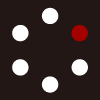
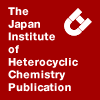
HETEROCYCLES
An International Journal for Reviews and Communications in Heterocyclic ChemistryWeb Edition ISSN: 1881-0942
Published online by The Japan Institute of Heterocyclic Chemistry
e-Journal
Full Text HTML
Received, 21st July, 2008, Accepted, 3rd September, 2008, Published online, 3rd September, 2008.
DOI: 10.3987/COM-08-S(F)51
■ Synthesis and Biological Activities of VDR Antagonists; 25-Modified 1α,25-Dihdyroxyvitamin D3-26,23-Lactam (DLAM) Derivatives
Yuko Kato-Nakamura, Jun-ichi Namekawa, Seiichi ishizuka, Hiroshi Saitoh, Kazuya Takenouchi, and Kazuo Nagasawa*
Department of Biotechnology and Life Science, Tokyo University of Agriculture and Technology, 2-24-16 Nakamachi, Koganei, Tokyo 184-8588, Japan
Abstract
Three series of DLAM (1α,25-dihydroxyvitamin D3-26,23-lactam) derivatives (9 compounds) with 25-modification were synthesized. The biological evaluation of these derivatives revealed that the bulkiness at the 25-position of the DLAM causes a decrease in both the binding affinity for VDR and antagonistic activity.1α,25-Dihydroxyvitamin D3 (1, Figure 1) (1,25-D3), which is a hormonally active form of vitamin D3, exhibits various physiological actions including regulation of calcium homeostasis, bone mineralization, proliferation and differentiation of various types of cells, and immune modulation.1 Most of these actions of 1,25-D3 are mediated by its specific receptor, vitamin D receptor (VDR), which is a member of the nuclear receptor (NR) superfamily and acts as ligand-dependent gene transcriptional factor with coactivators.2, 3
More than 3000 analogs of 1,25-D3 have been synthesized as candidate ligands for VDR, and most of them were reported to show agonistic activities. Only four types of ligands, the 25-carboxylic ester derivative 2 (ZK168281),4 26,23-lactone 3 (TEI-9647),4c,5 AD-47 (4)6 and DLAM (1α,25-dihydroxyvitamin D3-26,23-lactam),7 have been reported as antagonists so far (Figure 1). These VDR antagonists are expected to be effective for the treatment of metabolic bone disease represented by Paget’s disease8 as well as biological tools for the elucidation of VDR function and the mode of action of 1,25-D3.
We have recently synthesized new DLAM derivatives having various aralkyl group on the nitrogen of the lactam ring ((23S,25S)-DLAM-1P (5)~4P (8)) and their stereoisomers. The biological activities of these compounds were evaluated, and the orders of the VDR binding affinity and antagonistic activity of these DLAM derivatives were found to be correlated.7c In our continuous structure-activity relationship (SAR) studies on DLAM derivatives, we focused on the substitution at C-25 position on DLAM, since some of the analogs on 1,25-D3 were reported to increase their binding affinities to VDR and/or activities by modifying the alkyl chain at C-25.9 Herein, we described the synthesis of C-25 modified new DLAM derivatives and evaluation of their VDR binding affinity and antagonistic activity.
Outset of our SAR studies, DLAM-2P was selected as a core skeleton for the structural development since one of its stereoisomers of (23S,25S)-DLAM-2P (6) showed the highest binding affinity for VDR with strongest antagonistic activity among the DLAMs.7c Thus, based upon our 1,3-diploar cycloaddition methodology, C-25 modified new DLAM-2P derivatives (9~11) were synthesized (Scheme 1).
The aldehyde 1210 was reacted with 2-phenylethylhydroxylamine to give the nitrone 13, which was subsequently reacted with the acrylic acid derivatives 14, 1511 and 1812 to give the isoxazolidines 16, 17, and 21, respectively, with four possible diastereomers at C23 and C25. The phthalimidomethyl ester of 16 and 17 was converted into methyl ester by hydrolysis with hydrazine followed by esterification of the resulting carboxylic acid with trimethylsilyl diazomethane to give 19 and 20. Reduction of the N-O bond in 19-21 with molybdenum hexacarobonyl simultaneous cyclization took place to give lactams 22, 23 and 24. The tertiary alcohol of 22-24 was protected as trimethylsilyl ether to give the CD-ring synthon 25-27. These CD-ring synthons 25-27 were reacted with enyne 2814 to construct the vitamin D3 triene skeleton in the presence of palladium catalyst. Finally, deprotection of the TBS and TMS groups with TBAF in THF at 50 °C gave 9-11 as a mixture of diastereomers at C23 and C25. These stereoisomers were separated with HPLC. In case of 9 and 11, three and two out of four possible diastereomers, i.e., 9a, 9b and 9d, and 11a and 11b, were isolated, respectively.15
The C-25 modified new DLAM-2P derivatives (9-11) in hand,16 the biological activities of these compounds were evaluated (Table 1). Firstly, the VDR binding affinity was examined by using the chick intestinal VDR as described previously.17 The VDR affinities of 9-11 were varied by the stereochemistries at C23 and C25, and substituents at C25. The (23S,25S)-stereoisomers of 9-11 showed higher binding affinities than other stereoisomers, and their binding affinities of 9a-11a were revealed to decrease with increasing their bulkiness at C-25-position, and compound 6 had the highest affinity with VDR (entries 1, 2, 5, and 9). Next we examined the antagonistic activities of 9-11 by the NBT-reduction method18 in terms of IC50 for differentiation of HL-60 cells induced by 10 nM of 1. As a result, only the DLAM derivatives with (23S,25S) stereochemistries of 9a-11a showed antagonistic activities. As in the case of the binding affinities for VDR, the antagonistic activities of them were reduced with increasing their bulkiness at C-25 position (entries 2, 5, and 9). With these experiments, structural development of DLAM-2P at C-25 with bulky substituents resulted in decreasing both VDR binding affinity and antagonistic activity. Since the VDR antagonistic activity of DLAM derivatives are elicited through the binding with VDR, sterically bulky group at C-25 was realized to cause only negative factors for binding by preventing the interaction of 25-hydroxyl group with His305 and His379 residues in VDR.19
In conclusion, structural development of DLAM at C-25 position was examined. The C-25 modified DLAM-2P derivatives 9-11 were synthesized, and evaluated their VDR binding affinity and antagonistic activity. As a result, the biological activities of both VDR binding affinity and antagonistic activity of these derivatives were decreased in relation to increasing the bulkiness at C-25 position. This result suggests that, as in the case of 1,25-D3 binding for VDR, the interaction between the 25-hydroxyl group of the DLAM and the VDR plays an important role in binding, and the steric hinderence at C-25 position in DLAM would affect negatively to the binding affinity and antagonistic activity in this case. Further SAR studies of DLAM derivatives and investigation to the variety of biological activities are in progress.
