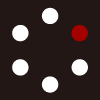
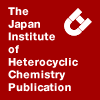
HETEROCYCLES
An International Journal for Reviews and Communications in Heterocyclic ChemistryWeb Edition ISSN: 1881-0942
Published online by The Japan Institute of Heterocyclic Chemistry
e-Journal
Full Text HTML
Received, 15th July, 2008, Accepted, 18th September, 2008, Published online, 22nd September, 2008.
DOI: 10.3987/COM-08-S(F)45
■ Tandem Metathesis Reactions Cascade Ring-Opening Metathesis (ROM)-Ring-Closing Metathesis (RCM)-Cross Metathesis Dimerization (CMD) in 7-Oxabicyclo[2.2.1]hept-5-one (7-Oxanorbornene) Derivatives
Ana Aljarilla and Joaquín Plumet*
Department of Organic Chemistry, Faculty of Chemistry, University of Complutense, 28040-Madrid, Spain
Abstract
The domino metathesis sequence ROM-RCM-CMD achieved on conveniently substituted 7-oxabicyclo[2.2.1]hept-5-ene (7-oxanorbornene) derivatives is reported. Experimental conditions in order to carry out this tandem metathetical sequence in a chemoselective fashion is also established.The use of olefin metathesis1 in domino or cascade reactions2 is a well established synthetic methodology which may be considered within the more general concept of “Concurrent Tandem Catalysis (CTC)”.3 These reactions sequences may consist in the combination of different modes of olefin metathesis4 or in the combination of a metathesis step followed by a non-metathesis reaction catalyzed by the same catalitically active specie.5 In the case of strained cycloolefins such as norbornene derivatives6 and considering only metathesis domino reactions the sequences ring-opening metathesis (ROM)-cross metathesis (CM)7, ROM-ring-closing metathesis (RCM)8 (Scheme 1)9 and ring-opening metathesis dimerization (ROMD)-CM10 (Scheme 2) have been previously reported. Many synthetic applications of these procedures were also published.11
In a previous report12 we have accounted for the sequence ROM-CM-RCM reactions in 7-oxanorbornene derivatives 1a-c using pre-catalyst 5 and in presence of allyl acetate to give, in good yields and after catalytic hydrogenation, substituted 2,6-dioxabicyclo[4.3.0]nonane derivatives 2a-c (Scheme 3). In the case of compounds 1b and 1c bicyclic derivatives 3b and 3c were also isolated in minor amounts whereas starting from 1a dimer 4a was also obtained in 10% isolated yield as 1:1 mixture E-Z diastereomers. Obviously this product arises from the non-isolated intermediate 3a via CM dimerization (CMD)13 reaction. On the other hand the presence of compound 4a in the reaction mixture makes evident that the sequence ROM-RCM-CMD is operative in this case. This sequence has, to the best of our knowledge, not optimized so far.14
Considering that the 2,6-dioxabicyclo[4.3.0]nonane skeleton appears widely distributed in nature15 and that the dimeric derivatives such as 4a have, to the best of our knowledge, never been synthesized being also potentially useful derivatives of the parent compound, we decided to explore the search for experimental conditions in order to obtain chemoselectively bicyclic compounds 3 or dimeric derivatives 4 starting from a common oxanorbornene precursor and using tandem metathesis reaction sequences. For this purpose we reasoned that the modification of experimental conditions (in particular reaction time) and nature and concentration of the precatalyst can direct chemoselectively the reaction toward the monomeric or the dimeric bicyclic compound.
In a preliminary experiment we have observed (1H-NMR) that compound 1a in absence of any external alkene (including ethylene) and in the presence of precatalyst 5 (5% mol) was transformed after 30 min. in compound 3a. Prolongation of the reaction time resulted in the evolution of 3a to other compound showing 1H-NMR signals assignable to 4a. After this first experiment we decided to carry out the appropriate synthetic study using compounds 1a and 1d as starting materials. The results are summarized in Table 1.
