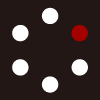
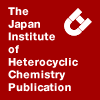
HETEROCYCLES
An International Journal for Reviews and Communications in Heterocyclic ChemistryWeb Edition ISSN: 1881-0942
Published online by The Japan Institute of Heterocyclic Chemistry
e-Journal
Full Text HTML
Received, 24th July, 2008, Accepted, 9th September, 2008, Published online, 11th September, 2008.
DOI: 10.3987/REV-08-638
■ Chemistry of 1-Methylenesugars: Synthetic Utilizations to 1’-C-Methyl-saccharides and Related Carbohydrates
Rie Namme and Shiro Ikegami*
Faculty of Pharmaceutical Sciences, Teikyo University, 1091-1, Sagamiko, Kanagawa 299-0195, Japan
Abstract
The efficient synthesis of protected 1-methylenesugars and their acid-catalyzed O-glycosidation are mentioned in connection with that of 1-C-methyl-pyranoses. Application of this process to synthesis of the pentasaccharide PI-88 analogue was accomplished in a protected form. Also the syntheses of trehalose analogues and ulsonic acid derivatives were successful in the similar process. 1,3-Dipolar cycloaddition with nitrones provides the new types of spiro-heterocycles combined with carbohydrates. The closely related methylene-carbohydrates also provide a novel synthetic process for cyclitols that are useful synthetic intermediates for voglibose and related drugs.INTRODUCTION
1. Glycosidation to 1-Methylenesugars
1-1 Preparation of 1-Methylenesugars
1-2 O-Glycosidation of 1-Methylenesugars
1-3 Stereochemistry at Anomeric Center
1-4 Synthetic Trial to Oligosaccharides (PI-88 Analogue)
2. Glycosidation of Ketopyranoses
2-1 Preparation of Ketopyranose
2-2 O-Glycosidation of Ketopyranose
2-3 Application to Synthesis of Trehalose Analogues
2-4 Application to Synthesis of Ulosonic Acid Derivatives
3. Cycloaddition to 1-Methylenesugars
3-1 Synthesis of spiro-ketodisaccharides
3-2 1,3-Dipolar Cycloaddition with Nitrones
3-3 Synthetic Utility of spiro-Sugars
CONCLUSION
INTRODUCTION
The important biological significance and potential chemotherapeutic value of carbohydrate compounds have stimulated much research activity on glycoside synthesis.
Our group has worked on the development of various sugar mimics such as ketosides, orthoester sugars, carbacyclins, aza-sugars, thia-sugars, and artificial oligosaccharides such as carbamate- and urea-linked saccharides.1,2 In this review, we mention the chemistry and synthetic utilities of unique sugars introduced a C-1 unit at anomeric position.
1. Glycosidation to 1-Methylenesugars
exo-Glycals, which have exocyclic olefin at anomeric position, have been actively examined about their preparation and utilization.3 exo-Methylenesugars, that is one of the simplest exo-glycals, are useful precursors for synthesizing C-glycosides and other C-glycosyl derivatives.4 Although C-glycosidations of exo-glycals have been well examined, their O-glycosidations were rarely reported until obtaining our observation. In this section, we describe the introduction of C-1 unit to pyranose as 1-exo-methylenesugars and its ability as glycosyl donors.
1-1 Preparation of 1-Methylenesugars
1-exo-Olefin-sugars have been prepared by several procedures, such as the Meyers’ variant of the Ramberg-Becklund reaction5, multiple step process with addition-elimination reactions6, and methylenation of sugar lactones by Tebbe’s reagent7 or dimethyltitanocene.8
Perbenzyl-1-exo-methylenesugars can be readily prepared by Tebbe-type methylenation (Petasis modification) of corresponding lactones. The reactions of d-gluco-, d-galacto, and d-mannono-1,5-lactones 1a–c with dimethyltitanocene afforded the corresponding tetra-O-benzyl-1-exo-methylenesugars 2a–c in high yields (Scheme 1).9
As the best way to elucidate the structure of the product, the X-ray single crystallographic structure of 1-methylenesugar 2a is represented in Fig.1 by ball and stick model. It is shown that 1-methylenesugar 2a is in chair conformation, which is also agreed with the 1H-NMR coupling constant. By NMR analysis, galacto- and manno-derivatives 2b and 2c were also suggested to be in a chair form.
On the other hand, this direct methylenation for the synthesis of peracetylglyconolactones could not be applied because they involve the ester function. Thus, multiple step synthesis was needed for preparing the O-acetyl-1-methylensugars.10 As shown in Scheme 2 for the preparation of acetylated methylenesugars 8, the subsequent procedures of aceylation, bromination and cyanation of the pyranose 3 afforded the compound 4. Then, the compound 4 was reductively converted into diphenylimidazolidine 5 with Raney-Ni and N,N’-diphenylethylenediamine. Acidic cleavage of the imidazolidine 5 gave the aldehyde, which was reduced to alcohol with NaBH(OAc)3, and then tosylated. The resulting tosylate 6 was treated with sodium iodide to afford the iodide 7. Then, dehydorohalogenation of 7 with AgF provided tetra-O-acetyl-1-methylenesugars 8.
