected.
Interestingly, analogous interception experiments performed with 1b and 1d also gave the corresponding products (7b) and (7c), respectively, although in the absence of the trapping agent, no products of type 4, formed via the intramolecular reaction, were observed.
The configuration of the C=C bonds of 7a–c is yet unknown. Surprisingly, reactions of amines with dicyanofumarates are rarely reported. The structure of the product obtained with 4-nitroaniline is believed to be the (Z)-isomer.13 The reactions with 1,2-diamines led to (Z)-configured 3-[α-cyano-α-(alkoxycarbonyl)methylidene]piperazin-2-ones.14 Similarly, cyanoacetic acid hydrazide and semicarbazide, respectively, reacted with dicyanofumarates to give heterocyclic products via an addition-cyclization-elimination sequence.15 In all of these cases, the initially formed products were neither isolated nor detected. However, the crystal structure of the enamine obtained from the reaction of dimethyl dicyanofumarate with pyrrolidine showed the (E)-configuration with a cis-orientation of the ester groups.16 Because the reaction of 1 and pyrrolidine, respectively, with dicyanofumarates occur via an analogous zwitterionic intermediate, we propose that the C=C bond in compounds (7) is also (E)-configured.
It is worth discussing the NMR-data of products (7a–c). The 1H-NMR spectrum of 7c shows two Me signals for Me2C(2') at 0.98 and 1.56 ppm and an AB-system for H2C(4'). In the 13C-NMR spectrum of this compound, C(2) and C(3) absorb at 157.1 and 70.4 ppm, respectively, confirming the ‘push-pull effect’ in the structure of 7c. The 1H-NMR spectrum of 7a is characterized by the presence of two AB-systems at 3.94/4.09 and 4.08/4.42 ppm for H2C(2') and H2C(4'), and, in the 13C-NMR spectrum, C(2) and C(3) absorb at 158.7 and 75.9 ppm, respectively. The presence of two AB-systems for the two azetidine CH2 groups is a clear evidence for hindered rotation about the N(1'),C(2)-bond, i.e., its partial double bond character (Scheme 4). Finally, 7b shows a similar pattern of signals in the 13C-NMR spectrum (158.5 and 70.8 ppm for C(2) and C(3), resp.). On the other hand, the two azetidine CH2 groups appear as an AB-system at 4.89/5.00 and, unexpectedly, as a broad singlet at 4.42 ppm.
The trapping experiments with methanol confirm the proposed mechanism of the ring enlargement in the reaction of 3-phenyl-1-azabicyclo[1.1.0]butanes (1) with 2,3-dicyanofumarates ((E)-5). The intermediate zwitterion of type 6 (Scheme 2) undergoes ring closure to give 4 in the case of the phenyl derivative (1c). Bulkier substituents in the ester ((E)-5) do not influence the course of the reaction. On the other hand, the replacement of the phenyl group in 1c by an ethyl group in 1b, or the presence of two methyl groups in 1d, prevents the formation of the ring-enlarged bicyclic product. Nevertheless, in all cases studied, the initially formed zwitterion of type 6 is efficiently trapped by methanol. The 1:1:1-adducts spontaneously eliminate HCN and could never be detected. It is worth mentioning that the formation of the final products (7) occurs stereoselectively to give exclusively the (E)-configured isomers.
The reactions of (E)-5 with electron-rich thiocarbonyl ylides (Figure 2) has been studied extensively.17 In this case, the appearance of a zwitterionic intermediate was evidenced by the 1,7-dipolar ring closure to give a reactive ketene imine, which subsequently was trapped by methanol. Based on these results, the zwitterion (6), in addition to the 1,5-cyclization to give 4a, could be expected to undergo a competitive 1,7-cyclization to a ketenimine (8) (Scheme 5). Trapping of the latter with methanol would yield the bicyclic iminoether (9). In the present study, however, products of type 9 have never been observed, and the 1,5-cyclization of 6 is highly preferred. In contrast to the zwitterions formulated in the reaction of (E)-5 with a sterically hindered thiocarbonyl ylide,17 the intermediate of type 6 reacts with methanol quickly, and neither five- nor seven-membered bicyclic products are formed.
