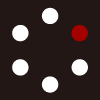
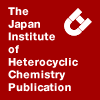
HETEROCYCLES
An International Journal for Reviews and Communications in Heterocyclic ChemistryWeb Edition ISSN: 1881-0942
Published online by The Japan Institute of Heterocyclic Chemistry
e-Journal
Full Text HTML
Received, 17th July, 2008, Accepted, 22nd August, 2008, Published online, 25th August, 2008.
DOI: 10.3987/COM-08-11496
■ Synthesis and Sulfuration of 2’-Adamantylidene-9-benzonorbornenylidenee
Yoshiaki Sugihara,* Ayumi Kobiki, and Juzo Nakayama*
Department of Chemistry, Faculty of Science, Saitama University, Urawa, Saitama 338-8570, Japan
Abstract
A novel alkene, 2’-adamantylidene-9-benzonorbornene 6, was synthesized from 2-adamantanone 7 in three steps resulting in a good overall yield. The alkene 6 reacted with elemental sulfur in refluxing o-Cl2C6H4 to give syn-thiirane 14, anti-thiirane 15, and 6. Almost the same ratio of the products was obtained when 14 or 15 was reacted with and without elemental sulfur under the same conditions. Sulfuration with Ph3CSCl in CH2Cl2 at -78 °C afforded 14, and that at room temperature furnished a 3:1 mixture of 14 and 15. In contrast, although the sulfuration with Ph3CSCl in CH2Cl2 at room temperature afforded 14 quantitatively, that at -78 °C gave 14 and 15 in 12 and 3% yields, respectively. Sulfuration by 1 molar equiv. of S2Cl2 or SCl2 afforded 14 and vic-dichloride 28.INTRODUCTION
Considerable attention has been paid to the study of the sulfuration of alkenes because sulfuration is important as a model reaction of the vulcanization of rubber, and is it easy to introduce sulfur atoms into their C=C bonds.1 One of the goals of its study would be the control of the number of sulfur atoms introduced. The number was found to noticeably depend on the structure of the alkenes when elemental sulfur was used as the sulfurating agent.2—5 Although, in most cases, sulfuration gives a complex mixture containing polymeric compounds, cyclic oligosulfanes are obtained as the principal component in some cases. Thus, the sulfuration of norbornene and dibenzobarrelene by elemental sulfur gave a mixture of the corresponding 1,2,3-trithiolane and 1,2,3,4,5-pentathiepane,2,3 and that of acenaphtho[1,2-a]- acenaphtylene afforded the corresponding pantathiepane as a sole product.4 On the other hand, sterically congested 2,2'-biadamantylidene reacted with elemental sulfur to furnish the corresponding thiirane exclusively.3 Sulfurations of anti- and syn-9,9'-bibenzonorbornenylidenes, 1 and 2,6 by elemental sulfur afforded the corresponding thiiranes, 3 and 4, respectively, with retention of the original stereochemistry, whereas those by S2Cl2 afforded a mixture of 3, 4, and vic-dichloride 5.5 We have been interested in introducing one sulfur atom into alkenes, that is, directly synthesizing thiiranes from alkenes. Although some number of direct syntheses has been reported so far, most of the methods seem to be specific to the starting alkenes and present difficulties in synthesizing sulfurating agents.7 Furthermore, most alkenes used in the study were hard to handle in laboratories, because the separation and analysis of the reaction mixture were difficult to achieve. We have designed a model alkene suitable for investigation into the reaction conditions for direct synthesis because of its ease in handling. Using 1 and 2 in the study would facilitate the analysis of the reaction mixture by 1H NMR and, therefore, 1 and 2 were thought to be a good candidate for the alkene. However, their synthesis from a commercial compound is lengthy and an extra reaction step is required in order to separate the two alkenes.6 We have designed a novel alkene carrying a 9-benzonorbornenylidene group to overcome the faults of 1 and 2. Here, we report the synthesis of 2'-adamantylidene-9-benzonorbornenylidene and its sulfuration.
RESULTS AND DISCUSSION
Synthesis of 2'-Adamantylidene-9-benzonorbornene 6
2’-Adamantylidene-9-benzonorbornenylidene 6 was synthesized from commercially available 2-adamantanone 7 in three steps. The ketone 7 was treated with cyclopentadiene in the presence of pyrrolidine to give fulvene 8.8 Reaction of 8 with benzyne, generated by reaction of anthranilic acid with
isoamyl nitrite in CH2Cl2 and dioxane at reflux, afforded diene 9 in 96% yield together with bis-adduct 10 in 3% yield. The diene 9 was hydrogenated with HN=NH, generated by oxidation of H2NNH2 with NaIO4, occurred chemoselectively to give 6 as colorless crystals in 98% yield.9 Hydrogenation with HCO2NH4 in the presence of 5% Pd/C in MeOH/CHCl3 (1:1) at reflux also afforded 6 in 87% yield.10 Neither 11 nor 12 was obtained in either reaction.