1-2 O-Glycosidation of 1-Methylenesugars
Firstly, the ability of 1-methylenesugars as powerful glycosyl donors in the glycosidation reactions was investigated. The acid-promoted O-glycosidation of 1-methylenesugar 2a with methyl 2,3,4-tri-O-benzyl-d-glucopyranoside 9 was tried, affording 1-C-methyldisaccharide 10a in complete α-selectivity (Table 1). Reaction conditions in this reaction were examined precisely and optimized using 2a and 9. It was found that the glycosidation proceeded effectively with trifluoromethanesulfonic acid (TfOH) in dichloromethane at –78°C (entry 2). Other Lewis acids also promoted this glycosidation, but the yield was lower than the reaction with TfOH (entries 5–8). Furthermore, the addition of a dehydrating agent was effective in proceeding glycosidation, and MS4A (one of the molecular shieves) was the most effective and suitable additive for this reaction. It should be noted that α-disaccharide was obtained as a single isomer even with any promoters in other solvents such as diethyl ether and toluene (entries 3–4).
Under optimized conditions, the glycosidation of galacto- and manno-derivertives 2b–c was performed with acceptor 9 (Scheme 3). 1-Methylenesugars 2b and 2c exhibit similar glycosidation reactivity as the glycosyl donors and the corresponding 1’-C-methyl-α-disaccharides 10b–c were obtained in high yields. In both cases, α-glycoside was obtained exclusively and β-glycoside was not detected.
To examine the scope of this reaction, the glycosidation of 2a–c with various sugar alcohols in pyranoses and glycono-1,5-lactones was carried out (Table 2).11 Similarly in the case of methyl pyranoside 9, the
primary hydroxy group at the 6-position (6-OH) of d-galactono-1,5-lactone 11 and d-mannono-1,5-lactone 12 were well glycosylated (entries 1–2) . The equatorial secondary alcohols in 13 and 14 were also glycosylated in high yields (entries 3–6). On the other hand, the axial secondary alcohols at the 4 or 2 position (4-OH or 2-OH), 2-OH in mannopyranoside 15 and 16, were less reactive and the corresponding 1’-C-methyldisaccharides were obtained only in 26–34% yield (entries 5–6). The axial hydroxyl group would generally be less reactive for glycosylation.
Next, the glycosidation of 1-methylenesugars with glycono-1,5-lactones 17–20 were examined. It was expected that the reactivity of 4-OH in galactono-1,5-lactone 18 or 2-OH in mannono-1,5-lactone 20 could be affected by the conformational change of a pyran ring. The glycosidation of 1-methylenesugars 2a–c with glucono-1,5-lactone 17 and galactono-1,5-lactone 18 was carried out and corresponding disaccharides were obtained in high yield without any stereochemical change of alcohols to be glycosylated. In the similar way, both 19 and 20 showed good reactivity in the glycosidation of 2a–c.
In all cases, the corresponding products as 1’-C-methyldisaccharide 21–30 were obtained in complete α-selectivity and β-glycosides were not detected.12
To investigate this unusually high stereoselectivity, we examined the glycosidation using peracetylated 1-methylensugars 8a–b in which the acyl group attached to C-2 of glycosyl donor (Scheme 4). If neighboring group participation by an acetyl group are present in the reaction, β-isomers of 1’-C-methyldisaccharides might be obtained. The glycosidation of 8a–b with acceptor 31 in the presence of TfOH gave the anomerically single α-disaccharides 32a–b in moderate yields. The low yield of 32a was due to the migration of an acetyl group in the acceptor 31. These results suggest that the stereoselectivity in this reaction is not affected by the 2-O-acetyl group of the glycosyl donors 8a–b.
1-3 Stereochemistry at Anomeric Center
The configurations at the anomeric position of ketosides were confirmed finally by determination by X-ray crystallographic analyses and NMR study.
It is known that the magnitude of three-bond carbon-proton coupling constant 3JC-1,3-H depends on the
dihedral angle between the C-1/C-2 bond and C-3/3-H bond.13 In 1-C-methylpyranoside, the 1-C-methyl exocyclic carbon and 2-H axial proton are oriented synclinally in α-ketoside and antipeliplanarly in β-ketoside (Figure 2). Therefore, the configuration of gluco- and galacto-ketopyranosides was determined by the analysis of vicinal C-H coupling constants 3JC,H in NMR spectra. Chemical shifts of 1-C-methyl and anomeric carbon were also compared.
By comparison of chemical shift and coupling constant 3J1-Me,2-H of disaccharides 21a and 21a(β) 14 with those of known compound 33α and 33β15, major isomer of 21a was assigned to α-glycoside (Table 3). The vicinal coupling constant 3J1-Me,2-H were measured for other 1’-C-methyldisaccharides, which were approximately 1.8 ~ 2.0 Hz. The result inidicates that newly formed glycosidic bonds have the α-configuration in gluco- and galacto-ketopyranosides.
manno-Derivative of 1,6-linked disaccharide 22c was obtained as colorless crystals. The X-ray crystallographic structure is shown in Figure 3. This X-ray analysis elucidated that manno-pyranoside possessed a chair conformation and α-anomeric configuration. This result suggests that the other 1-C-methyl-manno-pyranosides also possess the α-configuration because only single isomer was formed in every case. Thus, we could be fairly certain that the TfOH-promoted O-glycosidations of gluco-, galacto- and manno-1-methylenesugars give α-glycosides selectively.