From the inspection of Table 1 could be deduced that the chemoselective synthesis of 4a may easily be achieved using Grubb’s precatalyst 5 by prolongation of the reaction time regarding the synthesis of 3a. No other precatalysts nor reaction conditions were tested. In the case of compounds 6 and 7 monomeric bicyclic derivative 6 was obtained in excellent yields using precatalysts 8 and 9 under different experimental conditions whereas dimeric derivative 7 was only obtained in the presence of precatalyst 8 in 10% mol. and overnight (approximate 12h). In contrast with 4a the synthesis of 7 was only possible by modification of both precatalyst concentration and reaction time. On the other hand it should be pointed out that, under the experimental conditions described in Table 1, entry 5, the reaction took place with 100% conversion of the starting material 1d. Nevertheless compound 7 was only isolated in 43% yield exclusively as Z-diastereomer (63% overall yield in pure, isolated products). The E-diastereomer of 7 did not obtain from the crude reaction mixture.
In summary, in this report we have described a new metathesis tandem sequence ROM-RCM-CMD starting from conveniently substituted 7-oxanorbornene derivatives. The experimental conditions in order to carry out ROM-RCM- or ROM-RCM-CMD cascades in a chemoselective fashion have also been established.
EXPERIMENTAL
General Methods
All reactions were carried out under an argon atmosphere employing techniques in handling materials. All solvents were reagents grade. Dichloromethane was freshly distilled from calcium hydride. All other reagents and solvents were used as supplied. Flash chromatography was performed on silica gel columns (Keselgel 60, 230-400 mesh). Yields refer to chromatographically and spectroscopically pure compounds. Melting points were determined on a Gallenkamp apparatus and are uncorrected. 1HNMR and 13CNMR spectra were recorded on a Bruker AM-300 and Bruker AM-500 at room temperature in CDCl3 as solvent. Coupling constants are given in Hz and chemical shift are expressed in δ values (ppm). IR spectra were recorded on a Perkin-Elmer 781 apparatus in solution of dichloromethane (compound 3a, 4a, 6 and 7). Elemental analyses were carried out Perkin Elmer 2400 CHN apparatus at the Complutense University, Faculty of Pharmacy, Madrid.
Experimental procedure for metathesis reactions of compounds 1a and 1d: to a solution of 1.0 eq. oxanorbornene derivative in CH2Cl2 (22 mL/mmol) under Ar, catalysts 5, 7 or 8 was added in CH2Cl2 (55 mL/mmol). After the appropriate reaction time the solvent was removed at atmospheric pressure in the case of 1a or at vacuo for 1d. The reaction crude was purified by column chromatography (SiO2, hexane-EtOAc 4:1. Compounds 6 and 7 were also separated using a mixture pentane-Et2O 7:3).
Spectroscopic and analytical data for compounds 3a, 4a, 6 and 7.
Compound 3a.
Colourless oil. IR: νmax (CH2Cl2) 3041, 2939, 2875, 1155, 1089. Anal. Calcd for C9H12O2 (152.08): C, 71.03, H, 7.95. Found: C, 71.11, H, 7.80. 1H-NMR (CDCl3, 500 MHz): δ 6.11 (dd, 1H, J= 10.3, 3.45 Hz, H7), 6.07 (m, 1H, H6), 6.00 (ddd, 1H, J = 17.10, 10.30, 7.40 Hz, -CH = CH2), 5.26 (dm, 1H, J = 17.10 Hz, -CH = CH2trans), 5.13 (dm, 1H, J = 10.30 Hz, -CH = CH2cis), 4.33 (q, 1H, J = 7.40 Hz, H2), 4.24 (dd, 1H, J = 16.60, 3.45 Hz, H5), 4.09 (m, 1H, H3a), 4.06 (dq, 1H, J = 16.80, 3.45 Hz, H5’), 3.84 (q, 1H, J = 3.40 Hz, H7a), 2.51 (ddd, 1H, J = 13.90, 8.00, 6.90 Hz, H3), 1.83 (ddd, 1H, J = 13.90,7.40, 1.88 Hz, H3’), ppm. 13C-NMR (CDCl3, 75 MHz): δ 138.87 (-CH = CH2), 131.61 (C7), 122.71 (C6), 116.64 (-CH = CH2), 79.93 (C2), 76.39 (C3a), 73.42 (C7a), 64.52 (C5), 39.89 (C3) ppm.
Compound 4a.