The alkene 6 showed five multiplets centered at δ 1.21 (2H), 1.76 (14H), 3.77 (2H), 7.04 (2H), and 7.14 (2H) and one broad singlet at δ 2.56 (2H) in the 1H NMR spectrum. The bridgehead hydrogen signal of the 2-benzonorbornenylidene group (δ 3.77) appeared at a lower field than the corresponding signals of 1 (δ 3.65) and 2 (δ 3.66),6 whereas that of the 2-adamantylidene group (δ 2.56) appeared at a higher field than the corresponding signals of 2,2’-biadamantylidene 13 (δ 2.95).11 The 13C NMR spectrum showed a higher field shift of the sp2 carbon signal of the 2-adamantylidene group (δ 126.9) and a lower field shift of the sp2 carbon signal at the 9-position of the 9-benzonorbornenylidene group (δ 141.3) by comparison with the corresponding carbon signals of 1 (δ 134.1), 2 (δ 134.3), and 13 (δ 133.1). These sizable upfield or downfield shifts would be caused by the homoconjugation interaction between the central C=C bond and the benzene ring shown as 6”.12
The bis-adduct 10 was a product by cycloaddition reaction of 9 and benzyne.13 The structure of 10 was exo- or endo-adduct, 10a or 10b, with respect to the benzene ring of 9. Since no single crystals of 10 suitable for an X-ray analysis were obtained, the 1H NMR spectrum of 10 was compared with the spectra of 10a and 10b calculated by Gaussian 98.14 Thus, 10 showed five multiplets centered at δ 0.75 (2H), 1.59 (10H), 3.24 (2H), 3.86 (2H), and 7.12 (8H) and one broad singlet at δ 2.39 (2H) in the 1H NMR spectrum. The multiplet centered at δ 0.75, assigned to axial hydrogens (Ha) at the 4-position of the adamantylidene group, appeared at a higher field than the corresponding signals in 9 and 6, and that centered at δ 3.24, assigned to hydrogens (Hb) on the cyclobutene ring, developed newly when the further benzene ring was introduced into 6. The calculation of 10 at B3LYP/6-311+G(2d,p) level shows that the Ha and Hb signals appear at δ 0.96 and 3.13 for 10a and at δ 1.86 and 3.74 for 10b, respectively, revealing that the structure of 10 proves to be 10a. The ring current effect of the close benzene ring would result in the higher field shift of the signal of Ha.
Sulfuration of 2'-Adamantylidene-9-benzonorbornene 6 by Elemental Sulfur
The alkene 6 was sulfurated with elemental sulfur (1 molar equiv. as S8) in refluxing o-Cl2C6H4 to furnish anti- and syn-thiirane, 14 and 15, in 76 and 4% yields, respectively, together with 6 in 19% yield. The sulfuration in DMF at 135 °C afforded 14 and 15 in 54 and 6% yields, respectively, along with 6 in 40% yield. Prolonging the reaction time did not bring about the disappearance of 6 in spite of the presence of an excess of sulfur atoms. These observations suggest that sulfuration must be reversible.15
Actually, 15 reacted with and without elemental sulfur in refluxing o-Cl2C6H4 to afford about 19:1:5 and 23:1:9 mixtures of 14, 15, and 6, respectively. The reactions of 14 gave almost same the product ratios as did that of 15. The calculations for 14 and 15 at the B3LYP/6-311+G(2d,p) level predict that 14 is thermally more stable by 3.2 kcal mol–1 than is 15.14 The energy difference means that the calculated equilibrium ratio of 14 to 15 at 180 °C, the boiling point of o-Cl2C6H4, is 32:1. The solvent effect would make the calculated ratio slightly higher than the actual ratios. Two mechanisms of the reversible process might be possible. One includes direct decomposition of the thiiranes to 6 and elemental sulfur, followed by the resulfuration of 6. The other contains three consequent processes: 1) cleavage of the C—S bond close to the 9-benzonorbornenylidene group heterolytically or homolytically to give intermediates 16 and 17, or 18 and 19, 2) rotation about the central C—C bond, and 3) recombination of the C—S bond or elimination of sulfur from the intermediates.
Structure of 14 and 15
A molecular structure of 14 is shown in Figure 1. Selected bond length and bond angle data are summarized in Table 1. The C(2)—C(3) bond length, 1.477(2) Å, is slightly shorter than that of the parent thiirane, 1.484 Å,16 and is slightly longer than those of 3 and 4, 1.464(3) and 1.455(4) Å,6 respectively. At the same time, the S(1)—C(2) bond length, 1.817(2) Å, is nearly equal to that of the parent thiirane, 1.815 Å, but the S(1)—C(3) bond length, 1.856(2) Å, is longer. The C(2)—S(1)—C(3) and S(1)—C(3)—C(2) bond angles, 47.39(7) and 64.89(9)°, respectively, are smaller than those of the parent thiirane, 48.16 and 65.52°,16 respectively, but the S(1)—C(2)—C(3) bond angle, 67.72(9)° is larger. Deformation of the thiirane ring is probably due to steric repulsion among the S(1) atom and some hydrogen and carbon atoms in the substituents.
