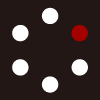
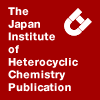
HETEROCYCLES
An International Journal for Reviews and Communications in Heterocyclic ChemistryWeb Edition ISSN: 1881-0942
Published online by The Japan Institute of Heterocyclic Chemistry
e-Journal
Full Text HTML
Received, 24th July, 2008, Accepted, 28th August, 2008, Published online, 1st September, 2008.
DOI: 10.3987/COM-08-S(F)57
■ Stereoslelctive Synthesis of a 4a,9-Disubstituted Octahydroacridine from Isatin
Hideki Abe, Yoshimi Sato, Kazuhiro Watanabe, Sakae Aoyagi,* Chihiro Kibayashi, and Tadashi Katoh*
Department of Synthetic Organic Chemistry, Tohoku Pharmaceutical University, 4-4-1 Komatsushima, Aoba-ku, Sendai 981-8558, Japan
Abstract
An efficient method for stereoselective synthesis of a 4a,9-disubstituted octahydroacridine derivative from isatin (2,3-indolinedione) was developed; the method is based on an intramolecular reaction of an N-acyliminium ion with a conjugated diene system.INTRODUCTION
Acridine derivatives, particularly octahydroacridines, form an important class of bioactive molecules in the field of pharmaceuticals and are inhibitors of gastric acid secretion.1 Although many different methods have thus far been reported for construction of the octahydroacridine skeleton,2–7 almost of these reactions lack stereoselectivity.
The N-acyliminium ion is an extremely important species in the synthesis of nitrogen-containing natural products. A large number of reactions between N-acyliminium ions and nucleophiles have been developed to date, and these have found widespread use in total syntheses of bioactive natural products,8 in which species such as olefins, allylsilanes, and aromatic rings act as π-nucleophiles in inter- or intramolecular reactions involving spirocyclization.9–14 We recently reported that a total synthesis of tricyclic marine alkaloids based on N-acyliminium ion–conjugated diene spirocyclization strategy.15 Herein, we report the stereoselective and efficient synthesis of a 4a,9-disubstituted octahydroacridine derivative 4 from isatin, which was discovered during an investigation of formic acid–induced spirocyclization of N-acylindolium ions with conjugated dienes.
RESULTS AND DISCUSSION
We began our investigation by preparing hemiaminal 3, an N-acylindolium ion precursor, from isatin (2,3-indolinedione, 1) as shown in Scheme 1. The N-Boc-oxyindole derivative 2 was obtained in two steps by protection of the benzylic carbonyl group in 1 as an ethylene acetal, and of the anilinic nitrogen atom with a Boc group.16 Addition of a Grignard reagent derived from (2E,4E)-9-bromonona-2,4-diene to 2 provided hemiaminal 3 in 56% yield. Treatment of 3 with formic acid in CH2Cl2 at 0 °C resulted in stereoselective formation of octahydroacridine derivative 4 in 54% yield as a single isomer, rather than spirocyclic compound 5, which is a reaction product expected from formic acid–induced spirocyclization based on our previous investigations.15
Although it was not possible to confirm the stereochemistry of the resulting acridine derivative 4 itself, the relative configuration of the octahydroacridine structure was determined as 4aR*,9S*,9aS* (Figure 1) by X-ray crystallographic analysis of the hydrochloride salt 6·HCl, which was derived from 4 via
deformylation followed by treatment with 1 M HCl, as shown in Scheme 2.
A reasonable mechanism for the formation of the octahydroacridine skeleton from the hemiaminal 3 is assumed as shown in Scheme 3 by a π-complex theory.17 Thus, an N-acylindolium ion 7 generated in situ from 3 forms a π-complex 8 with a nitrogen stabilized carbocation. Nucleophilic attack of the olefin moiety through a chairlike six-membered transition structure forms an intermediate π-complex 9 with a stable allyl cation in the side chain. Subsequent nucleophilic attack of the aromatic ring to an allyl cation, giving rise to an iminium intermediate 10, followed by aromatization then delivers a octahydroacridine skeleton 11, which is finally solvolyzed by formic acid to produce the octahydroacridine 4.
