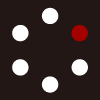
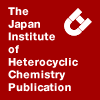
HETEROCYCLES
An International Journal for Reviews and Communications in Heterocyclic ChemistryWeb Edition ISSN: 1881-0942
Published online by The Japan Institute of Heterocyclic Chemistry
e-Journal
Full Text HTML
Received, 22nd July, 2008, Accepted, 28th August, 2008, Published online, 1st September, 2008.
DOI: 10.3987/COM-08-S(F)52
■ Facile Construction of Furan-fused Methano[11]annulenone Skeleton by Successive Aldol Condensations from 3,4-Furan-dicarbaldehydes
Yanmei Zhang, Nobuhiko Takezawa, oshikazu Horino, Ayumi Takai, Yasutomo Tsuji, Ryuta Mityatake, Mitsunori Oda,* Shigeyasu Kuroda*
Faculty of Engineering, Toyama University, Gofuku 3190, Toyama 930-8555, Japan
Abstract
Aldol condensation of furan-3,4-dicarbaldehyde with pentanedial in acetic acid gave 6H-cyclohepta[c]furan-5,7-dicarbaldehyde in a good yield. Then, successive condensation of 6H-cyclohepta[c]furan-5,7-dicarbaldehyde with dimethyl 1,3-acetonedicarboxylate under azeotropic conditions gave a diester derivative of furan-fused methano[11]annulenone also in a good yield. The hydrolysis of the diester groups and subsequent decarboxylation provided a non-substituted furan-fused methano[11]annulenone. A similar sequence starting from 2,5-dimethylfuran-3,4-dicarbaldehyde gave its dimethyl derivative. Cycloaddition and protonation of these furan-fused methano[11]annulenones were also studied.INTRODUCTION
Figure 1.Various furan-fused annulenes,1 including benzofuran as a typical example of [6]annulenofuran,2 have been synthesized from the viewpoints of their aromatic character and synthetic utility. Sondheimer et al. synthesized two types of furan-fused dehydroannulenes, 1 and 2, and reported that 1 has atropic nature for
the annulene ring based on its 1H NMR spectral data, whereas 2 is a diatropic compound.3,4 They also indicated that the furan ring part of 1 could be used as a foothold for producing a carbon framework through the annulene-1,2-dicarbaldehyde in the synthesis of annulenoannulenes.3 Annuleno[c]furan, a type of 1, also serves as a good enophile in Diels–Alder reactions. For example, Mitchell et al. reported that bridged [14]annuleno[c]furan 3 reacts various arynes to form cycloadducts, which were transformed to arene-annulated bridged [14]annulenes by subsequent deoxygenation.5 Meanwhile, such chemical behaviors of furan-fused annulenediones aroused our interest and we reported the synthesis and physical properties of 4.6 During the course of our continuing research on bridged annulenes,7 we have also been interested in annulenoannulenones, towards which an effective short-step synthesis of furan-fused annulenones as a synthetic intermediate is required. Herein we disclose a facile method for synthesizing such an annulenone, 5,11-methano-8H-[11]annuleno[c]furan-8-one (5).
RESULTS AND DISCUSSION
The straightforward route to the title compound 5 is shown in Scheme 1. Furan-3,4-dicarbaldehyde (6) reacts with pentanedial in the presence of piperidine in acetic acid at 120 °C for 4 h to give 6H-cyclohepta[c]furan-5,7-dicarbaldehyde (7) in 68% yield. The synthesis of 7 under similar reaction conditions in the presence of piperidine in refluxing acetic acid had been reported by Lepage et al.8 However, the reported yield was as low as 22%. After extensive work on the reaction conditions, we found that the yield could be greatly improved by modifying the reaction conditions. It is worth noting that a short reaction time and using hydrated pentanedial instead of 2-methoxy-2,3,4-trihydropyrane are crucial for the improvement of the yield and that low substrate concentration and flash chromatography of the product are important to gain a constant yield. Having a large quantity of 7 available, we next examined the aldol condensation of 7 with dimethyl 1,3-acetonedicarboxylate to construct the carbon framework of the desired 5. Although yields of similar condensations between arene-1,2-dicarbaldehyde and dimethyl 1,3-acetonedicarboxylate are moderate to good,9 those of the 1,3-carbaldehyde are usually less than 50%.10 After several reported reaction conditions were applied for the condensation of 7 to result in disappointing yields of 8,11 it was found that under azeotropic conditions with a Dean–Stark apparatus,