1-4 Synthetic Trial to Oligosaccharides (PI-88 Analogue) 16,17
The acid-promoted O-glycosidation of 1-methylenesugars afforded 1’-C-methyldisaccharides not only in excellent yield but also in a completely α-stereoselective manner. Taking advantage of this α-selective glycosidation, we explored the synthesis of artificial α-glycosidic sugar chain.
In order to examine the application to the oligosaccharide synthesis, the synthesis of Glc-α(1-6)-Glc linked 1-C-methyloligosaccharide was attempted (Scheme 5). 6-O-p-Methoxybenzyl (PMB)-protected 1-exo-methylenesugar 34 prepared for differentiating the 6-O-hydroxyl group from the other hydroxy groups was carried out under the established glycosidation conditions with hydroxylactone 11 and then the produced 1’-C-methyl-α-disaccharide 35 was converted into the corresponding glycosyl donor 36 by the methylenation. Disaccharide 35 was also converted into the acceptor 37 by the selective removal of PMB. Then, 2+2 glycosidation between 36 and 37 was performed and the tetrasaccharide 38 was obtained in complete α-selectivity. The result shows that this O-glycosidation of 1-methylenesugars could provide the preparative methodology to the new analogues of α-glycosidic oligosaccharides.
We next tried the synthetic application to the analogues of the bioactive sugar chains. Posphomannopentaose sulfate (PI-88), which was developed by Palish et al. in 1999 from the yeast P. holstii, shows potent heparanase inhibitory activity and antiangiogenesis properties.18 As PI-88 consists of five mannose units with all α-glycoside linkages, we planed that 1-C-methyl-substituted PI-88 analogue could be synthesized utilizing the α-stereoselective O-glycosidation of exo-glycals.
PMB-Protected manno-methylenesugar 39 prepared selectively was carried out under the O-glycosidation with 20. In this case, some modification of the rection conditions was needed. It was found that methanesulfonic acid (MsOH) was the best promoter to this glycosidation. Otherwise unexpected cyclized by-product was generated. The glycosidation of 39 with 20 in the presence of MsOH proceeded
smoothly, and the desired 1’-C-methyldisaccharide 40 was obtained α-stereoselectively in high yield. The removal of the PMB protection from the compound 40 with DDQ gave the disaccharidic glycosyl acceptor 41, which was glycosylated again to afford trisaccharide 42 as a single isomer. Further chain-elongation sequentially involving the removal of PMB and glycosidation furnished the pentasaccharide 46 in complete α-selectivity (Scheme 6).
During the investigation of the synthesis of pentasaccharide 46, 2+3 glycosidation was also examined. Disaccharidic donor 47 and trisaccharidic acceptor 43 were synthesized respectively and the glycosidation was performed. However, the glycosidation of 47 was rather unreactive and the desired pentasaccharide 46 was obtained only in 25% yield (Scheme 7). These results suggest that in some case, a step-by-step synthesis would be suitable in the synthesis of 1-C-methyl-oligosaccharide.
Finally, It would be hardly to say that we have not reached the final goal in this research. The final deprotection of the fully protected pentasaccharide 46 was not succeeded in spite of every successful trial because of the unexpectedly unstable glycosidic bonds presented in 46.
It is shown that the acid-promoted O-glycosidation by utilizing 1-methylenesugars provides a useful and efficient method, giving 1’-C-methyl-α-disaccharide with high α-selectivity in high yield. Furthermore, The method could be applied to the synthesis of the related α-oligosaccharide mimics.
2. Glycosidation of Ketopyranoses19
It is expected that the synthetic approach to 1-C-methyl-α-disaccharides can be accessed directly from ketopyranose. We also explored the glycosidation of ketoses and confirmed that 1-C-methyl-α-glycosides could be obtained as well. That is to say, the glycosidation of ketose is considered as another method to introduce C-1 unit at the anomeric position of the sugar chain. In this section, the preparation of
ketopyranose and its conversion into ketoside-type disaccharides are mentioned.
2-1 Preparation of Ketopyranose
Ketopyranoses were readily prepared from lactones by the addition of alkyl group with organometallic reagents. Here, the lactones 1a–c were treated with methyl lithium and converted into 1-C-methyl pyranose 48a–c, respectively. The resulting ketoses were acetylated by treatment with acetic anhydride and butyl lithium.20 These ketopyranoses were also used as glycosyl donors for 1-C-methyldisaccharide synthesis.
2-2 O-Glycosidation of Ketopyranose
We first investigated the reaction conditions for the O-glycosidation using 48a by employing methyl glycoside 9 as a glycosyl acceptor.