In summary, we have demonstrated the stereoselective and efficient construction of an octahydroacridine skeleton from 2,3-indolinedione. This methodology offers a new route for the synthesis of 4a,9-disubstituted octahydroacridine derivatives, the new acridine analogues. Investigation of the detailed reaction mechanism is currently in progress in our laboratories.
EXPERIMENTAL
tert-Butyl 2-hydroxy-3,3-ethylenedioxy-2-[(5E,7E)-nona-5,7-dienyl]indoline-1-carboxylate (3). To a cooled (0 °C) solution of 2 (1.28 g, 4.40 mmol) in THF (44 mL) was added dropwise a solution of Grignard reagent (13 mL), freshly prepared from (2E,4E)-9-bromonona-2,4-diene (2.68 g, 13.2 mmol) and magnesium (321 mg, 13.2 mmol) in THF solution, and the reaction mixture was stirred for 40 min at 0 °C. Saturated aqueous NH4Cl was added and the mixture was extracted with Et2O (2 × 150 mL). The combined organic layers were washed with brine, dried (MgSO4), and concentrated in vacuo. The residue was purified by column chromatography on silica gel (hexane–AcOEt, 5:1) to give 3 (1.02 g, 56%) as a yellow oil. IR (neat) 3393, 2977, 2929, 1731 cm–1; 1H NMR (400 MHz, CDCl3) δ 1.19–1.33 (4H, m), 1.43–1.51 (11H, m, including 9H, s, at δ 1.51), 1.70 (3H, d, J = 6.7 Hz), 1.90–2.02 (2H, m), 2.39 (1H, t, J = 7.3 Hz), 4.03–4.32 (4H, m), 5.41–5.60 (2H, m), 5.88–6.00 (2H, m), 7.08 (1H, m), 7.33 (1H, m), 7.62 (1H, m), 7.99 (1H, d, J = 8.2 Hz); 13C NMR (100.6 MHz, CDCl3) δ 18.0, 22.9, 28.3 (3C), 29.7, 32.1, 36.2, 65.5 (2C), 80.3, 95.4, 108.0, 121.7, 122.9, 123.5, 125.2, 126.7, 130.3, 130.6, 131.3, 131.6, 136.8, 153.1; HRMS (ESI–TOF) calcd for C24H34NO5 ([M+H]+) 416.2437, found 416.2445. Anal. Calcd for C24H33NO5: C, 69.37; H, 8.00; N, 3.37. Found: C, 69.49; H, 8.09; N, 3.17.
2-(Formyloxy)ethyl (4aR*,9S*,9aS*)-9-[(1E)-prop-1-enyl]-1,3,4,9,9a,10-hexahydroacridine-4a(2H)-
carboxylate (4). To a cooled (0 °C) solution of 3 (146 mg, 0.350 mmol) in CH2Cl2 (3.5 mL) was added dropwise formic acid (3.5 mL). After stirring for 48 h at 0 °C, the organic solvent was removed in vacuo and the residue was purified by column chromatography on silica gel (hexane–AcOEt, 5:1) to give 4 (65.2 mg, 54%) as a yellow oil. IR (neat) 3373, 2936, 2859, 1731 cm–1; 1H NMR (400 MHz, CDCl3) δ 1.26–1.41 (2H, m), 1.61–1.84 (9H, m, including 3H, dd, J = 6.4, 1.6 Hz, at δ 1.76), 2.08 (1H, dd, J = 11.2, 1.3 Hz), 3.40 (1H, t, J = 9.8 Hz), 4.16–4.32 (5H, m), 5.14 (1H, ddd, J = 15.0, 9.3, 1.6 Hz), 5.63 (1H, dq, J = 15.0, 6.5 Hz), 6.51 (1H, dd, J = 7.9, 1.0 Hz), 6.69 (1H, td, J = 7.5, 1.1 Hz), 6.96 (1H, t, J = 7.6 Hz), 7.05 (1H, dd, J = 7.7, 1.0 Hz), 7.87 (1H, s); 13C NMR (100.6 MHz, CDCl3) δ 17.9, 22.4, 25.8, 26.1, 37.1, 44.3, 44.8, 60.4, 61.4, 61.5, 114.7, 118.6, 124.7, 126.8, 128.2, 129.9, 133.8, 142.6, 160.4, 173.6; HRMS (ESI–TOF) calcd for C20H26NO4 ([M+H]+) 344.1826, found 344.1852.