In conclusion, the described results show that strained 1-azabicyclo[1.1.0]butanes (1) easily react with electron-deficient 2,3-dicyanofumarates ((E)-5), and the zwitterions of type 6 are formed as intermediates. In the absence of methanol, highly stabilized structures with the phenyl substituent at C(3) undergo 1,5-ring closure to yield 1-azabicyclo[2.1.1]hexanes (4). The presence of two methyl groups at C(2) of the 1-azabicyclo[1.1.0]butane prevents this ring-closure. Again, no formation of the ring-enlarged bicyclic system of type 4 was observed in the case of the 3-ethyl derivative (1b). However, the intermediacy of a zwitterion (6) is demonstrated by trapping experiments with methanol. The adducts formed thereby spontaneously eliminate HCN to yield the ‘push-pull stabilized’ products (7) in a stereoselective manner.
EXPERIMENTAL
General remarks. Melting points were determined in a capillary using a MEL-TEMP II apparatus (Aldrich) and are uncorrected. IR spectra were recorded with a FT-IR NEXUS instrument as KBr pellets or as films, and the positions of absorption bands are given in cm–1. 1H-NMR and 13C-NMR spectra were recorded on a BRUKER-AC-300 (1H at 300 MHz and 13C at 75 MHz) instrument in CDCl3 solutions using TMS (δ = 0 ppm) as an internal standard; chemical shifts (δ) in ppm. MS spectra were recorded on a LKB-2091 spectrometer using chemical ionization (CI-MS; with NH3) or electrospray (ESI) method; m/z (rel. %). Elemental analyses were performed in the Analytical Laboratory of the University of Zürich or in the Laboratory of the Polish Academy of Sciences (CBMiM) in Lodz.
Starting materials. For the preparation of the starting materials, known procedures were applied: 3-ethyl-1-azabicyclo[1.1.0]butane (1b),2 3-phenyl-1-azabicyclo[1.1.0]butane (1c),4 2,2-dimethyl-3- phenyl-1-azabicyclo[1.1.0]butane (1d),4 and dimethyl 2,3-dicyanofumarates ((E)-5a).18 Diethyl ((E)-5b) and diisopropyl 2,3-dicyanofumarate ((E)-5c) were prepared in analogy to (E)-5a using diethyl and diisopropyl cyanoacetate, respectively, as substrates for the reaction with thionyl chloride (see ref.18).
Synthesis of 1-azabicyclo[2.1.1]hexanes 4b and 4c. To a magnetically stirred solution of 1 mmol of (E)-5b (or (E)-5c) in 1 mL of CH2Cl2 at rt, 131 mg (1 mmol) of 1c dissolved in 1 mL of CH2Cl2 was added in small portions. The homogenous solution was left at rt overnight. Next day, the solvent was evaporated to dryness and crude products obtained as viscous oils were separated on preparative plates covered with SiO2 and CH2Cl2 as the eluent. Two well separated fractions were isolated and additionally purified by crystallization; yields refer to amounts obtained after chromatography.