In the 1H NMR spectra, the two hydrogen atoms of the methylenes of 14, which face the benzene ring and hence are placed under the influence of the ring current effect of the benzene ring,6 appeared as a multiplet centered at δ 1.07, which is a higher field than the multiplet centered at δ 2.00 assigned to the corresponding methylene hydrogens of 15. The bridgehead protons in the benzonorbornenylidene group of 14 and 15 appeared as a multiplet centered at δ 3.12 and 3.29, respectively, which are apart from the corresponding protons of 6 (δ 3.77), suggesting that, if a reaction mixture of the sulfuration of 6 contains only 14, 15, and 6 as compounds having protons that appeared in the range δ 3–4, a ratio of the mixture could easily be determined by 1H NMR analysis.
Sulfurations of 6 by Ph3CSCl and Ph3CSSCl
The alkene 6 reacted with 1 molar equiv. of Ph3CSCl17 in CH2Cl2 at –78 °C to give 14 in 60% yield along with 6 in 40% yield. A mixture of 14, 15, and 6 was obtained when the reactions were performed above –18 °C, and the ratio of 14 to 15 decreased as the reaction temperature increased. The yield of 6 increased
at rt because Ph3CSCl decomposes into Ph3CCl and elemental sulfur by the thiiranes prior to reacting with 6.5 In fact, Ph3CSCl reacted with 14 in CH2Cl2 at rt to form Ph3CCl, elemental sulfur, and 14 quantitatively. In contrast to these results, when 6 reacted with 1 molar equiv. of Ph3CSSCl, the ratio of 14 to 15 increased as the reaction temperature increased. In particular, the reaction at rt furnished 14 exclusively. The possible mechanism of the sulfurations is as follows. The alkene 6 reacts with Ph3CSCl to form thiiranium intermediates, 20 and 21. The intermediates liberate triphenylmethylcarbenium ions, which react with chloride ions to give Ph3CCl, via an E1 process to form the thiiranes.
On the other hand, 6 reacts with Ph3CSSCl to afford thiiranium intermediates, 22 and 23. The intermediates have the less polar Ph3C–S bond compared with 20 and 21, hence the liberation of triphenylmethylcarbenium ion from 22 and 23, which form thiirane sulfides, 26 and 27,18 and Ph3CCl, becomes slower. The C—S bond of 22 and 23 that is close to their 9-benzonorbornenylidene group cleaves easily to form carbenium salts, 24 and 25, which are stabilized by neighboring group participation of their benzene ring. Rotation about the central C—C bond of the carbenium salts, followed by recombination of the C—S bond takes place to furnish 26 and 27.19 The equilibrium among 22—25 in which 22 must be most stable, occurs under the reaction conditions. Finally, 26 and 27 eliminate sulfur to give 14 and 15, respectively.
Sulfurations of 6 by S2Cl2 and SCl2
The alkene 6 was sulfurated with 1 molar equiv. of S2Cl2 in CH2Cl2 at –78 °C. After the sulfuration was quenched by the addition of water at the same temperature, a 68:32 mixture of 14 and vic-dichloride 28 was obtained. Purification of the mixture by silica gel column chromatography furnished 14 and ketone 29 in 68 and 32% yields, respectively.20 The ketone 29 is most likely formed by silica gel catalyzed hydrolysis of 28 to chlorohydrin 32 through formation of the carbenium ion 30, which is in equilibrium with the epichloronium ion 31, followed by pinacol-type rearrangement of 32. The vic-dichloride 28 was not obtained from the reaction mixture in pure form. Therefore, 28 was synthesized separately by chlorine addition of 6 to confirm its structure. Reaction of 6 with 1.0 molar equiv. of SO2Cl2 in CH2Cl2 at –78 °C gave 28 whose spectral data were the same as those of the product that was formed by the sulfuration in quantitative yield.
Temperature-variable 1H NMR spectra of 28 showed that all signals broaden at rt and separate sharply as the temperature falls. The signal (δ 0.44) due to the Hc atom at the 1-position of the adamantylidene group appeared at a higher field than that (δ 2.99) due to the Hd atom at the 3-position at –50 °C, revealing that 28 adopts a gauche conformation like 28’ at low temperatures.21 Rotation about its central C—C bond occurs as the temperature gradually rises.
