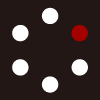
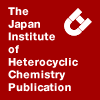
HETEROCYCLES
An International Journal for Reviews and Communications in Heterocyclic ChemistryWeb Edition ISSN: 1881-0942
Published online by The Japan Institute of Heterocyclic Chemistry
e-Journal
Full Text HTML
Received, 25th July, 2008, Accepted, 5th September, 2008, Published online, 9th September, 2008.
DOI: 10.3987/COM-08-S(F)60
■ First Synthesis of Biopterin α-D-Glucoside
Tadashi Hanaya,* Hiroki Baba, and Hiroshi Yamamoto
Graduate School of Natural Science and Technology, Okayama University, 3-1-1 Tsushima-naka, Okayama 700-8530, Japan
Abstract
A novel glycosyl donor, 4,6-di-O-acetyl-2,3-di-O-(4-methoxybenzyl)-α-D-glucopyranosy bromide (15) was efficiently prepared from D-glucose in 8 steps. The first synthesis of 2’-O-(α-D-glucopyranosyl)biopterin (2) was achieved by treatment of the key precursor, N2-(N,N-dimethylaminomethylene)-1’-O-(4-methoxybenzyl)-3-[2-(4-nitrophenyl)ethyl]biopterin (6) with 15 in the presence of silver triflate and tetramethylurea, followed by removal of the protecting groups.Some pterins having a hydroxyalkyl side-chain at C–6, a representative example being biopterin (1), have been found as glycosidic forms in certain prokaryotes; for example, 2’-O-(α-D-glucopyranosyl)biopterin (2)1-4 isolated from cyanobacteria and limipterin [2’-O-(2-acetamido-2-deoxy-β-D-glucopyranosyl)- biopterin] (3)5 isolated from a green sulfur photosynthetic bacterium. Glycosides of other pterins such as ciliapterin (L-threo-biopterin),6 neopterin,7 and 6-hydroxymethylpterin8 were also isolated from cyanobacteria, anaerobic photosynthetic bacteria, and chemoautotrophic archaebacteria. Although biopterin α-D-glucoside (2) is the most noteworthy among these pterin glycosides because of its abundant occurrence in various kinds of cyanobacteria, Anacystis nidulans,1 Oscillatoria sp.,2 Synechococcus sp.,3 and Spirulina platensis,4 there has been no report for synthesis of 2 since its first discovery in 1958.
The physiological function of the parent pterins has been studied in detail: e.g., 1 exhibits enzyme cofactor activity in aromatic amino acid hydroxylation9 and nitric oxide synthesis10 as the form of its tetrahydro derivative. By contrast, the functional roles of pterin glycosides have remained obscure, although some inhibitory activities against tyrosinase11 and photostabilization of photosynthetic pigments12 were reported for 2. Despite a considerable interest from the viewpoint of their biological activities and functions as well as structural proof of hitherto reported natural products, attempts at preparation of pterin glycosides have scarcely been made so far, except for our synthetic studies on limipterin (3) and ciliapterin glycosides.13-15 In a previous paper,14 we developed an efficient synthetic protocol for the pterin 2’-O-glycosides by way of the key intermediate, N2-(N,N-dimethylaminomethylene)-1’-O-(4-methoxybenzyl)-3-[2-(4-nitrophenyl)ethyl]biopterin (6) derived from L-rhamnose (or D-xylose) via 4 and 5 (Scheme 1). Glycosylation of 6 with tetra-O-benzoyl-α-D-glucopyranosyl bromide (7) in the presence of silver triflate afforded the 2’-O-(β-D-glucopyranosyl)biopterin derivative (8). In the present study, we therefore have undertaken to prepare an efficient glycosyl donor leading the preponderant production of pterin α-glycosides. We now describe the first synthesis of the representative, natural pterin glycoside, 2’-O-(α-D-glucopyranosyl)biopterin (2).
The stereoselective formation of the β-glycoside (8) from 6 was mainly caused by participation of the 2-O-benzoyl group of the glycosyl donor (7) through the formation of an acyloxonium ion intermediate.16 In order to avoid such a neighboring group participation, we sought to introduce an ether substituent for protection of 2-OH of a glycosyl donor. Taking into consideration the available combination of protecting groups employed for the synthetic pathway, p-methoxybenzyl (PMB) and acetyl groups were respectively chosen for protection of 2,3-OH and 4,6-OH of the glycosyl moiety.