trans-Diethyl 2,3-dicyano-4-phenyl-1-azabicyclo[2.1.1]hexane-2,3-dicarboxylate (trans-4b). Less polar fraction. Yield: 120 mg (34%). Colorless oil, isolated and purified chromatographically. IR (neat): 2987s, 2941m, 2245w (C≡N), 1767vs (C=O), 1750vs (C=O), 1501m, 1448m, 1392m, 1328m, 1261vs (O–C), 1243vs, 1095s, 1005s, 1020m, 1005m, 918m, 850m, 761vs, 725m, 700s. 1H-NMR (CDCl3): 1.33, 1.47 (2t, 2JH,H = 7.1 Hz, 2MeCH2); 3.52–3.59 (m, 1H); 3.71–3.74 (m, 1H); 3.87–3.90 (m, CH2O); 4.22–4.29 (m, 1H); 4.37–4.43 (m, 1H); 4.44–4.57 (m, CH2O); 7.22–7.27 (m, 2H, Ph); 7.34–7.39 (m, 3H, Ph). 13C-NMR (CDCl3): 13.8 (q, Me); 60.7 (s, Cq); 64.3, 64.7 (2t, 2CH2O); 64.8 (t, CH2); 65.8 (s, Cq); 66.1 (t, CH2); 68.6 (Cq); 114.4, 115.5 (2s, 2C≡N); 127.1, 128.7, 129.0 (3d, 5 arom CH); 131.2 (s, arom. Cq); 162.1, 163.5 (2s, 2C=O). CI-MS: 356 (14), 355 (22), 354 (100, [M+1]+). Anal. Calcd for C19H19N3O4: C, 64.58; H, 5.42; N, 11.89. Found: C, 64.47; H, 5.39; N, 11.73.
cis-Diethyl 2,3-dicyano-4-phenyl-1-azabicyclo[2.1.1]hexane-2,3-dicarboxylate (cis-4b). More polar fraction. Yield: 88 mg (25%). Colorless prisms, mp 143–144 °C (hexane/CH2Cl2). IR (KBr): 2986w, 2246w (C≡N), 1777s (C=O), 1740s (C=O), 1451w, 1279m, 1259vs (C=O), 1244vs (C=O), 1092s, 1055m, 1022w, 1007w, 921w, 851w, 706m. 1H-NMR (CDCl3): 1.11, 1.37 (2t, 2JH,H = 7.1 Hz, 2MeCH2); 3.55 (dd, 2JH,H = 8.5 Hz, 10.1 Hz, 1H); 3.78 (dd, 2JH,H = 5.6 Hz, 8.3 Hz, 2H); 4.04 (dd, 2JH,H = 8.4 Hz, 10.2 Hz, 1H); 4.20-4.34 and 4.35-4.49 (2m, 2CH2O); 7.06–7.15 (m, 2H, Ph); 7.35–7.44 (m, 3H, Ph). 13C-NMR (CDCl3): 13.7, 13.8 (2q, 2Me); 61.6, 71.5, 77.8 (3s, 3Cq); 62.7, 64.3 (2t, 2CH2); 64.1, 66.3 (2t, 2CH2O); 114.8, 115.5 (2s, 2C≡N); 126.2, 129.0, 129.4 (3d, 5 arom. CH); 130.9 (s, arom. Cq); 161.8, 164.9 (2s, 2C=O). CI-MS: 356 (8), 355 (22), 354 (100, [M+1]+). Anal. Calcd for C19H19N3O4: C, 64.58; H, 5.42; N, 11.89. Found: C, 64.29; H, 5.46; N, 11.90.
trans-Diisopropyl 2,3-dicyano-4-phenyl-1-azabicyclo[2.1.1]hexane-2,3-dicarboxylate (trans-4c). Less polar fraction. Yield: 107 mg (28%). Colorless prisms, mp 81–83 °C (hexane/CH2Cl2). IR (KBr): 2985m, 2245w (C≡N), 1763vs (C=O), 1746vs (C=O), 1466m, 1270vs (C=O), 1104s, 1054m, 909w. 1H-NMR (CDCl3): 1.24, 1.38, 1.45, 1.48 (4d, 2JH,H = 6.3 Hz, 2Me2CH); 3.60–3.52 (m, 1H); 3.72–3.69 (d, JH,H = 8.4 Hz, 1H); 3.84–3.91 (m, 2H); 5.10–5.19, 5.27–5.35 (2m, 2Me2CHO); 7.23–7.25 (m, 2H, Ph); 7.34–7.38 (m, 3H, Ph). 13C-NMR (CDCl3): 21.5, 21.6 (2q, 2Me2CH); 60.6 (s, Cq); 64.8, 66.0 (2t, 2CH2); 68.4 (s, 2Cq); 72.9, 73.6 (2d, 2Me2CHO); 114.6, 115.6 (2s, 2C≡N); 127.2, 128.7, 128.9 (3d, 5 arom. CH); 131.3 (s, arom. Cq); 161.6, 163.0 (2s, 2C=O). CI-MS: 384 (8), 383 (25), 382 (100, [M+1]+). Anal. Calcd for C21H23N3O4: C, 66.13; H, 6.07; N, 11.01. Found: C, 66.14; H, 6.05; N, 11.05.