As the glycosidation of 1-methylenesugars was achieved in excellent yield by promoting with TfOH, the O-glycosidation of ketose 48a was first examined under the same conditions. It was found that the O-glycosidation of 48a with TfOH did not complete even in the use of 1.5 eq. of TfOH. After several attempts by changing Lewis acid promoters, it was found that TMSOTf was most effective for this glycosidation reaction. The best conditions were the use of 0.2 eq. of TMSOTf in dichloromethane in the presence of MS4A. The reaction also tried in other solvents such as diethyl ether and toluene, but the yield was moderate. It should be noted that the O-glycosidation of the 1-C-methyl-glucopyranose 48a took place stereoselectively in the same manner and provided α-disaccharide 10a as a sole product.
Following the above described conditions, the glycosidation of galacto- and manno-derivatives 48b and 48c with 9 was carried out and gave the corresponding α-disaccharides 10b and 10c, respectively, with complete α-stereoselectivity (Scheme 9). The results indicate that the stereochemistry of the resulting ketoside is not affected by stereochemistry of the 2- or 4- hydroxyl groups.
Starting from α-ketopyranose, α-ketoside was selectively formed. These results indicate that the O-glycosidation proceeds in SN1 mechanism.
The transglycosidations of methyl 1-C-methylpyranosides 50a–c were examined in the purpose of exploring the scope of this reaction. Because of the higher stability of the methyl glycosidic ether bond, stronger acidic condition was required to the transglycosidation and tin tetrachloride was effective for transglycosidation (Scheme 10).
The O-transglycosidations afforded the corresponding α-glycosides as single isomers as well as the cases of 1-exo-methylenesugars and 1-C-methylsugars. In these glycosidations, a tertiary oxocarbenium ion V as a stable intermediate was generated from II or III and then combined with sugar alcohols to form the α-glycoside VI. The stability of the tertiary oxocarbenium ion V resulted in the α-stereoselectivity in the glycosidations due to the anomeric effect.21
2-3 Application to Synthesis of Trehalose Analogues22
Utilizing α-selective O-glycosidation of 1-C-methyl-pyranose, we explored the synthesis of α-ketosyl α-aldosides. α-Ketosyl α-aldosides are 1,1’-linked non reducing disaccharide containing ketoside-bond, and they are regarded as 1-C-methyl analogues of trehalose.23
We are also interested in whether or not the glycosidation proceeds in cross process between ketopyranose and aldopyranose. After detailed examination, it became clear that 1-O-acetyl-1-C-methylpyranoses 49a–c were useful as glycosyl donors and trimethylsilyl α-pyranosides 51a–c as glycosyl acceptors.24 The glycosidation of ketose 49a–c with 51a–c was carried out in the presence of TMSOTf in a solution of dichloromethane. The reactions proceeded effectively in exclusive α-selectivity to give α-ketopyranosyl α-aldopyranoside 52aa–cc in high yields. The stereochemistry of trimethylsilyl α-aldopyranoside was retained. However, only in the glycosidation of 49a with 51a, partially anomerization of α-aldopyranoside took place and then afforded the mixture of α,α-disaccharides 52aa and α,β-disaccharides 55aa in a ratio of 52aa:55aa=10:1.
Similarly, we investigated the glycosidation using trimethylsilyl β-pyranoside 54a–c as glycosyl acceptors. The glycosidation of ketoses 49a–c with β-aldopyranoside 54a–b proceeded α-selectively, affording α-ketopyranosyl β-aldopyranosides 55aa–cb. The anomerization of β-aldopyranoside 54a–b to α-aldopyranosides 51a–b was not observed and only α,β-disaccharides 55aa–cb were formed in each cases. Therefore, both α,α- and α,β-disaccharides were selectively synthesized. α-Ketopyranosyl aldopyranoside 52aa–cc and 55aa–cb were deprotected respectively by palladium-catalyzed hydrogenolysis and corresponding trehalose analogues 53aa–cc and 56aa–cb were obtained nicely.
In this reaction, it is supposed that the formation of oxocarbenium intermediate III from ketose I is generated faster than IV from aldoside II, so that cross-linked ketosyl aldoside can be produced.
We also tried the synthesis of dimethyl analogue of trehalose using ketoses 48a–c as glycosyl acceptor. The glycosidation between gluco-derivatives did not give desired disaccharide 57aa and afforded benzyl glucoside 58 instead. That is probably due to the bulkiness at the quaternary anomeric carbon. On the other hand, the glycosidation of galacto- and manno-derivatives afforded the corresponding disaccharides 57bb and 57cc respectively, of which deprotection produced 1,1’-dimethyl analogues of trehalose 59bb and 59cc.
Thus, we achieved the stereoselective synthesis of various 1-C-methyl analogues of trehalose by utilizing the glycosidation of ketoses.
2-4 Application to Synthesis of Ulosonic Acid Derivatives
It is expected that the similar O-glycosidation of ketoses would proceed even in using other 1-C-alkyl-hexopyranoses and lead the various types of ketosides. Thus, we demonstrated the synthesis of ulosonic acid derivatives employing O-glycosidation of 1-C-vinyl-pyranose followed by ozonolysis of the olefin part.