EXPREMENTAL
General
Flash column chromatography was performed on KP-SIL (Biotage Silica, 40-63 µm). NMR spectra were measured on JEOL AL-400 magnetic resonance spectrometers. Mass spectra were measured on Agilent LC/ MSD SL (Liquid Chromatograph mass spectrometer) and Shimadzu LCMS-IT-TOF (Liquid Chromatograph mass spectrometer-ion trap-Time of flight).
Preparation of DLAMs 9-11.
2-Ethylacrylic acid phthalimidomethyl ester (14). To a suspension of 2-ethylacrylic acid 29 (250 mg, 2.5 mmol) in DMF (8 mL) was added N-bromophthalimide (660 mg, 2.8 mmol) and KF (291 mg, 5.0 mmol), and the mixture was stirred at 80 ˚C for 12 h under argon atmosphere. The reaction mixture was concentrated. To the residue was added H2O (80 mL) and extracted with CH2Cl2. The organic layer was dried over MgSO4 and concentrated. The residue was purified by flash column chromatography on KP-SIL (hexane / AcOEt = 4 / 1) to give 14 (463 mg, 72%) as a white solid. 1H NMR (400 MHz, CDCl3) δ 7.94 (dd, J = 5.4, 3.2 Hz, 2 H), 7.79 (dd, J = 2.8, 1.4 Hz, 2 H), 6.16 (d, J = 1.0 Hz, 1 H), 5.80 (s, 2 H), 5.57 (d, J = 1.5 Hz, 1 H), 2.35-2.25 (m, 2 H), 1.06 (t, J = 7.4 Hz, 3 H).
2-Propylacrylic acid phthalimidomethyl ester (15). Similar to the synthesis of 14, a crude product, which was obtained from 2-propylacrylic acid 30 (300 mg, 2.6 mmol), N-bromophthalimide (695 mg, 2.9 mmol) and KF (306 mg, 5.3 mmol) in DMF (8 mL) at 80 ˚C for 12 h, was purified by flash column chromatography on KP-SIL (hexane / AcOEt = 4 / 1) to give 15 as colorless oil (643 mg, 90%). 1H NMR (400 MHz, CDCl3) δ 7.94 (dd, J = 5.4, 2.7 Hz, 2 H), 7.79 (dd, J = 5.4, 2.7 Hz, 2 H), 6.16 (d, J = 0.7 Hz, 1 H), 5.80 (s, 2 H), 5.57 (d, J = 1.5 Hz, 1 H), 2.32-2.23 (m, 2 H), 1.55-1.43 (m, 2 H), 0.96-0.89 (m, 3 H).
N-[3-(4-Bromomethylene-7α-methyloctahydroinden-1-yl)-1-butylidene]phenetylamine N-oxide (13). To a suspension of 3-(4-bromomethylene-7α-methyloctahydroinden-1-yl)butyraldehyde 1210 (788 mg, 2.64 mmol) in CH2Cl2 (25 mL) was added 2-phenyethylhydroxylamine (722 mg, 5.26 mmol) and Et3N (5.0 mL), and the mixture was stirred at rt for 1 h under argon atmosphere. To the mixture was added saturated NH4Cl aq. at 0 ˚C, and the aqueous layer was extracted with CH2Cl2. The organic layer was dried over MgSO4 and concentrated. The residue was purified by flash column chromatography on KP-SIL (hexane / AcOEt = 2 / 1) to give 13 (1.28 g, 99%) as colorless oil. 1H NMR (400 MHz, CDCl3) δ 7.31-7.20 (m, 5 H), 6.38 (t, J = 5.7 Hz, 1 H), 5.64 (s, 1 H), 3.97 (t, J = 6.7 Hz, 2 H), 3.23-3.19 (m, 2 H), 2.88-2.86 (m, 1 H), 2.46 (dt, J = 17.2, 4.4 Hz, 1 H), 2.28-2.19 (m, 1 H), 1.97-1.13 (m, 12 H), 0.79 (d, J = 6.6 Hz, 3 H), 0.53 (s, 3 H).
3-[2-(4-Bromomethylene-7a-methyloctahydroinden-1-yl)propyl]-5-ethyl-2-phenethylisoxazolidine-5-carboxylic acid methyl ester (19). To a suspension of 13 (577 mg, 1.43 mmol) in toluene (10 mL) was added the suspension of 14 (463 mg, 1.79 mmol) in toluene (5 mL), and the mixture was stirred at 90 ˚C for 12 h under argon atmosphere. The resulting mixture was concentrated. The residue was roughly purified by flash column chromatography on KP-SIL (hexane / AcOEt = 4 / 1). The resulting crude product was dissolved in n-butanol (25 mL). To the mixture was added hydrazine (3.3 mL), and stirred at 90 ˚C for 1 h under argon atmosphere. To the mixture was added water at 0 ˚C, and concentrated. The residue was diluted with AcOEt, and washed with 1N HCl. The organic layer was dried over MgSO4 and concentrated. The residue was roughly purified by flash column chromatography on KP-SIL (hexane / AcOEt = 4 / 1). The resulting crude product was dissolved in benzene / MeOH = 9 / 1 (5 mL). To the mixture was added TMSCHN2 (1.0 mL, 0.59 mmol), and stirred at rt for 15 min. The resulting mixture was concentrated to give 19 (269 mg, 41%) as colorless oil. 1H NMR (400 MHz, CDCl3) δ 7.32-7.15 (m, 5 H), 5.64 (brs, 1 H), 3.79-3.75 (m, 3 H), 3.10-0.72 (m, 30 H), 0.57-0.52 (m, 3 H).