Sulfuration with 1.0 molar equiv. of SCl2 furnished 14 and 28 in 42 and 57% yields, respectively. When 6 was sulfurated with 0.5 molar equiv. of S2Cl2 at –78 °C, 14 and 15 were obtained in 71% and 7% yields, respectively, revealing that two sulfur atoms of S2Cl2 are involved with the formation of thiiranes and, even if 15 is formed by the sulfuration, further reaction of 15 with S2Cl2 would occur to form 14 and 28.
In fact, 15 reacted with S2Cl2 under the same conditions as the sulfurations of 6 to afford 14 and 28 in 91% and 9% yields, respectively. The same reaction of 15 with SCl2 and those of 14 with S2Cl2 and SCl2 occurred to give a mixture of 14 and 28.
The possible mechanism of the sulfuration with SXCl2 is as follows. The alkene 6 reacts with SXCl2 to form thiiranium salts, 33 and 34. Then, 14 and 15 are produced from the salts together with SCl2 (X = 2) or Cl2 (X = 1). Cl2 is also produced by equilibrium SCl2 and S2Cl2,22 and reacts with 6 to give 28. After 33 and 34 come to equilibrium with the carbenium salts, 35 and 36, isomerization of 15 to 14 and formation of the sulfanyl chlorides, 37 and 38, proceed. The reason 14 is a main product having a sulfur atom seems to be its thermal stability compared with 15. The epichloronium salt 31 is formed by elimination of sulfur from 37 and 38, and then is finally transformed into 28.
CONCLUSION
We succeeded in synthesizing a novel alkene 6 from commercially available 2-adamantanone in three steps. The sulfuration of 6 with elemental sulfur proceeded to give a mixture of thiiranes 14 and 15. The syn-thiirane 15 was readily transformed into the alkene 6 and thermally more stable 14 under the sulfuration conditions, although no other products having sulfur atoms such as polymers and cyclic oligosulfanes were observed. The sulfuration with SCl2 and S2Cl2 afforded 14 and vic-dichloride 28. A ratio of the sulfuration products could easily be determined by their 1H NMR spectra. From these results, we believe that 6 is a good candidate for the model alkene for studying the reaction conditions of the direct synthesis of thiiranes from alkenes, and have been using 6 to investigate the reaction conditions of direct synthesis with general applicability.
EXPERIMENTAL
Solvents were dried and purified in the usual manner. All the reactions were carried out under argon. Silica-gel column chromatography was performed on silica gel 60N (Kanto, 63—210 µm, spherical, neutral). Melting points were determined on a Mel-Temp capillary tube apparatus and are uncorrected. 1H and 13C-NMR spectra were recorded on a Bruker DRX400, a Bruker ARX400, a Bruker AM 400 (400.4 MHz for 1H and 100.6 MHz for 13C), a Bruker AC300P (300.1 MHz for for 1H and 75.5 MHz for 13C), or a Bruker AC200 (200.1 MHz for for 1H and 50.3 MHz for 13C) spectrometer using CDCl3 or CD2Cl2 as the solvent with TMS for 1H and with CDCl3 or CD2Cl2 for 13C as the internal standard. IR spactra were recorded on a Hitachi FT-IR 660+ spectrophotometer. Mass spectra were recorded on a JEOL JMS-DX303 spectrometer operating at 70 eV in the EI mode or using m-nitrobenzyl alcohol as a matrix in the FAB mode. Elemental analyses were performed by the molecular Analysis and Life Science Center of Saitama University.
