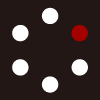
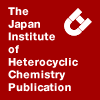
HETEROCYCLES
An International Journal for Reviews and Communications in Heterocyclic ChemistryWeb Edition ISSN: 1881-0942
Published online by The Japan Institute of Heterocyclic Chemistry
e-Journal
Full Text HTML
Received, 25th July, 2008, Accepted, 27th August, 2008, Published online, 28th August, 2008.
DOI: 10.3987/COM-08-S(F)61
■ Modified 3-Hydroxypipecolic Acid Derivatives as an Organocatalyst
Yuichi Yoshimura, Chiaki Ohara, Tatsunori Miyagawa, and Hiroki Takahata*
Tohoku Pharmaceutical University, 4-4-1 Komatsushima, Aoba-ku, Sendai 981-8558, Japan
Abstract
Novel 3-hydroxypipecolic acid derivatives were synthesized from glycine by using the method we developed previously. Among the 3-hydroxypipecolic acid derivatives obtained, a 2,3-trans-3-O-TBDPS-4,5-didehydro derivative showed the most promising result as an asymmetric catalyst in the Mannich reaction of ethyl 4-(methoxyphenylimino)acetate and n-hexylaldehyde.INTRODUCTION
The field of asymmetric organocatalysis has made rapid progress because of a strong demand for development of economical and environment-friendly catalysts.1 Proline 1, one of the earliest organocatalysts developed, has been recognized as a powerful asymmetric catalyst that is able to catalyze various reactions including aldol, 1d,2 Mannich,1d,3 Michael addition,1d,4 and Diels-Alder1d,5 reactions. Much interest has been shown in the search for new catalysts based on a proline skeleton, and many proline derivatives, e.g., 2, 3, and 4 as shown in Chart 1, have been reported.6-8
In contrast to the extensive studies on proline derivatives, there have been few reports on organocatalysis application of pipecolic acid 5, a naturally occurring homologue of proline.9 A study by Barbas revealed that pipecolic acid could act as an effective asymmetric organocatalyst for the Mannich reaction.9b We have studied the synthesis of various hydroxylated piperidine and pyrrolidine derivatives that may act as glycosidase inhibitors as transition state analogues (azasugars).10 Thus, the results of the study by Barbas prompted us to synthesize pipecolic acid derivatives and evaluate their organocatalytic functionality.
RESULTS AND DISCUSSION
As an initial organocatalyst candidate, we selected 3-hydroxy-4,5-didehydropipecolic acid 6 in which the double bond and hydroxyl moieties could potentially be used for further manipulations of the molecule. This would be an advantage for synthesizing various derivatives and effective for searching for a novel organocatalyst. To prove our concept, we envisioned the synthesis of pipecolic acid derivatives 7-10 from the common intermediate for 6 (Chart 2).
Recently, we have achieved the synthesis of all stereoisomers of 3-hydroxypipecolic acids from N-allylglycine derivative 11, obtained easily from glycine, as shown in Scheme 1.11 By this method, pipecolic acid derivatives 6, 7, and 8 were synthesized. The synthesis includes a step for lipase-catalyzed kinetic resolution of racemic 12, which has the virtue of enabling both of the enantiomers of pipecolic acid derivatives to be prepared in the same way. This will be a great advantage for catalyst preparation since either of the desirable enantiomers of the catalyst will be obtainable by this method.
The chiral building block 14 obtained from lipase resolution11 [99% ee: determined by chiral HPLC after converting to the corresponding N-tosyl derivative (data not shown)] was also used for synthesizing 3-O-TBDPS derivatives, which were selected as organocatalyst candidates designed on the basis of 3-hydroxyproline derivative 2. Compound 14 was silylated at the 3-hydroxyl group, and subsequent deprotection of the Boc group gave a tetrahydropyridine derivative 15 in 83% yield. Hydrolysis of 15 by LiOH gave a 2,3-trans-3-O-TBDPS-4,5-didehydro derivative 9 in good yield (Scheme 2). Similarly, a 2,3-trans-3-O-TBDPS derivative 10 was synthesized from 16 obtained by catalytic hydrogenation of 14.11
The pipecolic acid derivatives 6-10 thus obtained were examined for their organocatalytic functionality. First, we tested an asymmetric Mannich reaction between ethyl 4-(methoxyphenylimino)acetate 18 and n-hexylaldehyde 19 in DMSO9b using 6, 7, and 8 and compared their abilities with those of pipecolic acid 5. After an appropriate reaction time, the resulting Mannich reaction products were isolated by silica gel column chromatography and their optical purities were determined by chiral HPLC analysis.12 The results are summarized in Table 1.