3-[2-(4-Bromomethylene-7a-methyloctahydroinden-1-yl)propyl]-5-propyl-2-phenethylisoxazolidine-5-carboxylic acid methyl ester (20). Similar to the synthesis of 19, to a suspension of 13 (700 mg, 1.74 mmol) in toluene (10 mL) was added the suspension of 15 (643 mg, 2.35 mmol) in toluene (7 mL), and the mixture was stirred at 90 ˚C for 12 h under argon atmosphere. The resulting mixture was concentrated. The residue was roughly purified by flash column chromatography on KP-SIL (hexane / AcOEt = 4 / 1). The resulting crude product was dissolved in n-butanol (25 mL). To the mixture was added hydrazine (3.6 mL), and stirred at 90 ˚C for 1 h under argon atmosphere. To the mixture was added water at 0 ˚C, and concentrated. The residue was diluted with AcOEt, and washed with 1N HCl. The organic layer was dried over MgSO4 and concentrated. The residue was roughly purified by flash column chromatography on KP-SIL (hexane / AcOEt = 4 / 1). The resulting crude product was dissolved in benzene / MeOH = 9 / 1 (5 mL). To the mixture was added TMSCHN2 (1.1 mL, 0.64 mmol), and stirred at rt for 15 min. The resulting mixture was concentrated to give 20 (269 mg, 34%) as colorless oil. 1H NMR (400 MHz, CDCl3) δ 7.32-7.16 (m, 5 H), 5.64 (brs, 1 H), 3.78-3.74 (m, 3 H), 3.10-0.80 (m, 32 H), 0.57-0.51 (m, 3 H).
5-[2-(4-Bromomethylene-7a-methyloctahydroinden-1-yl)propyl]-3-ethyl-3-hydroxy-1-phenethyl-
pyrrolidin-2-one (22). To a suspension of 19 (269 mg, 0.50 mmol) in MeCN / H2O = 7 / 1 (8 mL) was added Mo(CO)6 (205 mg, 0.78 mmol), and the mixture was stirred at 90 ˚C for 12 h under argon atmosphere. The resulting mixture was filtered over celite and concentrated. The residue was purified by flash column chromatography on KP-SIL (hexane / AcOEt = 2 / 1) to give 22 (132 mg, 53%) as colorless oil. 1H NMR (400 MHz, CDCl3) δ 7.33-7.16 (m, 5 H), 5.66 (brs, 1 H), 3.85-3.73 (m, 1 H), 3.55-3.46 (m, 1 H), 3.32-3.16 (m, 1 H), 2.94-2.64 (m, 3 H), 2.28-0.82 (m, 24 H), 0.61-0.56 (m, 3 H).
5-[2-(4-Bromomethylene-7a-methyloctahydroinden-1-yl)propyl]-3-propyl-3-hydroxy-1-phenethyl-
pyrrolidin-2-one (23). Similar to the synthesis of 22, a crude product, which was obtained from 20 (307 mg, 0.56 mmol) and Mo(CO)6 (230 mg, 0.87 mmol) in CH3CN / H2O = 7 / 1 (8 mL) at 90 ˚C for 12 h, was purified by preparative TLC (silica gel; hexane / AcOEt = 1/ 1) to give 23 as colorless oil (171 mg, 59%). 1H NMR (400 MHz, CDCl3) δ 7.33-7.17 (m, 5 H), 5.66 (brs, 1 H), 3.84-3.72 (m, 1 H), 3.55-3.47 (m, 1 H), 3.25-3.15 (m, 1 H), 2.95-2.73 (m, 3 H), 2.29-0.83 (m, 26 H), 0.60-0.55 (m, 3 H).
5-[2-(4-Bromomethylene-7a-methyloctahydroinden-1-yl)propyl]-3-ethyl-1-phenethyl-3-trimethyl-
silanyloxypyrrolidin-2-one (25). To a suspension of 22 (132 mg, 0.26 mmol) in CH2Cl2 (5 mL) was added trimethylsilyl-imidazole (0.2 mL, 1.30 mmol), and the mixture was stirred at rt for 12 h under argon atmosphere. To the resulting mixture was added water at 0 ˚C. The aqueous layer was extracted with CH2Cl2. The organic layer was dried over MgSO4 and concentrated. The residue was purified by preparative TLC (silica gel; hexane / AcOEt = 5 / 1) to give 25 as colorless oil (133 mg, 89%). 1H NMR (400 MHz, CDCl3) δ 7.33-7.16 (m, 5 H), 5.68-5.64 (m, 1 H), 3.77-3.50 (m, 2 H), 3.28-3.10 (m, 1 H), 2.92-2.75 (m, 3 H), 2.23-0.75 (m, 24 H), 0.58 (d, J = 4.6 Hz, 3 H), 0.16-0.10 (m, 9 H).
5-[2-(4-Bromomethylene-7a-methyloctahydroinden-1-yl)propyl]-3-propyl-1-phenethyl-3-trimethyl-
silanyloxypyrrolidin-2-one (26). Similar to the synthesis of 25, a crude product, which was obtained from 23 (171 mg, 0.33 mmol) and trimethylsilylimidazole (0.25 mL, 1.65 mmol) in CH2Cl2 (5 mL) at rt for 12 h, was purified by preparative TLC (silica gel; hexane / AcOEt = 5 / 1) to give 26 as colorless oil (174 mg, 90%). 1H NMR (400 MHz, CDCl3) δ 7.33-7.17 (m, 5 H), 5.68-5.64 (m, 1 H), 3.76-3.52 (m, 2 H), 3.26-3.10 (m, 1 H), 2.92-2.73 (m, 3 H), 2.23-0.86 (m, 26 H), 0.58 (d, J = 5.1 Hz, 3 H), 0.15-0.11 (m, 9 H).