cis-Diisopropyl 2,3-dicyano-4-phenyl-1-azabicyclo[2.1.1]hexane-2,3-dicarboxylate (cis-4c). More polar fraction. Yield: 73 mg (19%). Colorless prisms, mp 191–193 °C (hexane/CH2Cl2). IR (KBr): 2987w, 2248w (C≡N), 1767vs (C=O), 1731m (C=O), 1464w, 1378w, 1263vs (C=O), 1101s, 1056w, 927w, 701w. 1H-NMR (CDCl3): 0.86, 1.24, 1.29, 1.38 (4d, 2JH,H = 6.3 Hz, 2Me2CH); 3.52 (dd, 2JH,H = 8.5 Hz, 10.1 Hz, 1H); 3.74 (dd, 2JH,H = 5.6 Hz, 8.3 Hz, 2H); 4.04 (dd, 2JH,H = 8.4 Hz, 10.2 Hz, 1H); 4.88–4.96, 5.14–5.23 (2m, 2Me2CHO); 7.10–7.15 (m, 3H, Ph); 7.32–7.40 (m, 2H, Ph). 13C-NMR (CDCl3): 21.4, 21.3, 21.2 (3q, 2Me2CH); 62.4, 66.2 (2t, 2CH2); 61.6, 71.6, 77.6 (3s, 3Cq); 72.6, 72.9 (2d, 2Me2CHO); 115.1, 115.6 (2s, 2C≡N); 126.2, 128.9, 129.3 (3d, 5 arom. CH); 131.0 (s, arom. Cq); 161.4, 164.2 (2s, 2C=O). CI-MS: 384 (8), 383 (24), 382 (100, [M+1)+). Anal. Calcd for C21H23N3O4: C, 66.13; H, 6.07; N, 11.01. Found: C, 66.14; H, 6.05; N, 11.05.
Reactions of azabicyclobutanes 1b-d with (E)-5a in methanolic solutions. The solution containing 1 mmol of the corresponding 1 in 1 mL of MeOH was added at rt in small portions to a magnetically stirred solution containing 194 mg (1 mmol) of (E)-5a dissolved in 1 mL of MeOH. After 15 min, the solvent was evaporated in vacuo and the crude product obtained thereby was analyzed by 1H-NMR spectroscopy. A preliminary purification was achieved by chromatography on a short column filled with SiO2, and CHCl3 was used as the eluent. The isolated fraction was additionally purified on preparative plates (CH2Cl2 as the eluent), and only in the case of 7c, an analytically pure sample was obtained after crystallization. Reported yields were calculated for the products obtained after preparative LC (PLC).
Dimethyl (E)-2-(3’-ethyl-3’-methoxyazetidin-1-yl)-3-cyanobutanedioate (7a). Yield: 216 mg (77%). Colorless, thick oil isolated after chromatography (PLC, SiO2, CH2Cl2). IR (neat): 2954m, 2205s, (C≡N), 1748vs (C=O), 1705vs, (C=O), 1569vs (C=C), 1456s, 1436s, 1306s, 1250s, 1193s, 1177s, 1331s (br), 1070s, 1020w, 769m. 1H-NMR (CDCl3): 0.91 (t, 2JH,H = 7.3 Hz, MeCH2); 1.84 (q, 2JH,H = 7.3 Hz, MeCH2); 3.21, 3.73, 3.91 (3s, 3MeO); 3.94, 4.09 (AB, J = 10.0 Hz, CH2-azetidine); 4.08, 4.42 (AB, J = 11.5 Hz, CH2-azetidine). 13C-NMR (CDCl3): 6.9 (q, MeCH2); 26.6 (t, MeCH2); 50.7, 52.1, 53.6 (3q, 3MeO); 62.4, 62.8 (2t, 2CH2-azetidine); 71.0 (s, Cq); 75.9 (s, Cq-azetidine); 117.0 (s, C≡N); 158.7 (s, Cq); 161.9, 165.1 (2s, 2C=O). ESI-MS: 305 (100, [M+Na]+). Anal. Calcd for C13H18N2O5: C, 55.31; H, 6.43; N, 9.92. Found: C, 55.26; H, 6.21; N, 9.87.