1-C-vinyl-pyranoses 60a–c were prepared by the reaction of lactones 1a–c with vinylmagnesium bromide in good yields (Scheme 15). Under the above-mentioned conditions for 1-C-methyl-pyranose 48a–c, the O-glycosidations of 1-C-vinyl-pyranoses 60a–c with methyl glucoside 9 were carried out. The reaction was efficiently promoted by TMSOTf, and afforded corresponding disaccharides 61a–c with exclusive α-selectivity. The conversion of 1-C-vinyl-glycoside 61a–c to ulosonic acid derivatives was performed by a modification of Marshall’s method. Ozonolysis of the olefinic bond in a solution of sodium hydroxide-methanol and dichloromethane provided directly the methyl esters 62a–c (Scheme 16). In this way, the α-selective synthesis of ulosonic acid derivertives was succeeded.
3. Cycloaddition to 1-Methylenesugars
1-Methylenesugars can be also utilized for various structurally unique sugar compounds. In this section, the synthesis of spiro-sugars and their synthetic utilities are mentioned.
3-1 Synthesis of spiro-ketodisaccharides25
We mentioned the glycosidation of methylenesugars and ketoses under acidic conditions, in which the glycosidation took place α-stereoselectively and afforded α-ketosides in excellent yield. Taking adavantage of the α-selective glycosidation, we assumed that the glycosidation of 1-C-hydroxymethylpyranose in tandem manner of iterative glycosidation and intramolecular glycosidation would produce α-1,2-glycosyl-linked cyclic ketosaccharides. As various kinds of cyclodextrin have attracted greate attention, we demonstrated the stereoselective cycloglycosidation of ketose.
1-methylenesugars 2a–c were converted into hept-2-ulopyranoses 63a–c by the oxidation with N-methylmorpholine N-oxide and OsO4. The reaction proceeded in high α-stereoselectivity with producing trace β-isomers (Scheme 17). Treatment of the diol 63a with acids afforded two isomers of spiro-ketodisaccharide 64a and 65a. Various acids were scanned for the glycosidation and the effective formation of 64a and 65a was achieved with 1.5 eq. of TfOH in dichloromethane solution in a ratio of 1.1:1.0 (Table 5, entry 1). The ratio of 64a and 65a was influenced by the reaction temperature and 65a became the major product as the reaction temperature increased. When the glycosidation of 63a was performed under reflux conditions in diethyl ether using the combination of TsOH and TfONa, 64a and 65a were formed in 73% yield in a ratio of 1:7 (entry 2). The conditions at low temperature with TfOH and CaSO4 (Method A) was benefit to the formation of 64a, called kinetic control product and under the reflux conditions in diethyl ether, the thermodynamic product 65a was predominant.
Similarly, the cyclo-glycosidations of galacto- and manno-derivatives 63b and 63c were performed under the same conditions of Method A or Method B (entries 3–6). The reaction of 63a–c showed similar reactivity under the both conditions. However, the α,α-stereoselectivity 64c for 63c was higher than that in the case of 63a and 63b. Thus, the corresponding spiro-ketodisaccharides were afforded.
The compounds 64a–c and 65a–c were proved to be spiro-ketodisaccharides by mass spectral (FAB+) and NMR analyses and their structures having the α,α- and α,β- anomeric conformation were determined by instrumental analysis of the deprotected compounds 66a–c and 67a–c (Figure 4).
Furthermore, the conformation of 65a–c was confirmed finally by X-ray crystallographic analysis of the debenzylated compounds 67a–c (Figure 5). These results show that all of compounds 67a–c have a tricyclic chair-chair-chair form in thermodynamically stable conformation with the same α,β-anomeric configuration.
The structures of 64a–c were elucidated by the comparison of the three bonds carbon-proton coupling constants 3JC,H and the NOE analysis for the acetylated compounds. The 1H and 13C NMR spectra showed that the pyranose ring adopted the normal 4C1 chair form and the molecule had C2-symmetry. According to the coupling constant 3JC,H, anomeric configuration of 66a–c was revealed to be α,α-configuration. Taking account of the C2-symmetric conformation and the NOE measurement, the compounds 66a–c were confirmed to possess a chair-boat-chair form for the spiro-tricycles.
This self condensation reaction might involve the two steps of glycosidation of 63a in which α-glycosidic disaccharide is formed initially and then, the intramolecular cycloaddition takes place to give the products 64a and 65a. The formation of kinetically and thermodynamically less favorable β,β-spiroketodisaccharides were not observed. An attempt to prepare cyclic macroethers such as trimer, tetramer or more oligomers was unsuccessful in this glycosidation.
3-2 1,3-Dipolar Cycloaddition with Nitrones26
1,3-Dipolar cycloadditions of nitrons to alkenes have been widely used in organic synthesis. A stereoselective cycloaddition could be achieved by choosing an appropriate nitrone or alkene, which contains either electron-donating or electron-withdrawing substituents. We describe here the stereoselective synthesis of glycosyl spiro-isoxazolidine by the 1,3-dipolar cycloaddition of nitrone to 1-methylenesugar and the further exploration in converting the spiro-isoxazolidine to new C-glcosyl amino acid.