Colourless oil. IR: νmax (CH2Cl2) 3040, 2939, 2876, 1087, 1049. Anal. Calcd for C16H20O4 (276.14): C, 69.54, H, 7.30. Found: C, 69.69, H, 7.51. 1H-NMR (CDCl3, 300 MHz): δ 6.13- 6.00 (m, 4H, H6, H7), 5.86 (dd, 1H, J = 4.00, 2.00 Hz, -CHcis = CH), 5.83 (dd, 1H, J = 5.00, 2.50 Hz, -CHtrans = CH), 4.32 (m, 2H, H2), 4.18 (dm, 2H, J = 16.20 Hz, H5), 4.05 (m, 2H, H3a), 4.02 (dm, 2H, J = 16.20 Hz, H5’), 3.80 (q, 2H, J = 3.20 Hz, H7a), 2.49 (dt, 2H, J = 14.00, 7.40 Hz, H3), 1.81 (m, 2H, H3’) ppm. 13C-NMR (CDCl3, 75 MHz): δ 132.05 (-CHtrans = CH), 131.05 (-CHcis = CH), 130.03 and 129.93 (C7), 121.02 and 120.95 (C6), 77.36 and 76.97 (C2), 74.76 and 74.65 (C3a), 71.73 and 71.61 (C7a), 62.86 and 62.81 (C5), 38.31 and 38.13 (C3) ppm.
Compound 6.
Colourless oil. IR: νmax (CH2Cl2) 3080, 3024, 2924, 2928, 1725, 1253, 1057. Anal. Calcd for C9H10O3 (166.06): C, 65.05, H, 6.07. Found: C, 65.20, H, 6.18. 1H-NMR (CDCl3, 300 MHz): δ 6.91 (dd, 1H, J = 9.90, 5.00 Hz, H7), 6.18 (d, 1H, J = 5.00 Hz, H6), 5.90 (ddd, 1H, J = 17.10, 10.30, 7.40 Hz, -CH = CH2), 5.29 (dm, 1H, J = 17.10 Hz, -CH = CH2trans), 5.18 (dm, 1H, J = 10.30 Hz, -CH = CH2cis), 5.07 (m, 1H, H3a), 4.47 (q, 1H, J = 7.40 Hz, H2), 4.27 (t, 1H, J = 5.00 Hz, H7a), 2.66 (dt, 1H, J = 14.20, 7.40, 6.60 Hz, H3), 2.18 (ddd, 1H, J = 14.20, 6.60, 3.00 Hz, H3’) ppm. 13C-NMR (CDCl3, 75 MHz): δ 162.00 (CO), 140.63 (C7), 137.73 (-CH = CH2), 123.93 (C6), 117.72 (-CH = CH2), 80.69 (C3a), 79.99 (C2), 70.40 (C7a), 40.20 (C3) ppm.
Compound 7.
Colourless oil. IR: νmax (CH2Cl2) 2923, 2853, 1724, 1256, 1050. Anal. Calcd for C16H16O6 (304.09): C, 63.15, H, 5.30. Found: C, 63.26, H, 5.50. 1H-NMR (CDCl3, 300 MHz): δ 6.83 (dt, 2H, J = 9.90, 4.00 Hz, H7), 6.11 (dd, 2H, J = 9.90, 6.90 Hz, H6), 5.70 (ddd, 2H, J = 4.38, 2.10, 0.8 Hz, -CH = CH-), 4.98 (m, 2H, H3a), 4.42 (m, 2H, H2), 4.17 (td, 2H, J = 4.80, 2.10 Hz, H7a), 2.55 (m, 2H, H3), 2.10 (m, 2H, H3’) ppm. δ 160.88 (CO), 140.60 and 140.412 (C7), 132.93 and 132.58 (-CH = CH), 124.15 and 123.95 (C6), 80.79 and 80.69 (C3a), 78.95 and 78.72 (C2), 70.28 and 70.16 (C7a), 40.23 (C3) ppm.
ACKNOWLEDGEMENTS
Ministério de Educación y Ciencia, Spain (Project CTQ-2006-15279-03-01) is gratefully thanked for financial support. One of us (A.A.) thanks Ministerio de Educación y Ciencia for a Grant.