Dimethyl (E)-2-(3’-phenyl-3’-methoxyazetidin-1-yl)-3-cyanobutanedioate (7b). Yield: 180 mg (55%). Colorless, thick oil isolated after chromatography (PLC, SiO2, CHCl3). IR (neat): 2953s, 2206s (C≡N), 1747vs (C=O), 1709vs (C=O), 1569vs (C=C), 1450s, 1436s, 1306s, 1250vs, 1195s, 1138vs, 1065m, 1637m, 767s, 702s. 1H-NMR (CDCl3): 3.05, 3.74, 3.92 (3s, 3MeO); 4.42 (br.s, CH2); 4.89, 5.00 (AB, J = 11.8 Hz, CH2); 7.31–7.47 (m, 5H, Ph). 13C-NMR (CDCl3): 51.9, 52.1, 53.7 (3q, 3MeO); 63.5, 63.8 (2t, 2CH2-azetidine); 70.8 (s, Cq); 77.3 (s, Cq-azetidine); 116.7 (s, C≡N); 126.1, 129.0, 129.1 (3d, 5 arom. CH); 137.4 (s, arom. Cq); 158.5 (s, Cq); 161.8, 165.0 (2s, 2C=O). ESI-MS: 353 (100, [M+Na]+). Anal. Calcd for C17H18N2O5: C, 61.81; H, 5.49; N, 8.48. Found: C, 61.67; H, 5.33; N, 8.78.
Dimethyl (E)-2-(3’-phenyl-3’-methoxy-2,2-dimethylazetidin-1-yl)-3-cyanobutanedioate (7c). Yield: 190 mg (53%). Colorless prisms, mp 107–109 °C (MeOH). IR (KBr): 2953s, 2205m (C≡N), 1751vs (C=O), 1702s (C=O), 1549vs (C=C), 1440m (br), 1302m, 1265s, 1246s, 1193m, 1146s, 1133s, 769m, 703m. 1H-NMR (CDCl3): 0.98, 1.56 (2s, 2Me); 3.04, 3.74, 3.88 (3s, 3MeO); 4.78, 5.21 (AB, J = 12.3 Hz, CH2); 7.26–7.46 (m, 5H, Ph). 13C-NMR (CDCl3): 21.4, 25.6 (2q, 2Me); 52.0, 53.3 (2q, 3MeO); 56.8 (t, CH2); 70.4, 81.2, 83.0 (3s, 3Cq); 117.9 (s, C≡N); 127.1, 128.8, 128.9 (3d, 5 arom. CH); 135.4 (s, arom. Cq); 157.1 (s, Cq); 162.6, 165.3 (2s, 2C=O). ESI-MS: 381 (100, [M+Na]+). Anal. Calcd for C19H22N2O5: C, 63.67; H, 6.19; N, 7.82. Found: C, 63.47; H, 5.93; N, 7.76.