The reaction using 4,5-didehydro-2,3-trans-3-hydroxypipecolic acid 6 gave Mannich products (2S,3S)-syn-20 and its anti-isomer (2S,3R)-21 in moderate yields with high ees (entry 1), which were slightly lower than those of parental pipecolic acid (entry 4). The reaction using 2,3-cis-isomer 8 gave results similar to those of the reaction using 6, with slight improvement of syn-selectivity of diastereomer formation (entry 3). The reaction of 2,3-trans-3-hydroxypipecolic acid 7 gave results comparable to those of the reaction using pipecolic acids, but the reaction yield was slightly diminished (entry 2).
Because of the low solubility of compounds 6-8, the reaction solvents to be used for Mannich reaction were limited to polar solvents like DMSO. On the other hand, compounds 9 and 10 in which a TBDPS group was installed at the 3-hydroxyl group may have a more lipophilic character than that of compounds 6-8 and could be used in less polar solvents. Thus, we tested the asymmetric Mannich reaction using 9 and 10 in 1,4-dioxane, which is often used for proline-catalyzed reaction. Although these compounds themselves could not dissolve in dioxane, the reaction mixture became clear after addition of n-hexylaldehyde 19. As a result, both 9 and 10 showed improved results in comparison with those of 6-8 (Table 2, entries 1 and 3). The use of 10 mol% 2,3-trans-3-O-TBDPS-4,5-didehydro derivative 9 as a catalyst gave a result comparable to that of 30 ml% pipecolic acid 5. In addition, 9 also showed a promising result with high ee when used in the reaction between ethyl 4-(methoxyphenylimino)acetate and isopentylaldehyde (Table 2, entry 2). It is noteworthy that a new pipecolic acid-based catalyst that could be used in a less polar solvent was obtained by the simple modification of 6 as we initially planned.
The 3-O-TBDPS derivative 9 was further examined for its usefulness as an asymmetric catalyst for the Mannich reaction in various solvents. The results are summarized in Table 3. The Mannich reaction using 9 in DMSO, THF, CH2Cl2, CH3CN and DMF gave 20 and 21 in 64-77% yields with similar ee (entries 1-5). In contrast, the use of hexane as the solvent resulted in decreases in both chemical yield and ee (entry 6). The catalyst 9 could be used in aqueous solvent conditions; however, the reaction gave Mannich products with slight decreases in ee (entry 7). The broad solvent compatibility of 9 including an aqueous solvent would be advantageous for use as an organocatalyst.
In conclusion, we have synthesized five pipecolic acid derivatives 6-10 and examined their ability as asymmetric organocatalysts of the Mannich reaction. Among the compounds obtained, a 2,3-trans-3-O-TBDPS-4,5-didehydro derivative 9 showed the most promising result. The results suggest that our initial target, 4,5-didehydro-3-hydroxypipecolic acid, is a useful scaffold for the design and development of new organocatalysts.
EXPERIMENTAL
General. Melting points are uncorrected. NMR spectra were recorded at 400 MHz (1H), 100 MHz (13C) using CDCl3, CD3OD and D2O. As an internal standard, tetramethylsilane was used for CDCl3 and CD3OD and 1,4-dioxane was used for D2O. Mass spectra were obtained by EI or FAB mode. Silica gel for chromatography was Fuji Silysia PSQ 100B. When the reagents sensitive to moisture were used, the reaction was performed under argon atmosphere.