The nitrone 68 was readily prepared according to the Dondoni’s procedure27 by the reaction of ethyl glyoxalate with the corresponding N-benzylhydroxylamine and N-methylhydroxylamine, respectively.
We firstly examined the cycloaddition of the 1-methylenesugar 2a with the nitrone 68 under various conditions. This cycloaddition reaction in the presence of BF3•Et2O catalyst provided a mixture of α- and β-anomeric ketosyl spiro-isoxazolidines 69a and 70a with the α-isomer as predominant product (Scheme 18). This 1,3-dipolar cycloaddition proceeded diastereoselectively and gave only two isomers 69a and 70a possessing R-configuration at the C-3 position. In the presence of a catalytic amount of BF3•Et2O (0.3 eq) in dichloromethane, the cycloaddition at –78°C gave the adducts 69a and 70a in low yield and in a α,β-stereoselective ratio of 15.1:1 with the nearly 58% recovery of the starting material. The use of 1.3 eq of BF3•Et2O afforded the product 69a and 70a in 61% overall yield without remarkable change of α-streoselectivity. Moreover, increasing the reaction temperature up to 0°C after reacting at –78°C for 4 h resulted in 78% yield with satisfying α-stereoselectivity (α:β=11.3:1, Table 6, entry 1, Method A). It was found that the reactivity and selectivity were remarkably influenced by reaction temperature. In benzene solution at reflux, the reaction could efficiently proceed to afford 69a and 70a in excellent overall yield (96%) without any Lewis acid catalyst. Unfortunately, the stereoselectivity of the reaction was poor in this addition reaction (entry 2, Method B).
The 1,3-dipolar cycloaddition reactions of 2b–c to 68 were also carried out in the above-mentioned procedures; Method A: 1-methylenesugar, nitrone 68 (1.25 eq), BF3•Et2O (1.3 eq), dichloromethane, –78°C, then 0°C; Method B: benzene, reflux. The results are summarized in Table 6. The results of gluco- and galacto-derivatives were similar in yields and stereoselectivity. In the case of manno-derivative possessing axial 2-hydroxy group, the reaction exhibited an increase of β-stereoselectivity.
As another 1,3-dipolar cycloaddition, reactions with nitrile oxide were also studied. Nitrile oxide 71 was generated in situ from the corresponding ethyl chlorooximidoacetate under basic conditions and reacted with 1-methylenesugar 2a–c in dichloromethane. The cycloadducts having spiro-isoxazoline structure 72a–c were obtained in α-selectivity as a sole product. These stereoselective results are consistent with the RajanBabu’s observation.28
The 1,3-dipolar cycloaddition of 1-methylenesugar 2a–c and sugar nitrone 73 also produced the cycloadduct by refluxing in toluene. The cycloaddition of 2a afforded only two isomers 74a and 75a. Interestingly, the cycloaddition of galactose- and mannose-derivatives 2b and 2c with the nitrone 73 gave only β-isomers 75b and 75c, respectively. It should be mentioned that Lewis acids did not work as catalysts in this reaction. With the cycloadducts in hand, the reductive opening of isoxazoline ring was achieved by the treatment of 74a with zinc powder in AcOH/Ac2O. More directly, the cleavage of N-O bond and debenzylation were accomplished in one step by catalytic hydrogenation and a novel amino-C-ketosyl disaccharide 76a was obtained in 52% yield.
3-3 Synthetic Utility of spiro-Sugars
In connection with chemistry of sugar ortho esters that we developed previously, we have extended these observations to the related sugar chemistry through their conversions into other functionalities.2,29,30 Herein, an introduction of C-1 unit directly to ortho esters and their utilizations to other useful compounds were described.
Ortho ester 78 prepared from lactone 1a and neopentyl glycol 77 in 94% yield was treated with AlMe3 in dichloromethane afforded enol ether 80. By careful observation of the reaction, it is indicated that the first insertion of a methyl anion causes the cleavage of pyranose ring to afford ketal 79, and then cleavage of dioxane ring occurs by proton elimination. In the reaction with small amount of AlMe3, both ketal 79 and enol ether 80 were isolated. Actually, 79 was smoothly converted into 80 by treatment with AlMe3 (Scheme 21).
It seems to be a suitable precursor to cyclitols by chemical conversion of the resulting enol compound. We planned the development of a new method to the synthesis of cyclitols by intramolecular Aldol condensation. While Aldol condensation starting with silyl enol ethers has been well developed, there have been only a few reports on the condensation utilizing with alkyl enol ether.
The selective silyl (TBDMS) protection of 80 and consequent Swern oxidation produced the corresponding keto derivative 81. After careful investigation by using various cyclization conditions, the intramolecular Aldol condensation of 81 was achieved in catalyzing by ZnCl2 in THF-H2O (19:1) under reflux to produce cyclitol derivative 82a in 90% yield (Scheme 22). It should be noted that the addition of a small amount of H2O is essential in facilitating the condensation. For more convenient and practical synthesis of cyclitols, Aldol condensation without silyl-protection was also examined. Diol 80 was directly oxidized to keto-aldehyde 83 and succeeding Aldol condensation of 83 catalyzed by ZnCl2 in aqueous THF provided carbasugar 82a in 83% yield. Fortunately, enol ether moiety of 83 selectively reacted with ketone in the sugar part instead of terminal aldehyde after another ketone generation by hydrolysis. More practically, oxidation of 80 and succeeding cyclization of the crude oxidized product afforded 82a in 73% yield based on 80 (Scheme 22).