X-Ray Crystal-Structure Determination of cis-4c (Figure 1).19 All measurements were made on a Nonius KappaCCD diffractometer20 using graphite-monochromated MoKα radiation (λ = 0.71073 Å) and an Oxford Cryosystems Cryostream 700 cooler. Data reduction was performed with HKL Denzo and Scalepack.21 The intensities were corrected for Lorentz and polarization effects, but not for absorption. Equivalent reflections were merged. The data collection and refinement parameters are given below, and a view of the molecule is shown in Figure 1. The structure was solved by direct methods using SIR92,22 which revealed the positions of all non-hydrogen atoms. The non-hydrogen atoms were refined anisotropically. All of the hydrogen-atoms were placed in geometrically calculated positions and refined using a riding model where each hydrogen-atom was assigned a fixed isotropic displacement parameter with a value equal to 1.2Ueq of its parent C-atom (1.5Ueq for the methyl groups). The refinement of the structure was carried out on F2 using full-matrix least-squares procedures, which minimized the function Σw(Fo2–Fc2)2. A correction for secondary extinction was applied. Five reflections, whose intensities were considered to be extreme outliers, were omitted from the final refinement. Neutral atom scattering factors for non-hydrogen atoms were taken from ref.23, and the scattering factors for hydrogen-atoms were taken from ref.24 Anomalous dispersion effects were included in Fc;25 the values for ƒ’ and ƒ” were those of ref.26 The values of the mass attenuation coefficients are those of ref.27 All calculations were performed using the SHELXL97 program.28 Crystal data for cis-4c: Crystallized from hexane/CH2Cl2, C21H23N3O4, M = 381.43, colorless, prism, crystal dimensions 0.23 × 0.25 × 0.30 mm, triclinic, space group P, Z = 2, reflections for cell determination 5715, a = 10.1892(3) Å, b = 10.5551(2) Å, c = 10.7555(3) Å, α = 61.812(1)°, β = 77.635(1)°, γ = 84.420(2), V = 995.88(5) Å3, DX = 1.272 g⋅cm–3, (MoK) = 0.0892 mm–1, T = 160(1) K, and scans, 2θmax = 60°, total reflections measured 26597, symmetry independent reflections 5827, reflections with I > 2σ(I) 4451, reflections used in refinement 5822, parameters refined 258, R (on F; I > 2σ(I) reflections) = 0.0465, wR(F2) (all reflections) = 0.1234 (w = (σ2(Fo2) + (0.0517P)2 + 0.2317P)–1, where P = (Fo2+2Fc2)/3), goodness of fit 1.042, secondary extinction coefficient 0.029(5), final Δmax/σ 0.001, Δρ (max; min) = 0.29; –0.23 e Å–3.
ACKNOWLEDGEMENTS
We thank the analytical services of our institutes for analyses and spectra. G. M. thanks the Rector of the University of Łódź for financial support (Grant # 505/0712) and H. H. acknowledges financial support by F. Hoffmann-La Roche AG, Basel.
Dedicated to Professor Emeritus Keiichiro Fukumoto on the occasion of his 75th birthday
References
1. R. Bartnik and A. P. Marchand, Synlett, 1997, 1029. CrossRef
2. W. Funke W., Chem. Ber., 1969, 102, 3148. CrossRef
3. a) K. Hayashi, S. Hiki, T. Kumagai, and Y. Nagao, Heterocycles, 2002, 56, 433; CrossRef b) K. Hayashi, Y. Ikee, S. Goto, M. Shiro, and Y. Nagao, Chem. Pharm. Bull., 2004, 52, 89. CrossRef
4. A. G. Hortmann and D. A. Robertson, J. Am. Chem. Soc., 1972, 94, 2758. CrossRef
5. V. Aggarwal and J. Richardson, Science of Synthesis, 2004, 72, 21.
6. a) G. Alvernhe, A. Laurent, K. Toukami, R. Bartnik, and G. Mloston, J. Fluorine Chem., 1985, 29, 363; CrossRef b) G. Mloston and M. Celeda, Helv. Chim. Acta, 2005, 88, 1658. CrossRef