2-Hydroxyethyl (4aR*,9S*,9aS*)-9-[(1E)-prop-1-enyl]-1,3,4,9,9a,10-hexahydroacridine-4a(2H)-
carboxylate (6) and its hydrochloride salt (6·HCl). To a solution of 4 (15.8 mg, 46.1 µmol) in MeOH
(0.5 mL) was added NaBH4 (2.75 mg, 69.1 µmol) at 0 °C. After stirring for 30 min at 0 °C, H2O (1 mL) was added to the reaction mixture. The mixture was extracted with Et2O (2 × 10 mL), and the combined organic layers were washed with brine, dried (MgSO4), and concentrated in vacuo. The residue was purified by column chromatography on silica gel (hexane–AcOEt, 3:1) to afford 6 (14.3 mg, 90%) as a colorless oil. IR (neat) 3522, 3367, 2958, 2936, 1730 cm–1; 1H NMR (400 MHz, CDCl3) δ 1.28–1.78 (10H, m, including 3H, d, J = 6.4 Hz, at δ 1.76), 1.83–1.86 (2H, m), 2.14 (1H, d, J = 12.3 Hz), 3.34 (1H, t, J = 9.8 Hz), 3.48–3.59 (2H, m), 4.04 (1H, m), 4.18–4.26 (2H, m), 5.15 (1H, ddd, J = 14.9, 9.5, 1.0 Hz), 5.63 (1H, m), 6.54 (1H, d, J = 7.9 Hz), 6.72 (1H, t, J = 7.4 Hz), 7.00 (1H, t, J = 7.4 Hz), 7.09 (1H, d, J = 7.6 Hz); 13C NMR (100.6 MHz, CDCl3) δ 13.4, 17.9, 25.8, 26.2, 36.7, 44.6, 45.2, 60.6, 61.1, 65.8, 114.5, 118.8, 125.0, 127.0, 128.3, 130.1, 133.7, 143.2, 174.1; HRMS (ESI–TOF) calcd for C19H26NO3 ([M+H]+) 316.1913, found 316.1923.
To a solution of 6 in MeOH was added 1M aqueous HCl, and the mixture was concentrated in vacuo. The resulting white crystals were recrystallized from THF–hexane to give 6·HCl as colorless prisms. Mp 144–145 °C; IR (KBr) 3441, 2946, 1749 cm–1; 1H NMR (400 MHz, CDCl3) δ 1.32 (1H, br s), 1.59 (1H, m), 1.72–1.91 (10H, m, including 3H, dd, J = 6.5, 1.6 Hz, at δ 1.73), 2.35 (1H, m), 3.35 (1H, t, J = 4.7 Hz), 3.50–3.58 (2H, m), 4.00–4.15 (2H, m), 5.11 (1H, ddd, J = 15.1, 9.2, 1.6 Hz), 5.72 (1H, dq, J = 14.9, 6.5 Hz), 7.17 (1H, m), 7.22–7.29 (3H, m); 13C NMR (100.6 MHz, CDCl3) δ 17.6, 22.8, 26.0, 27.1, 35.2, 44.6, 45.6, 60.5, 65.9, 68.3, 122.4, 129.1, 129.2, 131.9, 132.3, 132.4, 133.2, 133.3, 170.1; HRMS (ESI–TOF) calcd for C19H26NO3 ([M·HCl–Cl]+) 316.1913, found 316.1910.