This procedure was applied to the conversion of galactonolactone and mannonolactone (Scheme 23). Starting from galactonolactone 1b, the conversion through ortho ester formation, insersion of C-1 unit, oxidation and intramolecular Aldol condensation provided carbasugar 82b in 64% overall yield. The structure of 82b was confirmed by X-ray single crystallographic analysis (Figure 6). In the same way, manno-carbasugar 82c was synthesized from 1c in 56% total yield. In the case of manno-derivative 82c, a small amount of stereoisomer also formed (major:minor = 10:1).
Cyclitol derivatives 82a–c are versatile synthons for the synthesis of valiolamine and its derivatives including voglibose (Figure 7). The short steps synthesis presented here may be a new practical entry for the preparation of cyclitols.
CONCLUSION
The introduction of one carbon atom to the anomeric position of pyranoses and their utilities toward various artificial sugar compounds were mentioned. The acid-catalyzed O-glycosidation of 1-methylenesugars or 1-C-methyl-pyranose produced 1’-C-methyldisaccharides in complete α-selectivity. This gycosidation was applied to the synthesis of ketoside-type artificial sugar chain, trehalose analogues, and ulosonic acid derivatives. Utilizing the synthetic property of 1-methylenesugars, the synthesis of conformationaly fixed spiro-ketosides and their conversion into sugar related compounds were achieved. The highly stereoselective synthesis of these compounds provides useful sugar mimics in synthetic and biological fields. Chemistry concerning closely related compounds to methylene-sugar was also described.
References 14
References
1. H. Takahashi, H. Kittaka, and S. Ikegami, J. Synth. Org. Chem. Japan, 2000, 58, 120; CrossRef D. Sawada, H. Takahashi, M. Shiro, and S. Ikegami, Tetrahedron Lett., 2005, 46, 2399; CrossRef D. Sawada, S. Sasayama, H. Takahashi, and S. Ikegami, Heterocycles, 2005, 66, 441; CrossRef H. Takahashi, T. Shida, Y. Hitomi, Y. Iwai, N. Miyama, K. Nishiyama, D. Sawada, and S. Ikegami, Chem. Eur. J., 2006, 12, 5861; CrossRef D. Sawada, S. Sasayama, H. Takahashi, and S. Ikegami, Tetrahedron Lett., 2006, 47, 7219; CrossRef D. Sawada, S. Sasayama, H. Takahashi, and S. Ikegami, Eur. J. Org. Chem., 2007, 1064; CrossRef D. Sawada, S. Sasayama, H. Takahashi, and S. Ikegami, Tetrahedron, 2008, 64, 8780. CrossRef
2. H. Ohtake, X. Li, H. Takahashi, and S. Ikegami, J. Synth. Org. Chem. Japan, 2002, 60, 206. CrossRef
3. C. Taillefumier and Y. Chapleur, Chem. Rev., 2004, 104, 263. CrossRef
4. L. Lay, F. Nicotra, L. Panza, G. Russo, and E. Caneva, J. Org. Chem., 1992, 57, 1304. CrossRef
5. F. K. Griffin, P. V. Murohy, D. E. Paterson, and R. J. K. Taylor, Tetrahedron Lett., 1998, 39, 8179; CrossRef M.-L. Alcaraz, F. K. Griffin, D. E. Paterson, and R. J. K. Taylor, Tetrahedron Lett., 1998, 39, 8183. CrossRef
6. M. Brockhaus and J. Lehmann, Carbohydr. Res., 1977, 53, 21; CrossRef J. Lehmann and C. Knapp, Carbohydr. Res., 1978, 63, 257; CrossRef J. M. Lancelin, J. R. Pougny, and P. Sinay, Carbohydr. Res., 1985, 136, 369. CrossRef
7. T. V. Rajan Babu and G. S. Reddy, J. Org. Chem., 1986, 51, 5458; CrossRef C. S. Wilcox, G. W. Long, and H. Suh, Tetrahedron Lett., 1984, 25, 395. CrossRef
8. C. R. Johson and B. A. Johons, Synlett, 1997, 1406; CrossRef R. Csuk and B. I. Glänzer, Tetrahedron, 1991, 47, 1655. CrossRef
9. X. Li, H. Ohtake, H. Takahashi, and S. Ikegami, Tetrahedron, 2001, 57, 4283. CrossRef
10. E. J. Hehre, C. F. Brewer, T. Uchiyama, P. Schlesselmann, and J. Lehmann, Biochemistry, 1980, 19, 3557. CrossRef
11. R. Namme, T. Mitsugi, H. Takahashi, M. Shiro, and S. Ikegami, Tetrahedron, 2006, 62, 9183. CrossRef
12. For intramolecular glycosidation, see T. Kobayashi, C. S. Regens, and S. E. Denmark, J. Synth. Org. Chem. Japan, 2008, 66, 616; CrossRef R. W. Friesen and C. F. Sturino, J. Org. Chem., 1990, 55, 5808; CrossRef E. Dubois and J. -M. Beau, Tetrahedron Lett., 1990, 31, 5165. CrossRef
13. In Advances in Carbohydrate Chemistry and Biochemistry, ed. by D. Horton, Academic, San Diego, 1995; Vol. 51, pp. 15-61.