Ethyl (2S,3S)-3-(tert-butyldiphenylsiloxy)-1,2,3,6-tetrahydropyridine-2-carboxylate (15). To a solution of 1411 (103 mg, 0.38 mmol), imidazole (39 mg, 0.57 mmol), DMAP (cat.) in CH2Cl2 (10 mL) was added TBDPSCl (118 mg, 0.46 mmol) at rt and the mixture was stirred at the same temperature for 9 h. After insoluble materials were removed by celite filtration, the filtrate was washed with brine and dried over Na2SO4. After filtration, the filtrate was concentrated and the residue was dissolved in CH2Cl2 (10 mL). To this solution was added TFA (4.5 mL, 59 mmol) and the mixture was stirred at rt for 11.5 h. After the solvents were removed under reduced pressure, the residue was dissolved in MeOH (15 mL) and the resulting solution was neutralized by NaHCO3. The insoluble materials were removed by filtration, the filtrate was concentrated. The residue was purified by silica gel column chromatography (n-hexane : AcOEt = 4 : 1) to give 15 (127 mg, 95 %, 2 steps) as a syrup. [α]D26 +58.2 ° (CHCl3, c 1.3); 1H NMR (400 MHz, CDCl3) δ 1.04 (9H, s), 1.22 (3H, t, J = 7.2 Hz), 1.84 (1H, brs), 3.26-3.37 (2H, m), 3.53 (1H, d, J = 6.3 Hz), 4.02-4.16 (2H, m), 4.49-4.50 (1H, m), 5.55 (1H, dd, J = 10.2, 2.4 Hz), 5.67 (1H, d, J = 11.6 Hz), 7.35-7.44 (6H, m), 7.66-7.72 (4H, m); 13C NMR (100 MHz, CDCl3) δ 14.0, 19.2, 26.8 (3C), 43.5, 60.9, 62.3, 67.1, 127.5, 127.6, 128.4 (2C), 128.6 (2C), 129.6 (2C), 129.7 (2C), 133.4, 134.1, 135.8, 135.8, 172.2; IR (neat): 2931.9, 2857.7, 1736.9, 1428.2, 1191.0, 1112.5, 702.8 cm-1; EI-MS (m/z): 409 (M+); HRMS Calcd for C24H31NO3Si: 409.2037. Found: 409.2065.
(2S,3S)-3-(tert-Butyldiphenylsiloxy)-1,2,3,6-tetrahydropyridine-2-carboxylic acid [(2S,3S)-3- (tert-Butyldiphenylsiloxy)-4,5-didehydropipecolic acid, 9]. To a solution of 15 (103 mg, 0.25 mmol) in MeOH (2.7 mL) and H2O (0.9 mL) was added LiOH (28 mg, 1.18 mmol) at rt and the mixture was stirred at the same temperature for 9 h. After neutralized by AcOH, the mixture was concentrated under reduced pressure. The residue was purified by silica gel column chromatography (CHCl3 : MeOH = 5 : 1) to give 9 (77 mg, 80 %) as a white solid. [α]D26 +109.8 ° (CHCl3 : MeOH = 5 : 1, c 0.1); 1H NMR (400 MHz, CDCl3 : CD3OD = 5 : 1) δ 1.11 (9H, s), 3.55 (1H, d, J = 17.4 Hz), 3.92 (1H, d, J = 2.4 Hz), 3.98 (1H, d, J = 17.4 Hz), 4.88 (1H, brs), 5.62-5.66 (1H, m), 5.72 (1H, d, J = 10.1 Hz), 7.38-7.47 (6 H, m), 7.69-7.73 (4H, m); 13C NMR (100 MHz, CDCl3 : CD3OD = 5 : 1) δ 18.9, 26.6 (3C), 39.3, 61.4, 63.6, 121.9, 127.0, 127.5 (2C), 127.7 (2C), 129.7 (2C), 129.9 (2C), 132.5, 133.2, 135.5, 135.6, 168.4; IR (KBr): 3409.4, 2932.4, 2857.7, 1635.0, 1567.5, 1112.18, 1060.8 cm-1; EI-MS (m/z): 381 (M+); HRMS Calcd for C22H27NO3Si: 381.1760. Found: 381.1764.
Ethyl (2S,3S)-3-hydroxypiperidine-2-carboxylate (16). Compound 16 (191 mg, 88%) was obtained by catalytic hydrogenation of 14 (216 mg, 0.80 mmol) as described in ref 11.