3-Benzyl-5-[2-(4-bromomethylene-7a-methyloctahydroinden-1-yl)propyl]-3-hydroxy-1-phenethyl-
pyrrolidin-2-one (24). The mixture of 13 (240 mg, 0.93 mmol) and 2-benzylacrylic acid methyl ester
1812 (530 mg, 3.00 mmol) in toluene (5 mL) was stirred at 90 ˚C for 12 h under argon atmosphere. The resulting mixture was concentrated. The residue was roughly purified by flash column chromatography on KP-SIL (hexane / AcOEt = 20 / 1). The crude product was dissolved in MeCN / H2O = 7 / 1 (12 mL). To the resulting mixture was added Mo(CO)6 (240 mg, 0.91 mmol), and the mixture was stirred at 90 ˚C for 12 h under argon atmosphere. The resulting mixture was filtered over celite and concentrated. The residue was purified by flash column chromatography on KP-SIL (hexane / AcOEt = 2 / 1) to give 24 (178 mg, 2steps 53%) as an amorphous solid. 1H NMR (400 MHz, CDCl3) δ 7.31-7.18 (m, 10 H), 5.65 (s, 1 H), 3.80-3.68 (m, 1 H), 3.44-3.43 (m, 1 H), 3.20-3.16 (m, 1 H), 3.05-2.73 (m, 5 H), 2.17-1.00 (m, 16 H), 0.86-0.82 (m, 3 H), 0.54-0.50 (m, 3 H).
3-Benzyl-5-[2-(4-bromomethylene-7a-methyloctahydroinden-1-yl)propyl]-1-phenethyl-3-trimethyl-
silanyloxypyrrolidin-2-one (27). To the solution of 24 (178 mg, 0.32 mmol) in CH2Cl2 (3.5 mL) was added TMSOTf (70 µL, 0.39 mmol) and 2,6-lutidine (55 µL, 0.47 mmol). The resulting mixture was stirred at 0 ˚C for 1 h under argon atmosphere. To the mixture was added saturated NaHCO3 aq. at 0 ˚C, and the aqueous layer was extracted with CH2Cl2. The organic layer was dried over MgSO4 and concentrated. The residue was purified by flash column chromatography on KP-SIL (hexane / AcOEt = 20 / 1) to give 27 (190 mg, 95%) as colorless oil. 1H NMR (400 MHz, CDCl3) δ 7.33-7.15 (m, 10 H), 5.65 (brs, 1 H), 3.75-3.44 (m, 2 H), 3.30-3.18 (m, 1 H), 3.14-2.54 (m, 5 H), 2.12-1.02 (m, 16 H), 0.94-0.82 (m, 3 H), 0.55-0.43 (m, 3 H), 0.26-0.15 (m, 9 H).
20(R)-(3-Ethyl-3-hydroxy-1-phenethylpyrrolidin-2-one-5-yl)methyl-9,10-secopregna-5(Z),7(E),10(19)-triene-1α,3β-diol (9). The mixture of Pd2(dba)3CHCl3 (23.0 mg, 0.023 mmol) and PPh3 (47.0 mg, 0.18 mmol) in toluene / Et3N = 1 / 1 (3.6 mL) was stirred at rt for 10 min under argon atmosphere. To the resulting mixture was added the solution of 25 (87.0 mg, 0.15 mmol) and 2814 (66.0 mg, 0.18 mmol) in toluene (1.8 mL), and refluxed at 120 ˚C for 1 h under argon atmosphere. The resulting mixture was diluted with AcOEt, then added saturated NH4Cl aq. at rt. The aqueous layer was extracted with AcOEt. The combined organic layer was washed with brine, dried over MgSO4 and concentrated. The residue was roughly purified by preparative TLC (silica gel; hexane / AcOEt = 5 / 1). The crude product was dissolved in THF (3 mL). To the resulting solution was added the 1 M solution of TBAF in THF (0.44 mL, 0.44 mmol), and stirred at 50 ˚C for 2 h under argon atmosphere. To the resulting mixture was added saturated NH4Cl aq. at rt. The aqueous layer was extracted with AcOEt. The combined organic layer was washed with brine, dried over MgSO4 and concentrated. The residue was roughly purified by preparative TLC (silica gel; hexane / acetone = 1 / 1) to give 9 as three diastereomers mixtures. These mixtures were further purified by HPLC three times [first; CHIRALPAK AD column, φ 20 x 250 mm, DAICEL, hexane / EtOH = 7 / 3, second; ODS-AM column, φ 30 x 250 mm, YMC, eluent A / B = 3 / 7 (Eluent A; H2O / MeCN = 95 / 5, eluent B; MeCN / MeOH = 6 / 4 + 0.5% H2O), third; CHIRALPAK AD column, φ 20 x 250 mm, DAICEL, hexane / EtOH = 85 / 15] to give 9a (1.2 mg, 2%), 9b (2.2 mg, 3%) and 9d (0.6 mg, 1%), respectively.
Spectral data for (23S,25S)-DLAM-2P-25Et (9a):16 1H NMR (400 MHz, CDCl3) δ 7.33-7.18 (m, 5 H),
6.38 (d, J = 11.5 Hz, 1 H), 6.02 (d, J = 11.0 Hz, 1 H), 5.33 (s, 1 H), 5.00 (s, 1 H), 4.43 (brs, 1 H), 4.23 (brs, 1 H), 3.80-3.70 (m, 1 H), 3.55-3.45 (m, 1 H), 3.25-3.15 (m, 1 H), 2.93-2.75 (m, 3 H), 2.63-2.55 (m, 1 H), 2.32 (dd, J = 13.7, 6.7 Hz, 1 H), 2.11 (dd, J = 13.7, 7.3 Hz, 1 H), 2.04-1.10 (m, 22 H), 0.96 (t, J = 7.4 Hz, 3 H), 0.88 (d, J = 5.6 Hz, 3 H), 0.56 (s, 3 H); HRMS calcd for C36H51NO4 562.3891, found 562.3915.