Reaction of Fulvene 8 with Benzyne. To a solution of 88 (4.0 g, 20 mmol) and isoamyl nitrite (4.1 mL, 30 mmol) in CH2Cl2 (160 mL) was added dropwise a solution of anthranilic acid (4.15 g, 30 mmol) in dioxane (40 mL) at reflux for 1 h. After the addition was finished, the reaction mixture was cooled to rt and then evaporated. Hexane and aqueous NaHCO3 solution were added to the residue and then the insoluble materials were collected by filtration. After the filtrate was separated, the organic layer was washed with H2O, dried over K2CO3, and evaporated. The residue was chromatographed on a column of silica gel and the column was eluted with hexane to give 5.3 g (96%) of 9 and 0.21 g (3%) of 10 in this order. 2'-Adamantylidene-9-benzonorbornadienylidene 9: colorless crystals (from CH2Cl2 and hexane); mp 90—91 °C; 1H NMR (300.1 MHz, CDCl3) δ 1.42—1.60 (m, 2H), 1.65—1.95 (m, 10H), 2.58 (br s, 2H), 4.30—4.40 (m, 2H), 6.80—7.00 (m, 4H), 7.11—7.28 (m, 2H); 13C NMR (75.5 MHz, CDCl3) δ 28.3, 28.5, 32.7, 37.2, 38.3, 38.5, 49.7, 117.4, 120.5, 124.1, 143.2, 151.0, 155.3; IR (KBr) 3061, 3002, 2990, 2923, 2851, 1556, 1447, 784, 761, 749, 723, 693, 552, 447 cm–1. Anal. Calcd for C21H22: C, 91.92; H, 8.08. Found: C, 91.78; H, 8.23. 2'-Adamantylidene-9-(2,3-o-phenylenebenzonorbornadienylidene) 10: colorless powder (from benzene); mp 295—298 °C; 1H NMR (400.4 MHz, CD2Cl2) δ 0.69—0.77 (d-like, 2H), 1.43—1.57 (m, 5H), 1.60—1.68 (m, 4H), 1.72—1.77 (m, 1H), 2.38 (br s, 2H), 3.29 (s, 2H), 3.87 (s, 2H), 7.07—7.13 (m, 4H), 7.17—7.21 (m, 2H), 7.23—7.27 (m, 2H); 13C NMR (100.6 MHz, CD2Cl2) δ 27.9, 28.3, 33.7, 37.2, 38.5, 38.8, 45.6, 49.5, 120.8, 122.2, 125.5, 127.3, 133.5, 136.3, 145.6, 147.4; IR (KBr) 3067, 2994, 2951, 2916, 2894, 2841, 1456, 1445, 767, 752, 743, 686, 678, 439 cm–1. Anal. Calcd for C27H26: C, 92.52; H, 7.48. Found: C, 92.26; H, 7.52.
Reaction of 9 with Diimide. To a mixture of 9 (1.60 g, 5.8 mmol), H2NNH2•H2O (7.3 g, 140 mmol), and saturated CuSO4 aqueous solution (2.0 mL) in CHCl3 (10 mL) and MeOH (10 mL) was added dropwise a solution of NaIO4 (6.2 g, 30 mmol) in water (60 mL) at rt for 1 h. After the addition was finished, the organic layer was separated, washed with H2O, dried over MgSO4, and evaporated. 2'-Adamantylidene-9-benzonorbornenylidene 6 was obtained in quantitative yield (1.61 g). 6: colorless crystals (from hexane); mp 115—116 °C; 1H NMR (300.1 MHz, CDCl3) δ 1.18—1.24 (m, 2H), 1.55—1.97 (m, 14H), 2.56 (br s, 2H), 3.73—3.80 (m, 2H), 7.00—7.08 (m, 2H), 7.10—7.17 (m, 2H); 13C NMR (75.5 MHz, CDCl3) δ 27.3, 28.4, 28.6, 34.0, 37.3, 38.5, 39.7, 42.8, 119.8, 125.2, 126.9, 141.3, 147.9; IR (KBr) 3070, 3015, 2992, 2968, 2953, 2899, 2862, 2845, 1466, 1446, 755, 730, 675, 572, 467, 457 cm–1. Anal. Calcd for C21H24: C, 91.25; H, 8.75. Found: C, 91.15; H, 8.78.
Hydrogenation of 9 by Ammonium Formate in the presence of Pd/C. Ammonium formate (1030 mg, 16.3 mmol) and 5% palladium on charcol (47 mg) was added to a solution of 9 (447 mg, 1.6 mmol) in CHCl3 (4.0 mL) and MeOH (4.0 mL). After being heated at reflux for 1 day, the insoluble material was removed by filtration on a pad of Celite. The filtrate was washed with H2O, dried over MgSO4, and evaporated. The compound 6 was obtained in 87% yield (392 mg).