References
1. For an authoritative book on metathesis reactions see: a) “Handbook of Metathesis”, Three volume set.n ed. by R. H. Grubbs, Wiley-VCH, Weinheim, 2003. See in particular Vol. 2: “Applications in Organic Synthesis”; See also: b) R. H. Grubbs and T. M. Trnka, in “Ruthenium-Catalyzed Olefin Metathesis”, Ruthenium in Organic Synthesis, ed. by S. I. Murahasi, Chp. 6, pp. 153-179, Wiley-VCH, Weinheim, 2004; For a comprehensive general review see: c) A. Fürstner, Angew. Chem. Int. Ed., 2000, 39, 3013. ; CrossRef For a concise overview see: d) A. H. Hoveyda and A. R. Zhugralin, Nature, 2007, 450, 243. CrossRef
2. For a treatise on domino reactions see: a) L. F. Tietze, G. Brasche, and K. Gericke, in “Domino Reactions in Organic Synthesis”, Wiley-VCH, Weinheim, 2006; CrossRef For a general review see: b) L. F. Tietze, Chem. Rev., 1996, 106, 115. CrossRef
3. Review: J. C. Wasilke, S. J. Obrey, R. Tom Baker, and G. C. Bazan, Chem. Rev., 2005, 105, 1001. CrossRef
4. See, for instance: a) S. Randl and S. Blechert, in “Tandem Ring-Closing Metathesis”. Ref. 1a, Vol. 2, Chp. 4, pp. 151-175; b) T. O. Schrader and M. L. Snapper, in “Ring-Opening-Cross Metathesis”. Ref. 1a, Vol. 2, Chp. 6, pp. 205-237; See also: c) S. Y. Han and S. Chang, in “General Ring-Closing Metathesis”. Ref. 1a, Vol. 2, Chp. 2. See, in particular, pp. 88-92; For other selected accounts see: d) S. J. Connon and S. Blechert, Topics in Organometallic Chem., 2004, 11, 93; CrossRef e) J. Mulzer and E. Oehler, Topics in Organometallic Chem., 2004, 13, 269; CrossRef f) M. Mori, J. Mol. Catalysis (A), 2004, 213, 73; CrossRef g) S. G. Aitken and A. D. Abell, Aust. J. Chem., 2005, 58, 3; CrossRef h) B. Schmid and J. Hermanns, Curr. Org. Chem., 2006, 10, 1363. CrossRef
5. See reference 3, p. 1011-1013 and: a) B. Schmidt, Pure Appl. Chem., 2006, 78, 469; CrossRef b) V. Dragutan and I. Dragutan, J. Organometal. Chem., 2006, 691, 5129. CrossRef
6. For a review on sequential metathesis reactions in oxa- and azanorbornene derivatives, see: a) O. Arjona, A. G. Csákÿ, and J. Plumet, Eur. J. Org. Chem., 2003, 611; CrossRef See also b) R. Medel and J. Plumet, Targets in Heterocyclic Systems, 2004, 8, 162.