Spectral data for (23R,25R)-DLAM-2P-25Et (9b):16 1H NMR (400 MHz, CDCl3) δ 7.34-7.19 (m, 5 H), 6.38 (d, J = 11.2 Hz, 1 H), 6.03 (d, J = 11.2 Hz, 1 H), 5.34 (s, 1 H), 5.01 (s, 1 H), 4.44 (brs, 1 H), 4.25 (brs, 1 H), 3.85-3.77 (m, 1 H), 3.55-3.47 (m, 1 H), 3.25-3.15 (m, 1 H), 2.95-2.74 (m, 3 H), 2.61 (dd, J = 13.7, 3.4 Hz, 1 H), 2.32 (dd, J = 13.4, 6.7 Hz, 1 H), 2.20 (dd, J = 13.5, 7.7 Hz, 1 H), 2.12-1.15 (m, 22 H), 1.05-0.91 (m, 6 H), 0.56 (s, 3 H); HRMS calcd for C36H51NO4 562.3891, found 562.3905.
Spectral data for (23R,25S)-DLAM-2P-25Et (9d):16 1H NMR (400 MHz, CDCl3) δ 7.32-7.16 (m, 5 H), 6.38 (d, J = 11.0 Hz, 1 H), 6.03 (d, J = 11.2 Hz, 1 H), 5.34 (s, 1 H), 5.01 (s, 1 H), 4.44 (brs, 1 H), 4.24 (brs, 1 H), 4.06-3.95 (m, 1 H), 3.35-3.27 (m, 1 H), 3.24-3.14 (m, 1 H), 2.95-2.69 (m, 3 H), 2.64-2.57 (m, 1 H), 2.42 (s, 1 H), 2.36-2.23 (m, 2 H), 2.04-1.06 (m, 19 H), 1.00 (d, J = 6.3 Hz, 3 H), 0.85 (t, J = 7.4 Hz, 3 H), 0.58 (s, 3 H); HRMS calcd for C36H51NO4 562.3891, found 562.3921.
20(R)-(3-Hydroxy-1-phenethyl-3-propylpyrrolidin-2-one-5-yl)methyl-9,10-secopregna-5(Z),7(E),10(19)-triene-1α,3β-diol (10). Similar to the synthesis of 9, a crude product, which was obtained from the solution of Pd2(dba)3CHCl3 (23.0 mg, 0.023 mmol) and PPh3 (47.0 mg, 0.18 mmol) in toluene / Et3N = 1 / 1 (3.6 mL), and the solution of 26 (86.9 mg, 0.15 mmol) and 2814 (66.0 mg, 0.18 mmol) in toluene (1.8 mL) at 120 ˚C for 1 h, was treated with 1 M TBAF in THF (0.56 mL, 0.56 mmol) at 50 ˚C for 2 h. After usual work up, the crude product was roughly purified by preparative TLC (silica gel; hexane / acetone = 1 / 1) to give 10 as four diastereomers mixtures. These mixtures were further purified by HPLC twice [first; ODS-AM column, φ 30 x 250 mm, YMC, Eluent A / B = 3 / 7 (eluent A; H2O / MeCN = 95 / 5, eluent B; MeCN / MeOH = 6 / 4 + 0.5% H2O), second; CHIRALPAK AD column, φ 20 x 250 mm, DAICEL, hexane / EtOH = 7 / 3] to give 10a (4.5 mg, 5%), 10b (5.1 mg, 6%), 10c (1.6 mg, 2%), and 10d (2.7 mg, 3%) respectively.
Spectral data for (23S,25S)-DLAM-2P-25nPr (10a):16 1H NMR (400 MHz, CDCl3) δ 7.33-7.18 (m, 5 H), 6.38 (d, J = 11.0 Hz, 1 H), 6.02 (d, J = 11.2 Hz, 1 H), 5.33 (s, 1 H), 5.00 (s, 1 H), 4.43 (brs, 1 H), 4.23 (brs, 1 H), 3.81-3.70 (m, 1 H), 3.55-3.45 (m, 1 H), 3.25-3.16 (m, 1 H), 2.93-2.75 (m, 3 H), 2.63-2.56 (m, 1 H), 2.32 (dd, J = 13.4, 6.6 Hz, 1 H), 2.20-1.10 (m, 25 H), 0.96 (t, J = 7.0 Hz, 3 H), 0.88 (d, J = 5.6 Hz, 3 H), 0.56 (s, 3 H); HRMS calcd for C37H53NO4 576.4047, found 576.4054.
Spectral data for (23R,25R)-DLAM-2P-25nPr (10b):16 1H NMR (400 MHz, CDCl3) δ 7.34-7.19 (m, 5 H), 6.38 (d, J = 11.2 Hz, 1 H), 6.03 (d, J = 11.2 Hz, 1 H), 5.34 (s, 1 H), 5.01 (s, 1 H), 4.44 (brs, 1 H), 4.24 (brs, 1 H), 3.85-3.75 (m, 1 H), 3.54-3.46 (m, 1 H), 3.25-3.14 (m, 1 H), 2.94-2.72 (m, 3 H), 2.61 (dd, J = 13.5, 3.3 Hz, 1 H), 2.32 (dd, J = 13.4, 6.7 Hz, 1 H), 2.25-2.15 (m, 2 H), 2.06-1.11 (m, 23 H), 1.00-0.93 (m, 6 H), 0.56 (s, 3 H); HRMS calcd for C37H53NO4 576.4047, found 576.4069.