7. R. Bartnik, S. Lesniak, G. Mloston, and J. Romanski, Pol. J. Chem., 1994, 68, 1347.
8. M. Woznicka, K. Urbaniak, G. Mloston, and H. Heimgartner, Heterocycles, 2006, 69, 351. CrossRef
9. G. Mloston, M. Woznicka, J. Drabowicz, A. Linden, and H. Heimgartner, Helv. Chim. Acta, 2008, 91, in press.
10. Y. Ikee, K. Hoshimoto, M. Nakashima, K. Hayashi, S. Sano, M. Shiro, and Y. Nagao, Bioorg. Med. Chem. Lett., 2007, 17, 942. CrossRef
11. G. Mloston, H. Heimgartner, Helv. Chim. Acta, 2006, 89, 442. CrossRef
12. C. K. Johnson, ORTEP II, Report ORNL-5138, Oak Ridge National Laboratory, Oak Ridge, Tennessee, 1976.
13. C. Dell’Erba, M. Novi, G. Petrillo, and C. Tavani, Tetrahedron, 1995, 51, 3905. CrossRef
14. a) Y. Yamada and H. Yasuda, J. Heterocycl. Chem., 1998, 35, 1389; CrossRef b) Y. Yamada, H. Yasuda, and M. Kasai, Heterocycles, 1999, 51, 2453. CrossRef
15. a) Y. Yamada, H. Yasuda, and A. Takayama, Heterocycles, 1998, 48, 1185; CrossRef b) Y. Yamada, H. Yasuda, and K. Yoshizawa, Heterocycles, 1998, 48, 2095. CrossRef
16. G. Mloston, M. Celeda, A. Linden, and H. Heimgartner, in preparation.
17. a) G. Mloston, R. Huisgen, H. Huber, and D. S. Stephenson, J. Heterocyclic Chem., 1999, 36, 959; CrossRef b) R. Huisgen, G. Mloston, H. Giera, and E. Langhals, Tetrahedron, 2002, 58, 507. CrossRef
18. C. J. Ireland and J. S. Pizey, J. Chem. Soc., Chem. Commun., 1972, 4. CrossRef
19. CCDC-692246 contains the supplementary crystallographic data for this paper. These data can be obtained free of charge from the Cambridge Crystallographic Data Centre via www.ccdc.cam.ac.uk/data_request/cif.
20. R. Hooft, KappaCCD Collect Software, Nonius BV, Delft, The Netherlands, 1999.
21. Z. Otwinowski and W. Minor, in ‘Methods in Enzymology’, Vol. 276, ‘Macromolecular Crystallography’, Part A, ed. by C. W. Carter Jr. and R. M. Sweet, Academic Press: New York 1997, pp. 307-326.
22. A. Altomare, G. Cascarano, C. Giacovazzo, A. Guagliardi, M. C. Burla, G. Polidori, and M. Camalli, SIR92, J. Appl. Crystallogr. 1994, 27, 435. CrossRef
23. E. N. Maslen, A. G. Fox, and M. A. O‘Keefe, in ‘International Tables for Crystallography’, ed. by A. J. C. Wilson, Kluwer Academic Publishers, Dordrecht, 1992, Vol. C, Table 6.1.1.1, p. 477.
24. R. F. Stewart, E. R. Davidson, and W. T. Simpson, J. Chem. Phys. 1965, 42, 3175. CrossRef
25. J. A. Ibers and W. C. Hamilton, Acta Crystallogr. 1964, 17, 781. CrossRef
26. D. C. Creagh, and W. J. McAuley, in ‘International Tables for Crystallography’, ed. by A. J. Wilson, Kluwer Academic Publishers, Dordrecht, 1992, Vol. C, Table 4.2.6.8, pp. 219-222.
27. D. C. Creagh, and J. H. Hubbell, in ‘International Tables for Crystallography’, ed. by A. J. C. Wilson, Kluwer Academic Publishers, Dordrecht, 1992, Vol. C, Table 4.2.4.3, pp. 200-206.
28. G. M. Sheldrick, SHELXL97, Program for the Refinement of Crystal Structures, University of Göttingen, Germany.