14. The glycosidation of 1-methylenesugar 2a with 2,4,6-tri-O-benzyl-D-glycono-1,5-lactone (11) with TsOH afforded α-disaccharide 21a and a small amount of β-disaccharide 21a(β). This was the only substrate that we could obtain β-isomer. Our attempt to anomerize α-glycoside into β-glycoside was unsuccessful.
15. P. Schlesselmann, H. Fritz, J. Lehmann, T. Uchiyama, C. F. Brewer, and E. J. Hehre, Biochemistry, 1982, 21, 6606. CrossRef
16. R. Namme, T. Mitsugi, H. Takahashi, and S. Ikegami, Tetrahedron Lett., 2005, 46, 3033. CrossRef
17. X. Li, H. Ohtake, H. Takahashi, and S. Ikegami, Synlett, 2001, 1885. CrossRef
18. C. R. Parish, C. Freeman, K. J. Brown, D. J. Francis, and W. B. Cowden, Cancer Res., 1999, 59, 3433; For a review, see: L. M. Khachigian and C. R. Parish, Cardiovasc. Drug Rev., 2004, 22, 1. CrossRef
19. X. Li, H. Ohtake, H. Takahashi, and S. Ikegami, Tetrahedron, 2001, 57, 4297. CrossRef
20. T. Yamanoi, Y. Oda, I. Yamazaki, M. Shinbara, K. Morimoto, and S. Matsuda, Lett. Org. Chem., 2005, 2, 242. CrossRef
21. For example, Carbohydrate Chemistry, ed. by G.-J. Boons, Blakie Academic & Professional, London, 1998, pp. 10-16.
22. R. Namme, T. Mitsugi, H. Takahashi, and S. Ikegami, Eur. J. Org. Chem., 2007, 3758. CrossRef
23. L. M. Crowe and J. H. Crowe, Biochim. Biophys. Acta, 1988, 946, 193; CrossRef C. Colaço, S. Sen, M. Thangarelu, S. Pinder, and B. Roser, Biotechnology, 1992, 10, 1007; CrossRef C. A. L. S. Colaco, C. J. S. Smith, S. Sen, D. H. Roser, Y. Newman, S. Ring, and B. J. Roser, in Formulations and Delivery of Proteins and Peptides, ed. by J. L. Cleland and R. Langer, Symposium Series No. 567, American Chemical Society, .
24. L.-F. Tietze, R. Fischer, and H.-J. Guder, Tetrahedron Lett., 1982, 23, 4661; CrossRef E. M. Nashed and C. P. J. Glaudemans, J. Org. Chem., 1989, 54, 6116; CrossRef D. X. Qiu, F. Y. Wang, and M. S. Cai, Synth. Commun., 1989, 19, 3453; CrossRef C. Kolar and G. Kneissl, Angew. Chem. Int. Ed. Engl., 1990, 29, 809; CrossRef W. Priebe, G. Grynkiewicz, and N. Neamati, Tetrahedron Lett., 1991, 32, 2079; CrossRef T. Mukaiyama and K. Matsubara, Chem. Lett., 1992, 1041; CrossRef A. Kirschning and G.-W. Chen, Tetrahedron Lett., 1999, 40, 4665. CrossRef
25. X. Li, H. Takahashi, H. Ohtake, and S. Ikegami, Tetrahedron, 2001, 57, 8053. CrossRef
26. X. Li, H. Takahashi, H. Ohtake, and S. Ikegami, Heterocycles, 2003, 59, 547; CrossRef X. Li, H. Takahashi, H. Ohtake, and S. Ikegami, Tetrahedron Lett., 2004, 45, 4123. CrossRef
27. A. Dondoni, S. Franco. F. Junquera, P. Merino, and T. Tejero, Synth. Commun., 1994, 24, 2537. CrossRef
28. As only one example reported on the 1,3-dipolar cycloaddition, see T. V. RajanBabu and L. Toma, Carbohyd. Res., 1986, 51, 5458.
29. H. Ohtake, N. Ichiba, M. Shiro, and S. Ikegami, J. Org. Chem., 2000, 65, 8164; CrossRef H. Ohtake, N. Ichiba, and S. Ikegami, J. Org. Chem., 2000, 65, 8171. CrossRef
30. H. Ohtake and S. Ikegami, Org. Lett., 2000, 2, 457; CrossRef H. Ohtake, X. Li, M. Shiro, and S. Ikegami, Tetrahedron, 2000, 56, 7109. CrossRef