Penta-O-acetyl-β-D-glucopyranose (9),17 derived from D-glucose, served as the starting material for preparation of methyl 4,6-di-O-acetyl-2,3-di-O-PMB-1-thio-β-D-glucopyranose (14) and its α-D-glucopyranosyl bromide derivative (15), the potential glycosyl donors for the pterin α-glycosides (Scheme 2). Treatment of 9 with thiourea and boron trifluoride etherate, followed by the action of methyl iodide and triethylamine, afforded the methyl 1-thio-β-D-glucopyranose derivative (10).18 Methanolysis of 10 in the presence of sodium methoxide and the subsequent acetalization with 2,2-dimethoxypropane in the presence of p-toluenesulfonic acid provided the 4,6-O-isopropylidene derivative (11).19 Treatment of 11 with p-methoxybenzyl chloride and sodium hydride in DMF gave the 2,3-di-O-PMB derivative (12). Hydrolysis of 12 in 70% acetic acid afforded methyl 2,3-di-O-PMB- 1-thio-β-D-glucopyranoside (13), which was then treated with acetic anhydride and pyridine to give the desired 4,6-di-O-acetyl derivative (14).20 The transformation of the thioglycoside (14) to the D-glucopyranosyl bromide (15)21 was achieved by reaction with bromine in dichloromethane in the presence of 2,6-lutidine.
Glycosylation of the 1’-O-PMB-biopterin derivative (6) with glycosyl donors (14, 15) was examined under various conditions in the presence of activators (Scheme 3). Treatment of 6 with the thioglycoside (14) in dichloromethane at room temperature in the presence of methyl triflate22 or N-iodosuccinimide-silver triflate23 as activators resulted in the formation of unidentified, decomposed compounds instead of the desired glycoside.24 While glycosylation of 6 with 4.0 mol equiv. of the glycosyl bromide (15) in dichloromethane in the presence of tetrabutylammonium bromide and N-ethyldiisopropylamine25 did not proceed, the same reaction in the presence of silver triflate (2.0 mol equiv.) and tetramethylurea (TMU)26 (1.0 mol equiv.) afforded an inseparable anomeric mixture (85:15) of the 2’-O-(α-D-glucopyranosyl)biopterin derivative (16a) and its β-anomer (16b) in 56% yield, along with the recovery of 6 (38%). The α-anomeric structure of 16a was derived from its J1,2 value (3.5 Hz) of 1H-NMR, while the larger J1,2 value (8.0 Hz) confirmed the β-form of 16a.
Separation of these isomers was achieved by removal of PMB groups and the subsequent acetylation. Thus, the mixture of 16a,b was treated with 2,3-dichloro-5,6-dicyano-1,4-benzoquinone (DDQ) in dichloromethane, followed by acetylation with acetic anhydride in pyridine, afforded the 2’-O-(2,3,4,6-tetra-O-acetyl-α-D-glucopyranosyl)biopterin derivative (17a) in 43% (total yield from 6) and its β-anomer (17b) in 8%.27
Removal of the protecting groups of 17a was carried out according to the following steps: treatment with aqueous ammonia (to cleave the N,N-dimethylaminomethylene and acetyl groups) and then with DBU28 (to cleave NPE group) furnished the desired 2’-O-(α-D-glucopyranosyl)biopterin (2) in 90% overall yield. The precise parameters obtained on 1H- and 13C-NMR spectra for 2 are listed in Table 1; the spectral data of the synthetic compound (2) were found to be essentially identical with those reported for natural product.4,11
The present work thus demonstrates the first synthesis of biopterin α-D-glucoside (2) by use of the key intermediate 1’-O-PMB-biopterin derivative (6) and the novel glycosyl donor (15) to preferentially provide an α-glycoside. Extension of this work including improvement of selectivity and yield for glycosylation as well as applications of these findings in synthesizing other natural pterin α-glycosides is in progress.
ACKNOWLEDGEMENTS
We are grateful to the SC-NMR Laboratory of Okayama University for the NMR measurements and to Okayama Foundation for Science and Technology (to T. H.) which partially supported this work.
* This paper is dedicated to Professor Emeritus Keiichiro Fukumoto on the occasion of his 75th birthday.
References
1. H. S. Forrest, C. Van Baalen, and J. Myers, Arch. Biochem. Biophys., 1958, 78, 95. CrossRef
2. T. Matsunaga, J. G. Burgess, N. Yamada, K. Komatsu, S. Yoshida, and Y. Wachi, Appl. Microbiol. Biotechnol., 1993, 39, 250.
3. Y. K. Choi, Y. K. Hwang, Y. H. Kang, and Y. S. Park, Pteridines, 2001, 12,121.
4. Y. Noguchi, A. Ishii, A. Matsushima, D. Haishi, K. Yasumuro, T. Moriguchi, T. Wada, Y. Kodera, M. Hiroto, H. Nishihara, M. Sekine, and Y. Inada, Mar. Biotechnol., 1999, 1, 207. CrossRef
5. K. W. Cha, W. Pfleiderer, and J. J. Yim, Helv. Chim. Acta, 1995, 78, 600. CrossRef
6. (a) M. Ikawa, J. J. Sasner, J. F. Haney, and T. L. Foxall, Phytochemistry, 1995, 38, 1229; CrossRef (b) S.-H. Cho, J.-U. Na, H. Youn, C.-S. Hwang, C.-H. Lee, and S.-O. Kang, Biochim. Biophys. Acta, 1998, 1379, 53.