7. After the first reports concerning the ROM-CM of strained olefins (see: a) M.F. Schneider and S. Blechert, Angew. Chem., Int. Ed. Engl., 1996, 35, 411; CrossRef b) M. F. Schneider, N. Lucas, J. Velder, and S. Blechert, Angew. Chem., Int. Ed. Engl., 1997, 36, 257; CrossRef c) O. Arjona, A. G. Csákÿ, M. C. Murcia, and J. Plumet, J. Org. Chem., 1999, 64, 9739, a considerable amount of literature on this topic have been appeared. For a general account see reference 4b. In this context the concept of Asymmetric Ring-Opening Metathesis-Cross Metathesis (AROM-CM) in norbornene derivatives and other strained bicyclic alkenes is currently considered as a powerful method for the construction of complex organic molecules in enantiomerically pure form; CrossRef See: d) A. H. Hoveyda, in “Catalytic Asymmetric Olefin Metathesis”. Ref. 1a, Vol. 2, Chp. 2, pp. 128-150; e) A. H. Hoveyda, in “Organic Synthesis Highlights”, ed. by H. G. Schmalz and T. Wirth, Wiley-VCH, Weinheim, 2003, p. 210; CrossRef See also: f) A. H. Hoveyda and R. R. Schrock, Chem. Eur. J., 2001, 7, 945. CrossRef
8. See referente 4a. For some further recent reports on the application of this methodology to the construction of polycyclic and polyheterocyclic ring systems from norbornene derivatives, see: a) M. Mori, H. Wakamatsu, Y. Sato, and R. Fujita, J. Mol. Catal. (A), 2006, 254, 64; CrossRef b) A. Aljarilla, M. C. Murcia, and J. Plumet, Synlett, 2006, 831; c) S. Maechling, S. E. Norman, J. E. McKendrick, S. Basra, K. Kopper, and S. Blechert, Tetrahedron, 2006, 47, 189; CrossRef d) Z. Liu and J. D. Rainier, Org. Lett., 2006, 8, 459; CrossRef e) J. Carreras, A. Avenoza, J. H. Busto, and J. M. Peregrina, Org. Lett., 2007, 9, 1235; CrossRef f) G. Calvet, N. Blanchard, and C. Kouklowsky, Org. Lett., 2007, 9, 1485; CrossRef g) C. K. Malik and S. Ghosh, Org. Lett., 2007, 9, 2537; CrossRef h) E. Groaz, D. Banti, and M. North, Adv. Synth. Catal., 2007, 349, 142; CrossRef i) E. Groaz, D. Banti, and M. North, Eur. J. Org. Chem., 2007, 3927; j) S. Maity and S. Ghosh, Tetrahedron Lett. 2008, 49, 1133; CrossRef k) E. Groaz, D. Banti, and M. North, Tetrahedron, 2008, 64, 2470; CrossRef l) M. Ikoma, M. Oikawa, and M. Sasaki, Tetrahedron, 2008, 64, 2740; CrossRef m) A. Aljarilla and J. Plumet, Heterocycles, 2008, 76, 827. CrossRef
9. Convenient appendage on position Z (Z = CH2, N) could induce the formation of the related tricyclic systems. See, for instance, references 8i and 8m. This possibility has been omitted in Scheme 1 for simplicity.
10. Only the specific case showed in Scheme 2 had, to the best of our knowledge, been reported for this tandem reaction. See O. Arjona, A. G. Csákÿ, M. C. Murcia, J. Plumet, and M. B. Mula, J. Organometal. Chem., 2001, 627, 105. CrossRef
11. For selected cases see reference 4. Other specific applications may be found in: a) J. A. Love, in “Olefin Methatesis Strategies in the Synthesis of Biologically Relevant Molecules”. Ref. 1a, Vol. 2, Chp. 9, pp. 296-352 with special contributions of K. C. Nicolau, S. A. Snyder (“The Olefin Metathesis Reactions in Complex Molecule Construction”, pp. 323-337), and S. F. Martin (“Applications of RCM in Alkaloid Synthesis”, pp. 338-352); For more recent accounts, see: b) S. Kotha and K. Lahiri, Synlett, 2007, 2767; CrossRef c) N. Holub and S. Blechert, Chemistry, Asian J., 2007, 2, 1064. CrossRef
12. O. Arjona, G. Csákÿ, M. C. Murcia, and J. Plumet, Tetrahedron Lett., 2000, 41, 9777. CrossRef
13. CMD reactions have been employed in the synthesis of macrocyclic compouds such as cylindrophanes A and F (see: a. A. B. Smith III, S. A. Kozmin, C. M. Adams, and D. V. Paone, J. Am. Chem. Soc., 2000, 122, 4984 and references therein), [3]-catenane ; CrossRef (see b. H. Iwamoto, K. Itoh, H. Nagamiya, and Y. Fukazawa, Tetrahedron Lett., 2003, 44, 5773) and paracyclophanes; CrossRef (see C. J. Tae and Y. K. Yang, Org. Lett., 2003, 5, 741); CrossRef For a review on CM reactions including synthetic applications see: d) S. J. Connon and S. Blechert, Angew. Chem. Int. Ed., 2003, 42, 1900. CrossRef
14. Analogous dimeric material was detected (LC-MS analysis) in a related process. See reference 8 l p. 2743.
15. For recent review, see: J. D. More, Org. Prep. Proc. Int., 2007, 39, 107.