8 was produced in 69% yield. The structure of 8 was confirmed by spectroscopic analysis and also by X-ray crystallographic analysis (Figure 1).12 The hydrolysis of 8 yielded the corresponding carboxylic acid, which was decarboxylated in the presence of copper powder at 120 °C in DMF to give the annulenone 5 as yellow oil in 73% yield. Thus, the facile and efficient construction of furan-fused [11]annulenone was accomplished. Using 2,5-dimethylfuran-3,4-dicarbaldehyde as a starting material, application of this protocol for synthesizing a derivative of 5 provided its 1,3-dimethyl derivative, 9, in 20% total yield.11
The title compound 5 was isolated as slightly acid- and air-sensitive yellow oil. Protonation of 5 in an acidic medium at room temperature resulted in decomposition of 5, whereas the 4,9-methano[11]annulenone (10) and furo[c]tropone 11 were stable under similar conditions. Although monitoring a solution of 5 in CF3CO2D at –15 °C by 1H NMR spectroscopy revealed the complete decomposition of 5 in 4 h, the transient protonated species 5D+ could be observed (Scheme 2). The olefinic protons of 5D+ resonate at a lower magnetic field by 1.10 ppm on average and the bridging methylene protons resonate at a higher magnetic field by 0.61 ppm on average, compared with those in CDCl3 solution. At a glance, these shifts appear to indicate a deshielding effect on the olefinic protons and a shielding effect on the bridging methylene protons based on the diamagnetic ring current induced by the peripheral 14π-electorn aromatic system in the protonated species. Since the magnitude of these shifts is less than those observed in 10 upon protonation, the protonated species 5D+ is thought to be weakly diatropic. In other words, the contribution of the peripheral 14π-electorn aromatic system in the protonated species is very small.
The furan ring of [11]annulenones, 5 and 8, serves as a 4π-component for the Diels–Alder reaction. On heating 5 and 8 with dimethyl acetylenedicarboxylate (DMAD) in toluene, cycloadducts 12 and 13 were obtained in 87% and 89% yields, respectively. The structure of 13 was determined by X-ray crystallography analysis (Figure 2) to reveal the syn-configuration between oxa- and methano-bridges in the product. The stereochemistry of 12 was deduced to have the same configuration as 13 by comparison of the spectroscopic data of 12 and 13. Thus, in these reactions, DMAD approaches the furan ring from the rear side of the methylene bridge.
In summary, the facile and efficient synthesis of furan-fused [11]annulenones has been accomplished by a four-step sequence starting from furan-dicarbaldehydes. The 11-membered ring of the protonated species of [11]annulenone 5 is weakly diatropic in nature. The furan ring of annulenones 5 and 8 indicated sufficient reactivity for DMAD. Such reactivity of these compounds gives the prospect of constructing a new framework towards annulenoannulenones by cycloaddition with suitable arynes. Further transformation of 5 along these lines is now in progress.
This paper is dedicated to Professor Emeritus Keiichiro Fukumoto on the occasion of his 75th birthday
References
1. A. T. Balaban, M. Banciu, and V. Ciorba, ‘Annulenes, Benzo-, Hetero-, Homo-Derivatives and their Valence Isomers,’ Vol I-III, CRC Press, Boca Raton, 1987; M. Nakagawa, ‘The Chemistry of Annulenes,’Osaka University Press, Osaka, 1996.
2. M. J. Haddadin, Heterocycles, 1978, 9, 865; CrossRef U. E. Wiersum, Aldrichimica Acta, 1981, 14, 53.
3. T. M. Cresp and F. Sondheimer, J. Am. Chem. Soc., 1975, 97, 4412; CrossRef T. M. Cresp and F. Sondheimer, J. Am. Chem. Soc., 1977, 99, 194. CrossRef
4. R. R. Jones, J. M. Brown, and F. Sondheimer, Tetrahedron Lett., 1975, 16, 4179; CrossRef R. H. Wightman and F. Sondheimer, Tetrahedron Lett., 1975, 16, 4183. CrossRef
5. R. H. Mitchell, V. S. Iyer, N. Khalifa, R. Mahadevan, S. Venugopalan, S. A. Weerawarna, and P. Zhou, J. Am. Chem. Soc., 1995, 117, 1514; CrossRef R. H. Mitchell and T. R. Ward, Tetrahedron., 2001, 57, 3689. CrossRef
6. S. Zuo, S. Kuroda, M. Oda, S. Kuramoto, Y. Mizukami, A. Fukuta, Y. Hirano, T. Nishikawa, S. Furuta, R. Miyatake, S. I. Shaheen, T. Kajioka, and M. Kyougoku, Heterocycles, 2001, 54, 159. CrossRef
7. S. Kuroda, T. Kajioka, A. Fukuta, N. C. Thanh, Y. Zhang, R. Miyatake, M. Mouri, S. Zuo, and M. Oda, Mini-Rev. Org. Chem., 2007, 4, 31. CrossRef
