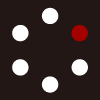
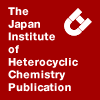
HETEROCYCLES
An International Journal for Reviews and Communications in Heterocyclic ChemistryWeb Edition ISSN: 1881-0942
Published online by The Japan Institute of Heterocyclic Chemistry
e-Journal
Full Text HTML
Received, 24th July, 2008, Accepted, 27th August, 2008, Published online, 28th August, 2008.
DOI: 10.3987/COM-08-S(F)58
■ Enantioselective Route to Aryl(1,3-butadien-2-yl)methanols: Formal Synthesis of (-)-Sporochnol A
Daisuke Yanagimoto, Kazuyuki Kawano, Keisuke Takahashi, Jun Ishihara, and Susumi Hatakeyama*
Department of Pharmaceutical Sciences, Graduate School of Biomedical Sciences, Nagasaki University, 1-14 Bunkyo-machi, Nagasaki 852-8521, Japan
Abstract
Ti(IV)-promoted reaction of the chiral acetals derived from aromatic aldehydes with 1-(tri-n-butyl)stannyl-2,3-butadiene followed by removal of the chiral auxiliary gave aryl(1,3-butadien-2-yl)methanols with high enantiomeric purity (>90% ee). The synthetic utility of this method was demonstrated by the formal synthesis of (-)-sporochnol A, a terpene possessing a chiral benzylic quaternary carbon center.We have previously developed an effective method for the highly enantioselective preparation of dienol 5 via Lewis acid-promoted reaction of chiral acetal 1 with buta-2,3-dienylsilane 2.1,2 In addition, we have demonstrated the synthetic utility of 5 as a chiral building block by utilizing it in the synthesis of natural products.3 However, this method could not be applied to the synthesis of the compounds having aromatic substituents 5 (R = Ar) due to the instability of 4 under the reaction conditions. We now report a highly enantioselective route to aryl(1,3-butadien-2-yl)methanols 5 (R = Ar) through butadienylation of 1 with buta-2,3-dienylstannane 34,5 using a mild mixed Ti(IV) reagent as a promoter.
We also demonstrate the utility of this methodology by the formal synthesis of (–)-sporochnol A, an enantiomer of which is a fish deterrent isolated from the marine alga Sporchnus bolleanus.6,7
To establish a reliable method for the preparation of aryl(1,3-butadien-2-yl)methanols 5 (R = Ar) in high enantiomeric purity, we examined the dienylation of chiral acetals 8a-g, prepared by acetalization of aldehydes 6a-g with 7,8 using BF3·Et2O and 3TiCl4·Ti(O-i-Pr)4 as a promoter according to the procedure4 previously established for the reaction of dimethyl acetals (Scheme 2, Table 1). The reactions were evaluated by HPLC analysis using a chiral column after converting 9 to 10 by Swern oxidation followed by retro-Michael reaction. The absolute configurations of 10 were determined to be S by 1H NMR analysis of R- and S-MTPA esters. As can be seen from Table 1, BF3·Et2O did not promote the dienylation at all in the case of chiral acetals unlike the corresponding dimethyl acetals4 (entries 1 and 3). On the other hand, 3TiCl4·Ti(O-i-Pr)4 was found to effectively promote the dienylation of 8a and other acetals 8f,g having electron-withdrawing groups (entries 2, 9, and 10). However, in the case of o-nitrophenyl derivative 8e, the desired product 9e was not produced due to the extreme insolubility of
the resulting Ti(IV)-coordinated complex in the reaction media (entry 8). For the reactions of rather sensitive acetals 8b-d having electron-donating groups, addition of 1.2 equiv of acetonitrile was found to markedly improve the yields of 99 by attenuating the Lewis acidity of 3TiCl4·Ti(O-i-Pr)4 (entries 4-7).
Having established a highly enantioselective route to dienols 10, we then investigated the synthesis of (–)-sporochnol A (13) using 10d10 as a chiral building block. It is of importance to provide a promising method for the construction of chiral benzylic quaternary carbon centers, which often occur in biologically intriguing terpenes and alkaloids.11 We envisaged that acid-promoted reaction of epoxy alcohol 11, available from 10, would allow us to access compound 12 having a benzylic quaternary carbon center as depicted in Scheme 3. Based on literature precedents,12 it was anticipated that this rearrangement would take place stereoselectively with inversion of configuration of the quaternary center.