Spectral data for (23S,25R)-DLAM-2P-25nPr (10c):16 1H NMR (400 MHz, CDCl3) δ 7.32-7.17 (m, 5 H), 6.37 (d, J = 11.2 Hz, 1 H), 6.01 (d, J = 11.5 Hz, 1 H), 5.33 (s, 1 H), 5.00 (s, 1 H), 4.43 (brs, 1 H), 4.23 (brs, 1 H), 4.06-3.96 (m, 1 H), 3.33-3.25 (m, 1 H), 3.22-3.13 (m, 1 H), 2.93-2.70 (m, 3 H), 2.64-2.56 (m, 1 H), 2.49 (s, 1 H), 2.32 (dd, J = 13.3, 7.0 Hz, 1 H), 2.21 (dd, J = 13.1, 6.4 Hz, 1 H), 2.04-1.10 (m, 23 H), 0.91 (d, J = 6.3 Hz, 3 H), 0.86 (t, J = 7.0 Hz, 3 H), 0.55 (s, 3 H); HRMS calcd for C37H53NO4 576.4047, found 576.4072.
Spectral data for (23R,25S)-DLAM-2P-25nPr (10d):16 1H NMR (400 MHz, CDCl3) δ 7.31-7.15 (m, 5 H), 6.38 (d, J = 11.0 Hz, 1 H), 6.03 (d, J = 11.2 Hz, 1 H), 5.34 (s, 1 H), 5.01 (s, 1 H), 4.44 (brs, 1 H), 4.24 (brs, 1 H), 4.07-3.97 (m, 1 H), 3.35-3.28 (m, 1 H), 3.23-3.14 (m, 1 H), 2.95-2.69 (m, 3 H), 2.64-2.57 (m, 1 H), 2.49 (s, 1 H), 2.36-2.23 (m, 2 H), 2.06-1.05 (m, 23 H), 1.00 (d, J = 6.6 Hz, 3 H), 0.87 (t, J = 7.0 Hz, 3 H), 0.58 (s, 3 H); HRMS calcd for C37H53NO4 576.4047, found 576.4080.
20(R)-(3-Benzyl-3-hydroxy-1-phenethylpyrrolidin-2-one-5-yl)methyl-9,10-secopregna-5(Z),7(E),10(19)-triene-1α,3β-diol (11). Similar to the synthesis of 9, a crude product, which was obtained from the solution of Pd2(dba)3CHCl3 (11.0 mg, 0.011 mmol) and PPh3 (22.0 mg, 0.084 mmol) in toluene / Et3N = 1 / 1 (2 mL), and the solution of 27 (45.0 mg, 0.07 mmol) and 2814 (31.0 mg, 0.084 mmol) in toluene (1 mL) at 120 ˚C for 2 h, was treated with 1 M TBAF in THF (0.14 mL, 0.14 mmol) at 50 ˚C for 4 h. After usual work up, the crude product was roughly purified by preparative TLC (silica gel; hexane / acetone = 1 / 1) to give 11 as two diastereomers mixtures. These mixtures were further purified by HPLC twice [first; CHIRALPAK AD column, φ 20 x 250 mm, DAICEL, hexane / EtOH = 8 / 2, second; ODS-AM column, φ 30 x 250 mm, YMC, eluent A / B = 25 / 75 (eluent A; H2O / MeCN = 95 / 5, eluent B; MeCN / MeOH = 6 / 4 + 0.5% H2O)] to give 11a (3.0 mg, 4%) and 11b (4.4 mg, 5%).
Spectral data for (23S,25S)-DLAM-2P-25Bn (11a):16 1H NMR (400 MHz, CDCl3) δ 7.32-7.17 (m, 10 H), 6.38 (d, J = 11.2 Hz, 1 H), 6.02 (d, J = 11.2 Hz, 1 H), 5.35 (s, 1 H), 5.01 (s, 1 H), 4.44 (brs, 1 H), 4.24 (brs, 1 H), 3.76-3.66 (m, 1 H), 3.50-3.40 (m, 1 H), 3.24-3.16 (m, 1 H), 3.05-2.58 (m, 6 H), 2.62-2.59 (m, 1H), 2.37 (s, 1H), 2.32 (dd, J = 13.4, 6.6 Hz, 1H), 2.38-1.12 (m, 19 H), 0.80 (d, J = 5.1 Hz, 3 H), 0.51 (s, 3 H); HRMS calcd for C41H53NO4 624.4047, found 624.4072.
Spectral data for (23R,25R)-DLAM-2P-25Bn (11b):16 1H NMR (400 MHz, CDCl3) δ 7.32-7.18 (m, 10 H), 6.37 (d, J = 11.2 Hz, 1 H), 6.01 (d, J = 11.0 Hz, 1 H), 5.34 (s, 1 H), 5.00 (s, 1 H), 4.44 (brs, 1 H), 4.23 (brs, 1 H), 3.80-3.69 (m, 1 H), 3.47-3.38 (m, 1 H), 3.23-3.12 (m, 1 H), 3.05-2.70 (m, 6 H), 2.64-2.55 (m,
1 H), 2.45 (s, 1 H), 2.32 (dd, J = 13.4, 6.6 Hz, 1H), 2.15-1.05 (m, 19 H), 0.84 (d, J = 6.6 Hz, 3 H), 0.51 (s,
3 H); HRMS calcd for C41H53NO4 624.4047, found 624.4077.
Vitamin D receptor (VDR) binding assay17
[26,27-Methyl-3H]-1α,25-dihydroxyvitamin D3 (180 Ci/ mmol, 15000 dpm) and various amounts of DLAM analogs to be tested were dissolved in 50 µL of absolute ethanol in 12 x 75 mm polypropylene tubes. The chick intestinal VDR (0.2 mg) and 1 mg of gelatin in 1 mL of phosphate buffer solution (pH 7.4) were added to each tube in an ice bath. The assay tubes were incubated in shaking water bath for 1 h at 25 ˚C and then chilled in an ice bath. Polypropylene glycol 6000 (40%, 1 mL) in distilled water was added to each tube, and the tubes were mixed vigorously and centrifuged ant 2260 x g for 60 min at 4 ˚C. The resulting pellet was cut off into a scintillation vial containing 10 mL of dioxane-based scintillation fluid and the radioactivity was counted with Beckman liquid scintillation counter (model LC6500). The relative potency of the analogs were calculated from their concentration needed to displace 50% of [26,27-Methyl-3H]-1α,25-dihydroxyvitamin D3 from the receptor compared with the activity of 1α,25-dihydroxyvitamin D3 (assigned a 100% value).