8. L. Lepage and Y. Lepage, Bull. Soc. Chim. Fr.,1988, 591.
9. D. S. Tarbell and B. Wargotz, J. Am. Chem. Soc., 1954, 76, 5761; CrossRef W. Ried and H. Bodem, Chem. Ber., 1956, 89, 708; CrossRef M. J. Cook and E. J. Forbes, Tetrahedron, 1968, 24, 4501; CrossRef B. Foehlisch, Synthesis, 1972, 564; CrossRef F. Pietra, Chem. Rev., 1973, 73, 293; CrossRef Also, for other recent results in this kind of condensations, see followings; P. Langer and U. Albrecht, Synlett, 2001, 526; CrossRef U. Albrecht, T. H. V Nguyen, and P. Langer, J. Org. Chem., 2004, 69, 3417. CrossRef
10. H. Ogawa, H. Kato, and M. Yoshida, Tetrahedron Lett., 1971, 12, 1793; CrossRef H. Ogawa and N. Shimojo, Tetrahedron Lett., 1972, 13, 4129; CrossRef H. Ogawa, N. Shimojo, and H. Kato, Tetrahedron, 1973, 29, 533; CrossRef W. Wagemann, K. Müllen, E. Vogel, T. Pilati, and M. Simonetta, Angew. Chem., Int. Ed. Engl., 1977, 16, 170. CrossRef
11. Selected data of new compounds are as follows; 8: Yellow crystals, mp 198-200 °C, 1H-NMR (CDCl3) δ = 2.22 (d, J =14.0 Hz, 1H), 2.78 (d, J = 14.0 Hz, 1H), 3.83 (s, 6H), 7.01 (s, 2H), 7.80 (s, 2H), 7.95 (s, 2H) ppm; 13C-NMR (CDCl3) δ = 30.2, 52.7, 123.2, 125.5, 128.8, 131.5, 141.5, 141.9, 164.9, 196.0 ppm; IR (KBr) νmax = 1704s, 1685m, 1510m, 1435m, 1254s, 1190m cm-1; UV (MeOH) λmax = 217 (logε = 3.99), 242 (4.18), 302 (4.67), 377sh (3.47) nm; HRMS: Calcd for C18H14O6 326.0790; Found 326.0781. 5: yellow oil, 1H-NMR (CDCl3) δ = 2.18 (d, J = 13.2 Hz, 1H), 2.53 (d, J = 13.2 Hz, 1H), 6.05 (dm as a part of AA’BB’, J = 12.0 Hz , 2H), 6.80 (s, 2 H), 6.96 (dm as a part of AA’BB’, J = 12.0 Hz, 2H), 7.90 (s, 2H) ppm; 13C-NMR (CDCl3) δ = 32.4, 119.5, 123.4, 127.8, 133.9, 137.3, 140.8, 196.2 ppm; IR (KBr) νmax = 2926s, 2854m, 1745m, 1608m, 1149m, 1089m cm-1; UV (MeOH) λmax = 229 (logε =3.92), 292 (3.90), 346sh (3.47), 410sh (3.03) nm; HRMS: Calcd for C14H10O2 210.0681; Found 210.0649.