According to the procedure we established previously,13 dienol 10d was subjected to VO(acac)2-catalyzed epoxidation to give epoxide 14 as a single diastereomer in almost quantitative yield. When epoxide 14 (90% ee) was treated with PPTS (0.1 equiv) in CH2Cl2 at room temperature followed by methoxymethyl chloride in the presence of Hünig’s base in the same flask, the desired product 15 was obtained in 50% yield together with α,β-unsaturated aldehyde 16 (10% yield). However, the enantiomeric purity was eroded to 80% ee from 90% ee (the enantiomeric purity of the starting epoxide 14), indicating that some racemization took place during the transformation of 14 to 15. After considerable experimentation, we eventually found conditions where the rearranged product could be obtained without racemization. Thus, upon exposure of 14 to acetalization conditions8 using 1,2-di(trimethylsilyloxy)ethane and trimethyl triflate in CH2Cl2 at –40 °C, acetal 1714 of 88% ee was produced in 65% yield. Acetal 17 was then converted to ester 20 via 18 and 19 by a four-step sequence involving Dess-Martin oxidation, Wolff-Kishner reduction, acidic hydrolysis, and Horner-Emmons olefination. The specific rotation, [α]D18 +17.6 (c 1.00) [lit.7c [α]D20 +19.6 (c 1.00)], and spectral data of 20 thus obtained was identical with those reported for the precursor of (–)-sporochnol A (13) by Fadel and Vandromme7c so that the formal synthesis of 13 was accomplished. At this stage, it was unambiguously proved that the above-mentioned rearrangement of 14 occurred with inversion of configuration of the quaternary center.
In conclusion, the present work provides a highly enantioselective method for the preparation of aryl(1,3-butadien-2-yl)methanols and demonstrates an effective methodology for the construction of a chiral benzylic quaternary carbon center.
ACKNOWLEDGEMENTS
We are grateful to Professor Meiming Luo (Sichuan University) for his contribution as a postdoctoral fellowship of the Japan Promotion of Science in the early stage of this work.
† Dedicated to Professor Emeritus Keiichiro Fukumoto on the occasion of his 75th birthday.
References
1. S. Hatakeyama, K. Sugawara, M. Kawamura, and S. Takano, Tetrahedron Lett., 1991, 32, 4509. CrossRef
2. For other enantioselective approaches to dienols 5, see: a) R. Soundararajan, G. Li, and H. C. Brown, J. Org. Chem., 1996, 61, 100; CrossRef b) C.-M. Yu, S.-J. Lee, and M. Jeon, J. Chem. Soc., Perkin Trans. 1, 1999, 3557; CrossRef c) J. A. Smulik and S. T. Diver, Org Lett., 2000, 2, 2271. CrossRef
3. For a review, see: S. Hatakeyama, J. Synth. Org. Chem. Jpn., 1997, 55, 793.
4. M. Luo, Y. Iwabuchi, and S. Hatakeyama, Chem. Commun., 1999, 267. CrossRef
5. M. Luo, Y. Iwabuchi, and S. Hatakeyama, Synlett, 1999, 1109. CrossRef
6. Y.-C. Shen, P. I. Tsai, W. Fenical, and M. E. Hay, Phytochemistry, 1993, 32, 71. CrossRef