Assay for HL-60 cell differentiation: The human promyelocytic leukemia cell line HL-60 was purchased from a cell bank (Japanese Cancer Research Resources Bank, cell#: JCRB0085). The HL-60 cells were cultured in RPMI1640 medium supplemented with 10% FBS. The cell concentration at seeding was adjusted to 2 x 104 cells / mL, and the seeding volume was 1 mL / well. To assess the vitamin D3-antagonistic activities of test compounds, the HL-60 cells were incubated with various concentrations (1 x 10-4 M to 3 x 10-3 M) of a test compound (added to the culture in 1 µL of ethanol solution) in the presence of 1 x 10-5 M 1α,25-dihydroxyvitamin D3 (added to the culture in 1 µL of ethanol solution) for 96 h at 37 ˚C in a humidified atmosphere of 5% CO2/air without a medium change. The same amount of vehicle was added to the control culture. NBT-reducing assay was performed according to the method of Collins.18 Briefly, cells were collected, washed with PBS, and suspended in serum-free medium. NBT / TPA solution (dissolved in PBS) was added. Final concentrations of NBT and TPA were 0.1% and 100 ng / mL, respectively. Then, the cell suspensions were incubated at 37 ˚C for 25 min. After incubation, cells were collected by centrifugation and resuspended in FCS. Cytospin smears were prepared, the counter-staining of nuclei was done with Kemechrot solution, and the ratio of NBT-positive cells was counted under microscope.
ACKNOWLEDGEMENTS
The work described in this paper was supported by Grants-in-Aid for Scientific Research from the ministry of Education, Science, Sports and Culture, Japan, and the funds from the Mochida Memorial
Foundation for Medicinal and Pharmaceutical Research, and the TERUMO Life Science Foundation.
Reference 11
References
0. †This paper is dedicated to Professor Dr. Keiichiro Fukumoto on the occasion of his 75th birthday.
1. a) ‘Vitamin D, second ed.,’ ed. by D. Feldman, J. W. Pike, and F. H. Glorieux, Elsevier Academic Press, New York, 2005; b) ‘Vitamin D Physiology, Molecular Biology, and Clinical, Applications,’ ed. by M. F. Holick, Humana Press, Totowa, 1999; c) R. Bouillon, W. H. Okamura, and A. W. Norman, Endocr. Rev., 1995, 16, 200.
2. a) R. M. Evans, Cell, 1988, 240, 889; b) K. Umezono, K. K. Murakami, C. C. Thompson, and R. M. Evans, Cell, 1991, 65, 1255. CrossRef
3. K. Takeyama, Y. Masuhiro, H. Fuse, H. Endoh, A. Murayama, S. Kitanaka, M. Suzuwa, J. Yanagisawa, and S. Kato, Mol. Cell. Biol., 1999, 19, 1049.
4. a) M. Herdick, A. Steinmeyer, and C. Carlberg, Chem. Biol., 2000, 7, 885; CrossRef b) Y. Bury, A. Steinmeyer, and C. Carlberg, Mol. Pharmacol., 2000, 58, 1067; c) A. Toell, M. M. Gonzalez, D. Ruf, A. Steinmeyer, S. Ishizuka, and C. Carlberg, Mol. Pharmacol., 2001, 59, 1478; d) S. Väisänen, M. Peräkylä, A. Kärkkäinen, A. Steinmeyer, and C. Carlberg, J. Mol. Biol., 2002, 315, 229. CrossRef
5. a) D. Miura, K. Manabe, K. Ozono, M. Saito, Q. Gao, A. W. Norman, and S. Ishizuka, J. Biol. Chem., 1999, 274, 16392; CrossRef b) K. Ozono, M. Saito, D. Miura, T. Michigami, S. Nakajima, and S. Ishizuka, J. Biol. Chem., 1999, 274, 32376; CrossRef c) C. M. Bula, J. E. Bishop, S. Ishizuka, and A. W. Norman, Mol. Endcrinol. 2000, 11, 1788; CrossRef d) S. Ishizuka, D. Miura, K. Ozono, M. Chokki, H. Mimura, and A. W. Norman, Endcrinology, 2001, 142, 59; CrossRef e) K. Takenouchi, R. Sogawa, K. Manabe, H. saitoh, Q. Gao, D. Miura, and S. Ishizuka, J. Steroid. Biochem. Mol. Biol., 2004, 89-90, 31; CrossRef f) S. Ishizuka, N. Kurihara, D. Miura, K. Takenouchi, J. Cornish, T. Cundy, S. V. Reddy, and G. D. Roodman, J. Steroid. Biochem. Mol. Biol., 2004, 89-90, 331; CrossRef g) S. Saito, M. Masuda, T. Matsunaga, H. Saito, M. Anzai, K. Takenouchi, D. Miura, S. Ishizuka, M. Takimoto-Kamimura, and A. Kittaka, Tetrahedron, 2004, 60, 7951. CrossRef
6. a) K. Yamamoto, D. Abe, N. Yoshimoto, M. Choi, K. Yamagishi, H. Tokiwa, M. Shimizu, M. Makishima, and S. Yamada, J. Med. Chem., 2006, 49, 1313; CrossRef b) Y. Inaba, K. Yamamoto, N. Yoshimoto, M. Matsunawa, S. Uno, S. Yamada, and M. Makishima, Mol. Pharmacol., 2007, 71, 1298. CrossRef