7,9-Bis(methoxycarbonyl)-1,3-dimethyl-5,11-methano[c]furan-8-one: Yellow crystals, mp 213-215 °C, 1H-NMR (CD2Cl2) δ = 2.28 (d, J =14.0 Hz, 1H), 2.45 (s, 6H), 2.76 (d, J = 14.0 Hz, 1H), 3.75 (s, 6H), 6.86 (s, 2H), 7.72 (s, 2H) ppm; 13C-NMR (CD2Cl2) δ = 12.4, 30.7, 52.3, 119.4, 127.3, 128.5, 130.8, 141.5, 149.8, 165.0, 196.0 ppm; IR (KBr) νmax = 1713s, 1660m, 1580m, 1434m, 1265s, 1245s, 1216s, 766w cm-1; UV (MeOH) λmax = 254 (logε = 4.21), 318 (4.54), 376 (4.96) nm; 9: Yellow crystals, mp 95-97 °C, 1H-NMR (CDCl3) δ = 2.28 (d, J =12.4 Hz, 1H), 2.48 (s, 6H), 2.58 (d, J = 12.4 Hz, 1H), 5.99 (dm as a part of AA’BB’, J = 12.2 Hz, 2H), 6.62 (s, 2H), 6.92 (dm as a part of AA’BB’, J = 12.2 Hz, 2H) ppm; 13C-NMR (CDCl3) δ = 12.5, 32.8, 119.4, 121.1, 127.2, 133.0, 137.7, 147.9, 196.2 ppm; IR (KBr) νmax = 1608s, 1573s, 1396m, 1261s, 1151m, 1084s, 929w, 886m cm-1; UV (MeOH) λmax = 231 (logε = 4.11), 302 (4.42), 411 (3.17) nm; 12: yellow powder, mp 90-91 °C, 1H-NMR (CDCl3) δ = 0.08 (d, J = 11.2 Hz, 1H), 1.36 (d, J = 11.2 Hz, 1H), 3.84 (s, 6 H), 6.13 (s, 2H), 6.35 (d, J = 11.2 Hz , 2H), 7.39 (d, J = 11.2 Hz, 2H), 7.41 (s, 2H) ppm; 13C-NMR (CDCl3) δ = 36.1, 52.6, 86.2, 120.4, 124.8, 126.9, 137.2, 150.3, 150.7, 163.0, 192.1 ppm; IR (KBr) νmax = 2927m, 2854w, 1736s, 1719s, 1706s, 1655m, 1637s, 1596s, 1438m, 1300s, 1259s, 1216s, 1112m cm-1; UV (MeOH) λmax = 241 (logε = 3.82), 309(4.08), 383 (3.11) nm; HRMS: Calcd for C20H16O6 352.0947; Found 352.0949. 13: Yellow crystals, mp 235-237 °C, 1H-NMR (CDCl3) δ = 0.16 (d, J =13.2 Hz, 1H), 1.92 (d, J = 13.2 Hz, 1H), 3.85 (s, 6H), 3.86 (s, 6H), 6.13 (s, 2H), 7.52 (s, 2H), 8.07 (s, 2H) ppm; 13C-NMR (CDCl3) δ = 33.3, 52.7, 52.9, 86.2, 117.5, 122.7, 128.3, 139.7, 150.6, 151.7, 162.7, 164.7, 191.9 ppm; IR (KBr) νmax = 1716s, 1699s, 1663m, 1638m, 1437m, 1307m, 1273s, 1226s cm-1; UV (MeOH) λmax = 216 (logε = 3.98), 265 (4.30), 311 (4.51), 391 (3.47) nm; HRMS: Calcd for C24H20O10 468.1056; Found 468.1092.
12. The X-ray data for 8: C18H14O6, Mw = 326.30, monoclinic, space group P21/c (#14), a = 11.574(5), b =7.529(3), c = 17.605(4) Å, β = 100.06(2) deg, V = 1510.4(9) Å3, Z = 4, Dcalc = 1.435 g cm-3, μ(Mo-Kα) = 1.09 cm-1, 5352 independent reflections, 217 parameters, R = 0.050, Rw = 0.116, T = 298 K. Those for 13: C24H20O10, Mw = 468.42, triclinic, space group P1 / (#2), a = 9.788(3), b = 14.132(4), c = 9.179(3) Å, β = 116.27(2) deg, V = 1071.2(6) Å3, Z = 2, Dcalc = 1.452 g cm-3, μ(Mo-Kα) = 1.14 cm-1, 5344 independent reflections, 387 parameters, R = 0.053, Rw = 0.135, T = 298 K. Estimated standard deviations for the bond lengths and angles are 0.004-0.007 (ang) and 0.2-0.4 (deg), respectively, for the non-hydrogen atoms. Crystallographic data excluding structures have been deposited with the Cambridge Crystallographic Data Centre as supplementary publication numbers CCDC 695660 for 8 and CCDC 695659 for 13. A copy of the data can be obtained free of charge from CCDC, 12 Union road, Cambridge CB2 1EZ. UK [DIRECT LINE: +44 1223 762910, Fax: +44 (0) 1223-336033 or e-mail: linstead@ccdc.cam.ac.uk; deposit@ccdc.cam.ac.uk