7. For the enantioselective syntheis of sporochnol, see: a) M. Takahashi, Y. Shioura, T. Murakami, and K. Ogasawara, Tetrahedron: Asymmetry, 1997, 8, 1235; CrossRef b) T. Kamikubo, M. Shimizu, and K. Ogasawara, Enantiomer, 1997, 2, 297; c) A. Fadel and L. Vandromme, Tetrahedron: Asymmetry, 1999, 10, 1153; CrossRef d) C. A. Luchaco-Cullis, H. Mizutani, K. E. Murphy, and A. H. Hoveyda, Angew. Chem. Int. Ed., 2001, 40, 1456; CrossRef e) Y. Kita, A. Furukawa, J. Futamura, K. Ueda, Y. Sawama, H. Hamamoto, and H. Fujioka, J. Org. Chem., 2001, 66, 8779; CrossRef f) S. Ohira, A. Kuboki, T. Hasegawa, T. Kikuchi, T. Kutsukake, and M. Nomura, Tetrahedron Lett., 2002, 43, 4641; CrossRef g) R. Alibés, F. Busqué, G. G. Bardají, P. de March, M. Figueredo, and J. Font, Tetrahedron: Asymmetry, 2006, 17, 2632. CrossRef
8. T. Tsunoda, M. Suzuki, and R. Noyori, Tetrahedron Lett., 1980, 21, 1357. CrossRef
9. Compound 9d: [α]D20 -118.8° (c 2.68, CHCl3); FTIR (neat) 3438, 1610, 1510, 1250, 1036 cm-1; 1H NMR (300 MHz, CDCl3) δ 7.29 (d, J = 8.4 Hz, 2H), 6.87 (d, J = 8.7 Hz, 2H), 6.26 (dd, J = 11.1, 17.7 Hz, 1H), 5.39 (brd, J = 1.2 Hz, 1H), 5.29 (brs, 1H), 5.23 (dd, J = 1.2, 18.0 Hz, 1H), 5.10 (s, 1H), 5.00 (d, J = 10.5 Hz, 1H), 4.11-4.01 (m, 1H), 3.87-3.70 (m, 1H), 3.79 (s, 3H), 2.55 (d, J = 3.0 Hz, 1H), 1.66-1.51 (m, 2H), 1.24 (d, J = 6.3 Hz, 3H), 1.09 (d, J = 6.0 Hz, 3H); 13C NMR (75 MHz, CDCl3) δ 159.3, 146.5, 136.1, 132.2, 128.7, 115.4, 114.9, 113.9, 78.1, 70.2, 64.2, 55.2, 44.5, 23.3, 19.1; HRMS calcd for C17H24O3 (M+) 276.1725, found 276.1725.
10. Compound 10d: [α]D22 -85.4° (c 0.61, CHCl3); FTIR (neat) 3417, 1610, 1512, 1252, 1034 cm-1; 1H NMR (300 MHz, CDCl3) δ 7.31 (d, J = 8.7 Hz, 2H), 6.88 (d, J = 8.7 Hz, 2H), 6.31 (dd, J = 11.4, 18.0 Hz, 1H), 5.44 (s, 1H), 5.44 (d, J = 3.6 Hz, 1H), 5.33 (s, 1H), 5.17 (d, J = 17.6 Hz, 1H), 5.03 (d, J = 11.1 Hz, 1H), 3.80 (s, 3H), 1.91 (dd, J = 1.7, 3.8 Hz, 1H); 13C NMR (75 MHz, CDCl3) δ 159.3, 147.7, 136.0, 134.3, 128.3, 115.4, 113.9, 73.5, 55.3; HRMS calcd for C12H14O2(M+) 190.0994, found 190.1004.
11. For reviews, see: a) S. F. Martin, Tetrahedron, 1980, 36, 419; CrossRef b) K. Fuji, Chem. Rev., 1993, 93, 2037. CrossRef
12. For reviews, see: a) K. Suzuki, J. Synth. Org. Chem. Jpn., 1988, 46, 365; b) H. Fujioka, Y. Yoshida, and Y. Kita, J. Synth. Org. Chem. Jpn., 2003, 61, 133.
13. S. Hatakeyama, K. Sugawara, and S. Takano, Tetrahedron Lett., 1991, 32, 4513. CrossRef
14. Compound 17: [α]D19 -15.8° (c 2.10, CHCl3); FTIR (neat) 3506, 2887, 1601, 1508, 1246, 1028 cm-1; 1H NMR (300 MHz, CDCl3) δ 7.38 (d, J = 9.0 Hz, 2H), 6.88 (d, J = 9.0 Hz, 2H), 6.05 (dd, J = 11.1, 18.0 Hz, 1H), 5.42 (dd, J = 1.2, 11.1 Hz, 1H), 5.31 (s, 1H), 5.25 (dd, J = 1.1, 17.9 Hz, 1H), 4.07 (ddd, J = 6.0, 11.1, 18.3 Hz, 2H), 3.91-1.86 (m, 4H), 3.80 (s, 3H), 2.34 (t, J = 6.3 Hz, 1H); 13C NMR (75 MHz, CDCl3) δ 158.4, 138.4, 131.4, 129.6, 117.7, 113.6, 107.2, 65.5, 65.2, 55.2, 53.1; HRMS calcd for C14H18O4 (M+) 250.1205, found 250.1200