Sulfuration of 6 by Elemental Sulfur in Refluxing o-Cl2C6H4. A suspension of 6 (970 mg, 3.5 mmol) and elemental sulfur (898 mg, 3.5 mmol as S8) in o-Cl2C6H4 (10 mL) was heated under reflux for 48 h. The mixture was evaporated under reduced pressure. The residue was placed on a column of silica gel and the column was eluted with hexane to give 190 mg (20%) of 6 and with CHCl3/hexane (1:4) to give 821 mg (76%) of 14 and 39 mg (4%) of 15. anti-Thiirane 14: colorless crystals (from CH2Cl2 and MeOH); mp 164—166 °C; 1H NMR (400.4 MHz, CDCl3) δ 1.02—1.12 (m, 2H), 1.31—1.40 (m, 2H), 1.40—1.46 (m, 1H), 1.50—1.60 (m, 2H), 1.63 (br s, 2H), 1.70—1.82 (m, 4H), 1.88—1.93 (m, 1H), 1.99—2.04 (m, 2H), 2.23—2.35 (m, 2H), 3.10—3.14 (m, 2H), 7.09—7.15 (m, 2H), 7.16—7.22 (m, 2H); 13C NMR (75.5 MHz, CDCl3) δ 26.4, 27.2, 27.6, 36.8, 37.3, 37.4, 38.2, 47.3, 66.1, 79.7, 120.7, 126.1, 146.5; IR (KBr) 3043, 3025, 2983, 2959, 2920, 2903, 2858, 2842, 1451, 1441, 736, 586, 480 cm–1; MS (EI) m/z 308 (M+, 100%), 309 (M++1, 26%), 310 (M++2, 8%). Anal. Calcd for C21H24S: C, 81.76; H, 7.84. Found: C, 81.72; H, 7.92. syn-Thiirane 15: colorless crystals (from CH2Cl2 and MeOH); mp 202—203 °C (decomp.); 1H NMR (400.4 MHz, CDCl3) δ 1.42—1.49 (m, 2H), 1.80—1.90 (m, 6H), 1.93—2.11 (m, 8H), 2.25—2.33 (m, 2H), 3.26—3.32 (m, 2H), 7.09—7.15 (m, 2H), 7.17—7.23 (m, 2H); 13C NMR (75.5 MHz, CDCl3) δ 26.8, 27.0, 27.8, 37.2, 37.6, 38.4, 38.8, 49.0, 70.3, 83.0, 120.7, 125.7, 147.6; IR (KBr) 3048, 2998, 2987, 2972, 2918, 2845, 1456, 763, 743, 578, 498 cm–1; MS (EI) m/z 308 (M+, 100%), 309 (M++1, 26%), 310 (M++2, 8%). Anal. Calcd for C21H24S: C, 81.76; H, 7.84. Found: C, 81.81; H, 7.93.
Sulfuration of 6 by Elemental Sulfur in DMF at 135 °C. A suspension of 6 (8.0 mg, 29 µmol) and elemental sulfur (7.4 mg, 29 µmol as S8) in DMF (0.6 mL) was heated at 135 °C for 20 h. The mixture was evaporated under reduced pressure to give 15.4 mg of a mixture of 14 (54%), 15 (6%), and 5 (40%).
X-Ray Crystallographic Analysis of anti-Thiirane 14. A single crystal of 14 of suitable quality and size was obtained by crystallization from a mixture of CH2Cl2 and hexane. Oscillation and nonscreen Weissenberg photographs were collected on the imaging plates of the MAC DIP3000 diffractometer by using Mo-Kα radiation (λ = 0.71073 Å) and the data collection was made by the MAC DENZO program system. Absorption collection was made by multi-scan method (SORTAV). Cell parameters were determined and refined by using the MAC DENZO for all observed reflections. The structure was solved by direct methods using SIR97 in the maXus program system. The atomic coordinates and the anisotropic thermal parameters of the non-H atoms were refined on F2 by full matrix least squares using SHELXL-97 {weighting scheme: w = 1/[σ2(F2) + (0.0493P)2] where P = (FO2 + 2FC2)/3}. C21H24S (308.49), orthorhombic, space group Pnma, a = 18.295(<1), b =10.430(<1), c = 8.417(<1) Å, V = 1606.11(10) Å3, Z = 4, Dcalc = 1.276 Mgm–3, µ = 0.196 mm–1, crystal dimension: 0.23 × 0.19 × 0.14 mm, θ range: 0—27.07°, number of measured reflections: 2020, number of independent reflections: 1844, number of observed reflections: 1423 [I > 2σ(I)], refined parameters: 159, R = 0.0499 [I > 2σ(I)], wR2 = 0.0953 (all reflections), S = 0.972, (Δ/σ)max = <0.001, Δρmax = 0.185 eÅ–3, Δρmin = –0.369 e Å–3.
General Procedure for Sulfuration of 6 by Ph3CSXCl. To a solution of 6 in CH2Cl2 was added dropwise a solution of Ph3CSXCl (1.0 molar equiv.) in CH2Cl2 at –78 °C, –18 °C or rt. After being stirred for 2 h at the same temperature, the reaction was quenched by addition of a solution of NaOEt in MeOH. The organic layer was washed with H2O, dried over K2CO3, and evaporated. The residue was placed on a column of silica gel and the column was eluted with CHCl3/hexane (1:4) to give a mixture of 14, 15, and 6.