7. a) Y. Kato, Y. Hashimoto, and K. Nagasawa, Molecules, 2003, 8, 488; CrossRef b) Y. Kato, Y. Nakano, H. Sano, A. Tanatani, H. Kobayashi, R. Shimazawa, H. Koshino, Y. Hashimoto, and K. Nagasawa, Bioorg. Med. Chem. Lett., 2004, 14, 2579; CrossRef c) Y. Nakano, Y. Kato, K. Imai, E. Ochiai, J. Namekawa, S. Ishizuka, K. Takenouchi, A. Tanatani, Y. Hashimoto, and K. Nagasawa, J. Med. Chem., 2006, 49, 2398; CrossRef d) K. Cho, F. Uneuchi, Y. Kato-Nakamura, J. Namekawa, S. Ishizuka, K. Takenouchi, K. Nagasawa, Bioorg. Med. Chem. Lett., 2008, 18, 4287. CrossRef
8. a) C. Menaa, J. Barsony, S. V. Reddy, J. Cornish, T. Cundy, and G. D. Roodman, J. Bone. Miner. Res., 2000, 15, 228; CrossRef b) N. Kurihara, S. V. Reddy, C. Menaa, D. Anderson, and G. D. Roodman, J. Clin. Invest., 2000, 105, 607; CrossRef c) R. J. Leach, and G. D. Roodman, J. Clin. Endocrinol. Matab., 2001, 86, 24; CrossRef d) S. V. Reddy, N. Kurihara, C. Menaa, G. Landucci, D. Forthal, B. A. Koop, J. J. Windle, and G. D. Roodman, Endocrinology, 2001, 142, 2898; CrossRef e) W. E. Friedrichs, S. V. Reddy, J. M. Bruder, T. Cundy, J. Cornish, F. R. Singer, and G. D. Roodman, J. Bone. Miner. Res., 2002, 17, 145; CrossRef f) N. Kurihara, S. Ishizuka, A. Demulder, C. Menaa, and G. D. Roodman, J. Steroid. Biochem. Mol. Biol., 2004, 89-90, 321; CrossRef g) S. Ishizuka, N. Kurihara, D. Miura, K. Takenouchi, J. Cornish, T. Cundy, S. V. Reddy, and G. D. Roodman, J. Steroid. Biochem. Mol. Biol., 2004, 89-90, 331; CrossRef h) N. Kurihara, S. V. Reddy, N. Araki, S. Ishizuka, K. Ozono, J. Cornish, T. Cundy, F. R. Singer, and G. D. Roodman, J. Bone Miner. Res., 2004, 19, 1154; CrossRef i) S. Ishizuka, N. Kurihara, S. V. Reddy, J. Cornish, T. Cundy, and G. D. Roodman, Endocrinology, 2005, 146, 2023. CrossRef
9. a) T. Eguchi, H. Sai, S. Takatsuto, N. Hara, and N. Ikekawa, Chem. Pharm. Bull., 1988, 36, 2303; b) T. Eguchi, N. Ikekawa, K. Sumitani, M. Kumegawa, S. Higuchi, and S. Otomo, Chem. Pharm. Bull., 1990, 38, 1246; c) T. Miyahara, M. Harada, M. Miyata, A. Sugure, Y. Ikemoto, T. Takamura, S. Higuchi, S. Otomo, H. Kozuka, and N. Ikekawa, Calcif. Tissue Int., 1992, 51, 218; CrossRef d) N. Kubodera, H. Watanabe, T. Kawanishi, and M. Matsumoto, Chem. Pharm. Bull., 1992, 40, 1494; e) M. Harada, T. Takamura, S. Kajita-Kondo, A. Kozakai, T. Miyahara, H. Kozuka, N. Ikekawa, S. Higuchi, and S. Otomo, Calcif. Tissue Int., 1995, 56, 220; CrossRef f) Y. Ono, A. Kawase, H. Watanabe, A. Shiraishi, S. Takeda, Y. Higuchi, K. Sato, T. Yamauchi, T. Mikami, M. Kato, N. Tsugawa, T. Okano, and N. Kubodera, J. Med. Chem., 1998, 6, 2517. CrossRef
10. N. Saito, H. Saito, M. Anzai, A. Yoshida, T. Fujishima, K. Takenouchi, D. Miura, S. Ishizuka, H. Takayama, and A. Kittaka, Org. Lett., 2003, 5, 4859. CrossRef
11. We applied phthalimidomethyl ester as the protective group of the carboxyl group of 29 and 30 because the boiling points of the methyl ester of 29 and 30 are relatively low to handle.20,21 Synthesis of phtalimidoester 14 and 15 were shown in Scheme 2.
12. D. Basavaiah and N. Kumaragurubaran, Tetrahedron Lett., 2001, 42, 477. CrossRef
13. B. M. Trost, J. Dumas, and M. Villa, J. Am. Chem. Soc., 1992, 114, 9836. CrossRef
14. N. Saito, M. Masuda, H. Saito, K. Takenouchi, S. Ishizuka, J. Namekawa, M. Takimoto-Kamimura, and A. Kittaka, Synthesis, 2005, 15, 2533. CrossRef
15. We couldn’t get all four diastereomers of 9 and 11, though we obtained four diastereomers of 10. We presumed that the amount of the final product 9 was too small (9.8 mg) so that we lost one diastereomer during the HPLC separation process. In case of 11, only two diatereomers of 21 were generated during the 1,3-dipolarcycloaddition reaction of 13 and 18 because of the steric hinderance of the benzyl group in 18. Thus, two major isomers of 11a and 11b were obtained at the final separation process.
16. Stereochemistries at C23 and C25 of 9-11 were determined by comparison with the 1H NMR spectral data for DLAM-2P (6).7c The typical chemical shifts of each stereoisomers for 9-11 were underlined in their experiment sections.
17. S. Ishizuka, K. Bannai, T. Naruchi, and Y. Hashimoto, Steroids, 1981, 37, 33. CrossRef
18. S. J. Collins, F. W. Ruscetti, R. E. Gallagher, and R. C. Gallo, J. Exp. Med., 1979, 149, 969. CrossRef
19. N. Rochel, J. M. Wurtz, A. Mitschler, B. Klaholz, and D. Moras, Mol. Cell., 2000, 5, 173. CrossRef
20. R. Kimura, A. Ichihara, and S. Sakamura, Synthesis, 1979, 7, 516. CrossRef
21. B. Garkowik, Ukr. Khim. Zh., 1961, 27, 671.