7. (a) A. Suzuki and M. Goto, J. Biochem., 1968, 63, 798; (b) X. Lin and R. H. White, J. Bacteriol., 1988, 170, 1396.
8. H. W. Lee, C. H. Oh, A. Geyer, W. Pfleiderer, and Y. S. Park, Biochim. Biophys. Acta, 1999, 1410, 61. CrossRef
9. (a) S. Kaufman and E. E. Kaufman, ‘Folates and Pterins,’ ed. by R. Blakley and S. J. Benkovic, J. Wiley & Sons, New York, 1985, Vol. 2, pp. 251-352; (b) S. Kaufman and D. B. Fisher, ‘Molecular Mechanisms of Oxygen Activation,’ ed. by O. Hayaishi, Academic Press, New York, 1974, pp. 285-369; (c) G. R. Guroff, J. Biol. Chem., 1969, 244, 142.
10. (a) N. S. Kwon, C. F. Nathan, D. J. Stuehr, J. Biol. Chem., 1989, 264, 20496; (b) M. A. Marletta, Cell, 1994, 78, 927. CrossRef
11. Y. Wachi, S. Yoshida, K. Komatsu, and T. Matsunaga, Jpn. Patent, 05,286,989, 1993 (Chem. Abstr., 1994, 120, 161782t).
12. T. Saito, H. Ishikawa, Y. Hada, K. Fukui, Y. Kodera, A. Matsushima, and Y. Inada, Dyes Pigments, 2003, 56, 203. CrossRef
13. (a) T. Hanaya, K. Soranaka, K. Harada, H. Yamaguchi, R. Suzuki, Y. Endo, H. Yamamoto, and W. Pfleiderer, Heterocycles, 2006, 67, 299; CrossRef (b) T. Hanaya, H. Baba, H. Toyota, and H. Yamamoto, Tetrahedron, 2008, 64, 2090. CrossRef
14. T. Hanaya, H. Toyota, and H. Yamamoto, Synlett, 2006, 2075. CrossRef
15. (a) T. Hanaya, H. Baba, and H. Yamamoto, Carbohydr. Res., 2007, 342, 2159 ; CrossRef (b) T. Hanaya, H. Baba, M. Kanemoto, and H. Yamamoto, Heterocycles, 2008, 76, 635. CrossRef
16. G. Wulff and G. RÖhle, Angew. Chem., Int. Ed. Engl., 1974, 13, 157, and references cited therin. CrossRef
17. M. L. Wolfrom and A. Thompson, Methods Carbohydr. Chem., 1963, 2, 211.
18. F. M. Ibatullin, K. A. Shabalin, J. V. Jänis, and A. G. Shavva, Tetrahedron Lett., 2003, 44, 7961; use of larger amounts of methyl iodide (10 mol equiv.) and TEA (12 mol equiv.) than those reported in this lit. led to a much improved yield (89%) of 10 from 9 (lit., 57% by use of 1.1 equiv. of methyl iodide and 3.1 equiv. of TEA). CrossRef
19. All newly isolated compounds hereafter in this paper gave satisfactory analytical and 1H-NMR (mostly at 600 MHz) data, which will be presented in a full paper in the near future.
20. 1H-NMR for 14 (500 MHz, CDCl3) δ = 1.94, 2.06 (3H each, 2s, AcO-4,6), 2.22 (3H, s, MeS-1), 3.48 (1H, dd, J1,2 = 9.8, J2,3 = 8.8 Hz, H-2), 3.54 (1H, ddd, J4,5 = 10.1, J5,6a = 5.2, J5,6b = 2.4 Hz, H-5), 3.61 (1H, t, J3,4 = 9.5 Hz, H-3), 3.80 (6H, s, MeO), 4.08 (1H, dd, J6a,6b = 12.2 Hz, Hb-6), 4.20 (1H, dd, Ha-6), 4.35 (1H, d, H-1), 4.59, 4.77 (2H each, 2d, 2J = 11.0 Hz, CH2O-2 or 3), 4.67, 4.81 (2H each, 2d, 2J = 10.1 Hz, CH2O-2 or 3), 5.02 (1H, t, H-4), 6.855, 6.86, 7.18, 7.30 (2H each, 4 d, Jo,m = 8.8 Hz, C6H4).