Sulfuration of 6 by 1.0 molar equiv. of S2Cl2 at –78 °C. To a solution of 6 (100 mg, 360 µmol) in CH2Cl2 (10 mL) was added dropwise a solution of S2Cl2 (49 mg, 360 µmol) in CH2Cl2 (5 mL) at –78 °C. After being stirred for 30 min at same temperature, the reaction was quenched by addition of ice water. The organic layer was separated, washed with H2O, dried over K2CO3, and evaporated to give 131 mg of a 68:32 mixture of 14 and 28. The mixture was placed on a column of silica gel and the column was eluted with CHCl3/hexane (1:4) to give 76 mg of 12 (68%) and with CHCl3 to give 34 mg of 29 (32%). Ketone 29: colorless crystals (from CH2Cl2 and hexane); mp 189—191 °C; 1H NMR (400.4 MHz, CDCl3) δ 0.88 (br s, 1H), 1.28—1.48 (m, 3H), 1.55—1.75 (m, 5H), 1.85 (br s, 1H), 1.91 (br s, 1H), 1.98—2.07 (m, 2H), 2.10—2.22 (m, 2H), 2.26—2.31 (m, 2H), 2.68—2.75 (m, 1H), 3.49—3.52 (m, 1H), 3.87—3.89 (m, 1H), 7.13—7.24 (m, 4H); 13C NMR (75.5 MHz, CDCl3) δ 19.3, 23.7, 26.9, 27.5, 31.4, 32.4, 32.8, 32.9, 33.2, 33.3, 39.3, 42.6, 51.5, 54.7, 124.7, 125.0, 126.7, 126.8, 136.3, 142.4, 214.5; IR (KBr) 3036, 2967, 2951, 2905, 2853, 1700, 760, 753, 490 cm–1; MS (FAB) m/z 293 (MH+). Anal. Calcd for C21H24O: C, 86.25; H, 8.27. Found: C, 85.97; H, 8.38.
Synthesis of 28 by Reaction of 6 by SO2Cl2 at –78 °C. To a solution of 6 (100 mg, 360 µmol) in CH2Cl2 (5.0 mL) was added dropwise a solution of SO2Cl2 (49 mg, 360 µmol) in CH2Cl2 (3.0 mL) at –78 °C. After being stirred for 15 h at the same temperature, the reaction was quenched by addition of ice water. The organic layer was separated, washed with H2O, dried over K2CO3, and evaporated to give 125 mg of 28 (quantitatively). The 1H and 13C NMR spectra of 28 are consistent with those of the product by the reaction of 6 with S2Cl2. vic-Dichloride 28: colorless crystals (from CH2Cl2 and MeOH); mp 126—127 °C; 1H NMR (300.1 MHz, CDCl3, 25 °C) δ 1.02—2.65 (m, 18H), 4.76—4.14 (br s, 2H), 6.98—7.22 (m, 4H); 1H NMR (400.4 MHz, CDCl3, –50 °C) δ 0.43—0.44 (m, 1H), 1.01—1.07 (m, 1H), 1.19—1.23 (m, 2H), 1.30—1.36 (m, 1H), 1.52—1.66 (m, 4H), 1.77—1.81 (m, 2H), 1.98—2.02 (m, 1H), 2.16—2.23 (m, 2H), 2.41—2.47 (m, 2H), 2.56—2.61 (m, 1H), 2.98—2.99 (m, 1H), 3.80—3.87 (m, 1H), 4.14—4.16 (m, 1H), 7.14—7.20 (m, 4H); 13C NMR (100.6 MHz, CDCl3, –50 °C) δ 25.5, 26.0, 27.0, 29.1, 33.5, 35.9, 36.0, 36.1, 38.0, 38.1, 40.3, 55.4, 57.3, 89.2, 97.8, 120.2, 121.6, 126.2, 126.6, 143.2, 144.2; IR (KBr) 3032, 3006, 2976, 2953, 2901, 2862, 1470, 1453, 1279, 1099, 862, 797, 763, 746, 582, 508 cm–1; MS (EI) m/z 129 (100%), 346 (M+, 94%), 348 (M++2, 61%), 350 (M++4, 12%). Anal. Calcd for C21H24Cl2: C, 72.62; H, 6.97. Found: C, 72.57; H, 7.01.
General Procerdure for Sulfuration of 6 by SXCl2 at –78 °C. To a solution of 6 (100 mg, 360 µmol) in CH2Cl2 (10 mL) was added dropwise a solution of SXCl2 (1.0 or 0.5 molar equiv.) in CH2Cl2 (5.0 mL) at –78 °C. After being stirred for 30 min at the same temperature, the reaction was quenched by addition of ice water. The organic layer was separated, washed with H2O, dried over K2CO3, and evaporated. A ratio of the the products was estimated by 1H NMR.
General Procerdure for Reactions of 14 or 15 with 1.0 molar equiv. of SXCl2 at –78 °C. To a solution of 14 or 15 in CH2Cl2 was added dropwise a solution of SXCl2 (1.0 molar equiv.) in CH2Cl2 at –78 °C. After being stirred for 30 min at the same temperature, the reaction was quenched by addition of ice water. The organic layer was separated, washed with H2O, dried over K2CO3, and evaporated. A ratio of the the products was estimated by 1H NMR.
ACKNOWLEDGEMENTS
This work was supported by Grants-in-Aid for Scientific Research from Japan Society for the Promotion of Science.
References
. †Dedicated to Prof. Kunio Ogasawara on the occasion of his 70th birthday.