Crystal data for hydrochloride salt (6·HCl). Crystal size: 0.45 × 0.45 × 0.25 mm; Cell dimension: a = 8.5990 (13) Å, b = 10.015 (3) Å, c = 11.427 (3) Å; Cell volume: 936.2 (3) Å3; Z = 2.
ACKNOWLEDGEMENTS
This work was supported in part by a grant for private universities and a Grant-in-Aid for Science Research from the Ministry of Education, Culture, Sports, Science, and Technology (MEXT) of Japan (No. 17790020) and also by a grant for the High Technology Research Program from MEXT and the Promotion and Mutual Aid Corporation for Private Schools of Japan.
Dedicated to Professor EmeritusKeiichiro Fukumoto on the occasion of his 75th birthday
References
1. (a) A. Canas-Rodriguez, R. G. Canas, and A. Mateo-Bernardo, An. Quin., Ser. C, 1987, 83, 24; (b) P. Lafargue, J. L. Moriniere, P. Pont, and J. Mennier, R. C. Acad. Sci. Ser. C, 1970, 270, 1186; (c) V. G. Ermolaeva, V. G. Yashunskii, A. I. Polezhaeva, and M. D. Mashkovskii, Khim.-Farm. Zh., 1968, 2, 20.
2. M. Nagai, Bull. Chem. Soc. Jpn., 1991, 64, 330. CrossRef
3. K. Sakanishi, I. Mochida, H. Okazaki, and M. Soeda, Chem. Lett., 1990, 319. CrossRef
4. K. Sakanishi, M. Ohira, I. Mochida, H. Okazaki, and M. Soeda, J. Chem. Soc., Perkin Trans. 2, 1988, 1769. CrossRef
5. R. W. Layer and J. C. Westfahl, J. Org. Chem., 1979, 44, 1146. CrossRef
6. S. Sakane, Y. Matsumura, Y. Yamamura, Y. Ishida, K. Maruoka, and H. Yamamoto, J. Am. Chem. Soc., 1983, 105, 672. CrossRef
7. J. Tanaka, K. Takabe, K. Taniguchi, and T. Katagiri, Nippon Kagaku Kaishi, 1981, 1043.
8. (a) W. N. Speckamp and H. Hiemstra, Tetrahedron, 1985, 41, 4367; CrossRef (b) W. N. Speckamp and M. J. Moolenaar, Tetrahedron, 2000, 56, 3817; CrossRef (c) B. E. Maryanoff, H.-C. Zhang, J. H. Coheu, I. J. Turchi, and C. A. Maryanoff, Chem. Rev., 2004, 104, 1431. CrossRef
9. (a) H. E. Schoemaker and W. N. Speckamp, Tetrahedron Lett., 1978, 1515; CrossRef (b) H. E. Schoemaker and W. N. Speckamp, Tetrahedron, 1980, 36, 1515. CrossRef
10. (a) D. A. Evans and E. W. Thomas, Tetrahedron Lett., 1979, 411; CrossRef (b) D. A. Evans and R. E. Cherpeck, J. Am. Chem. Soc., 1982, 104, 3695. CrossRef
11. W. H. Whaley and T. R. Govindachari, Org. React., 1951, 6, 151.
12. E. Langkopf and D. Schinzer, Chem. Rev., 1995, 95, 1375. CrossRef
13. C. Y. Hong, N. Kado, and L. E. Overman, J. Am. Chem. Soc., 1993, 115, 11028. CrossRef
14. A. R. Ofial and H. Mayr, J. Org. Chem., 1996, 61, 5823. CrossRef
15. (a) H. Abe, S. Aoyagi, and C. Kibayashi, J. Am. Chem. Soc., 2005, 127, 1473; CrossRef (b) H. Abe, S. Aoyagi, and C. Kibayashi, Angew. Chem., Int. Ed., 2002, 41, 3017. CrossRef
16. N. Papaioannou, J. T. Blank, and S. J. Miller, J. Org. Chem., 2003, 68, 2728. CrossRef
17. M. J. S. Dewar and C. H. Reynolds, J. Am. Chem. Soc., 1984, 106, 1744. CrossRef