21. 1H-NMR for 15 (500 MHz, CDCl3) δ = 1.95, 2.05 (3H each, 2s, AcO-4,6), 3.53 (1H, dd, J2,3 = 9.2, J1,2 = 3.9 Hz, H-2), 3.80, 3.81 (3H each, 2s, MeO), 3.92 (1H, t, J3,4 = 9.5 Hz, H-3), 4.02 (1H, dd, J6a,6b = 12.5, J5,6b = 2.1 Hz, Hb-6), 4.14 (1H, ddd, J4,5 = 10.4, J5,6a = 4.6, Hz, H-5), 4.26 (1H, dd, Ha-6), 4.58, 4.80 (2H each, 2d, 2J = 11.3 Hz, CH2O-2 or 3), 4.62, 4.67 (2H each, 2d, 2J = 11.6 Hz, CH2O-2 or 3), 5.05 (1H, dd, H-4), 6.26 (1H, d, H-1), 6.86, 6.88, 7.19, 7.29 (2H each, 4 d, Jo,m = 8.6 Hz, C6H4).
22. H. Lönn, Carbohydr. Res., 1985, 139, 105.
23. H. Yin, F. W. D’Souza, and T. L. Lowary, J. Org. Chem., 2002, 67, 892. CrossRef
24. In model experiments using thioglycoside (14) with 2-propanol in CH2Cl2, the corresponding glycosides were obtained in good yields: 95% (α:β = 60:40) by use of MeOTf; 91% (56:44) by use of NIS-AgOTf.
25. R. U. Lemieux, K. B. Hendriks, R. V. Stick, and K. James, J. Am. Chem. Soc., 1975, 97, 4056. CrossRef
26. S. Hanessian and J. Banoub, Methods Carbohydr. Chem., 1980, 8, 247.
27. 1H-NMR for 17a (600 MHz, CDCl3) δ 1.18 (3H, d, J2’, 3’ = 6.4 Hz, H3-3’), 1.98, 1.99, 1.995, 2.08, 2.19 (3H each, 5s, AcO-1’, AcO-2,3,4,6*), 3.16 [2H, t, 3J = 7.8 Hz, CH2CH2-N(3)], 3.19, 3.23 (3H each, 2s, Me2N), 3.83 (1H, ddd, J4,5 = 10.3, J5,6a = 4.2, J5,6b = 2.2 Hz, H-5*), 3.94 (1H, dd, J6a,6b = 12.5 Hz, Hb-6*), 4.19 (1H, dd, Ha-6*), 4.45 (1H, qd, J1’,2’ = 4.6 Hz, H-2’), 4.58, 4.60 [1H each, 2dt, 2J = 12.0 Hz, CH2-N(3)], 4.80 (1H, dd, J2,3 = 10.3, J1,2 = 3.9 Hz, H-2*), 5.00 (1H, dd, J3,4 = 9.5 Hz, H-4*), 5.22 (1H, d, H-1*), 5.33 (1H, dd, H-3*), 6.06 (1H, d, H-1’), 7.42, 8.14 (2H each, 2d, Jo,m = 8.8 Hz, C6H4), 8.86 (1H, s, CH=N-2), 8.90 (1H, s, H-7), *for glycosyl moiety. 1H-NMR for 17b (600 MHz, CDCl3) δ 1.31 (3H, d, J2’, 3’ = 6.4 Hz, H3-3’), 1.88, 1.95, 2.01, 2.09, 2.13 (3H each, 5s, AcO-1’, AcO-2,3,4,6*), 3.18 [2H, t, 3J = 7.6 Hz, CH2CH2-N(3)], 3.19, 3.235 (3H each, 2s, Me2N), 3.70 (1H, ddd, J4,5 = 10.0, J5,6a = 5.1, J5,6b = 2.4 Hz, H-5*), 4.13 (1H, dd, J6a,6b = 12.2 Hz, Hb-6*), 4.23 (1H, dd, Ha-6*), 4.54 (1H, qd, J1’,2’ = 5.9 Hz, H-2’), 4.61, 4.63 [1H each, 2dt, 2J = 12.4 Hz, CH2-N(3)], 4.66 (1H, d, J1,2 = 8.1 Hz, H-1*), 4.87 (1H, dd, J2,3 = 9.5 Hz, H-2*), 5.01 (1H, dd, J3,4 = 9.5 Hz, H-4*), 5.06 (1H, dd, H-3*), 5.94 (1H, d, H-1’), 7.43, 8.15 (2H each, 2d, Jo,m = 8.5 Hz, C6H4), 8.75 (1H, s, H-7), 8.84 (1H, s, CH=N-2), *for glycosyl moiety.
28. (a) T. Hanaya, K. Torigoe, K. Soranaka, H. Yamamoto, Q. Yao, and W. Pfleiderer, Pteridines, 1995, 6, 1; (b) F. Himmelsbach, B. S. Schulz, T. Trichtinger, R. Charubala, and W. Pfleiderer, Tetrahedron, 1984, 40, 59. CrossRef