1. M. G. Voronkov, N. S. Vyazankin, E. N. Deryagina, A. S. Nakhmanovich, and V. A. Usov, ‘Reactions of Sulfur with Organic Compounds,’ Plenum, New York, 1987.
2. P. D. Bartlett and T. Ghosh, J. Org. Chem., 1987, 52, 4937. CrossRef
3. J. Nakayama, Y. Ito, and A. Mizumura, Sulfur Lett., 1992, 14, 247.
4. Y. Sugihara, H. Takeda, and J. Nakayama, Tetrahedron Lett., 1998, 39, 2605; CrossRef Y. Sugihara, H. Takeda, and J. Nakayama, Eur. J. Org. Chem., 1999, 597. CrossRef
5. Y. Sugihara, K. Noda, and J. Nakayama, Tetrahedron Lett., 2000, 41, 8913. CrossRef
6. Y. Sugihara, K. Noda, and J. Nakayama, Bull. Chem. Soc. Jpn., 2000, 73, 2351. CrossRef
7. W. Adam and R. M. Bargon, Chem. Rev., 2004, 104, 251 and references cited therein. CrossRef
8. X. Zhang, S. I. Khan, and C. S. Foote, J. Org. Chem., 1995, 60, 4102. CrossRef
9. J. M. Hoffman, Jr. and R. H. Schlessinger, Chem. Commun., 1971, 1245. CrossRef
10. H. S. P. Rao and K. S. Reddy, Tetrahedron Lett., 1994, 35, 171. CrossRef
11. Y. Kabe, T. Takata, K. Ueno, and W. Ando, J. Am. Chem. Soc., 1984, 106, 8174. CrossRef
12. H.-O. Kalinowski, S. Berger, and S. Braun, ‘Carbon-13 NMR Spectroscopy (translated by J. K. Becconsall),’ John Wiley and Sons, Chichester, 1984, p. 137.
13. H. E. Simmons, J. Am. Chem. Soc., 1961, 83, 1657. CrossRef
14. M. J. Frisch, G. W. Trucks, H. B. Schlegel, G. E. Scuseria, M. A. Robb, J. R. Cheeseman, V. G. Zakrzewski, J. A. Montgomery, Jr., R. E. Stratmann, J. C. Burant, S. Dapprich, J. M. Millam, A. D. Daniels, K. N. Kudin, M. C. Strain, O. Farkas, J. Tomasi, V. Barone, M. Cossi, R. Cammi, B. Mennucci, C. Pomelli, C. Adamo, S. Clifford, J. Ochterski, G. A. Petersson, P. Y. Ayala, Q. Cui, K. Morokuma, D. K. Malick, A. D. Rabuck, K. Raghavachari, J. B. Foresman, J. Cioslowski, J. V. Oritz, A. G. Baboul, B. B. Stefanov, G. Liu, A. Liashenko, P. Piskorz, I. Komaromi, R. Gomperts, R. L. Martin, D. J. Fox, T. Keith, M. A. Al-Laham, C. Y. Peng, A. Nanayakkara, C. Gonzalez, M. Challacombe, P. M. W. Gill, B. Johnson, W. Chen, M. W. Wong, J. L. Andres, C. Gonzalez, M. Head-Gordon, E. S. Replogle, and J. A. Pople, Gaussian, Inc., Pittsburgh PA, 1998, Gaussian 98, Revision A.7.
15. W. Chew and D. N. Harpp, Sulfur Rep., 1993, 15, 1 and references cited therein. CrossRef
16. K. Okiye, C. Hirose, D. G. Lister, and J. Sheridan, Chem. Phys. Lett., 1974, 24, 111; CrossRef W. Ando, N. Choi, and N. Tokitoh, ‘Comprehensive Heterocyclic Chemistry II,’ Vol. 1A, ed. by A. Padwa, Pergamon, Oxford, 1996, Chap. 1.05.
17. I. A. Abu-Yousef and D. N. Harpp, Sulfur Rep., 2003, 24, 255 and references cited therein.
18. I. A. Abu-Yousef and D. N. Harpp, J. Org. Chem., 1998, 63, 8654. CrossRef
19. Y. Sugihara, Y. Aoyama, and J. Nakayama, Chem. Lett., 2001, 980. CrossRef
20. R. T. Baker, J. C. Calabrese, S. A. Westcott, and T. B. Maeder, J. Am. Chem. Soc., 1995, 117, 8777. CrossRef
21. Y. Sugihara, K. Noda, and J. Nakayama, Tetrahedron Lett., 2000, 41, 8907. CrossRef
22. F. A. Cotton, G. Wilkinson, C. A. Murillo, and M. Bochmann, ‘Advanced Inorganic Chemistrey,’ 6th ed., Wiley-Interscience, New York, 1999, pp. 518-519