Ethyl (2S,3S)-3-(tert-butyldiphenylsiloxy)piperidine-2-carboxylate (17). Compound 17 (syrup, 341 mg, 92 % 2 steps; after purification by silica gel column chromatography: n-hexane : AcOEt = 3 : 1) was obtained from 16 (246 mg, 0.90 mmol) by the same procedure described in the synthesis of 15. [α]D21 +36.0 ° (CHCl3, c 1.0); 1H NMR (400 MHz, CDCl3) δ 1.01 (9H, s), 1.24 (3H, t, J = 7.2 Hz), 1.37-1.46 (1H, m), 1.57 (1H, dt, J = 9.7, 3.9 Hz), 1.68-1.72 (2H, m), 2.51 (1H, m), 2.90 (1H, dt, J = 13.0, 8.7 Hz), 3.33 (1H, d, J = 8.7 Hz), 3.82-3.88 (1H, m), 4.06-4.17 (2H, m), 7.34-7.44 (6H, m), 7.65-7.70 (4H, m) 13C NMR (100 MHz, CDCl3) δ 13.9, 19.1, 25.4, 26.7 (3C), 33.2, 44.7, 60.8, 66.8, 71.54, 127.4 (2C), 127.5 (2C), 129.5 (2C), 129.6 (2C), 133.4, 134.4, 135.7, 135.8, 172.6; IR (neat): 2932.8, 2857.7, 1734.1, 1428.0, 1192.4, 1111.8, 703.7 cm-1; FAB-MS (m/z) : 412 (M++1); HRMS Calcd for C24H34NO3Si: 412.2308. Found: 412.2293
(2S,3S)-3-(tert-Butyldiphenylsiloxy)pipecolic acid [(2S,3S)-3-(tert-Butyldiphenylsiloxy)pipecolic acid, 10]. Compound 10 (white solid, 151 mg, 55 % along with 28% recovery of 17; after purification by silica gel column chromatography: CHCl3 : MeOH = 5 : 1) was obtained from 17 (298 mg, 0.72 mmol) by the same procedure described in the synthesis of 9. [α]D21 −19.7 ° (CHCl3 : MeOH = 5 : 1, c 0.1); 1H NMR (400 MHz, CDCl3 : CD3OD = 5 : 1) δ 1.13 (9H, s), 1.54 (3H, brs), 2.12 (1H, brs), 3.12 (1H, d, J = 13.5 Hz), 3.29 (1H, t, J = 11.6), 3.70 (1H, s), 3.81 (1H, brs), 4.68 (1H, s), 7.38-7.47 (6 H, m), 7.65-7.70 (4H, m); IR (KBr): 3446.7, 3047.7, 2934.1, 2858.0, 1635.4, 1562.3, 1371.2, 1111.6, 1060.3, 1018.0 cm-1; EI-MS (m/z): 384 (M++1); HRMS Calcd for C22H29NO3Si: 383.1917. Found: 383.1931.
Procedures for asymmetric Mannich reactions. Following the procedure reported by Barbas et al.,9 the asymmetric Mannich reaction was done. After purification, by silica gel column chromatography, the optical purities of the products were analyzed by HPLC using a chiral column. Typically, ethyl 4-(methoxyphenylimino)acetate (103 mg, 0.5 mmol) was dissolved in a solvent (5 mL) and aldehyde (0.75 mmol) was dropwise added to the mixture at rt. After addition of catalyst (10 mol%), the mixture was stirred at room temperature. The reaction was quenched with aq. NH4Cl, and the whole mixture was extracted with AcOEt×3, then dried (Na2SO4). After filtration, the filtrate was concentrated under reduced pressure, and the residue was purified by silica gel column chromatography (n-hexane : AcOEt = 9-5 : 1).
Ethyl 3-formyl-2-(p-methoxyphenylamino)-4-methylpentanoate ((2S,3S)-20 and (2S,3R)-21). 1H-NMR (400 MHz, CDCl3): purified 1 : 0.8 mixture of diastereomers, * denotes anti diastereomer δ 1.03 (1.7H, d, J = 6.8 Hz), 1.08 (1.3H*, d, J = 6.8 Hz), 1.13 (1.3H*, d, J = 6.8 Hz), 1.17 (1.7H, d, J = 7.2 Hz), 1.22 (3H, t × 2, J = 7.2 Hz), 2.06-2.15 (0.44H*, m), 2.27-2.36 (0.55H, m), 2.53-2.62 (1H, m), 3.74 (3H, s), 3.80-3.92 (1H, m), 4.16 (2H, q×2, J = 7.2 Hz), 4.30-4.34 (1H, m), 6.66 (2H, d, J = 8.7 Hz), 6.76-6.79 (2H, m), 9.75 (0.44H, d, J = 3.4 Hz), 9.78 (0.55H, d, J = 2.9 Hz); HPLC: CHIRALPAK AS-H, hexane : i-PrOH = 99 : 1, Flow Rate 1.0 mL/min, Retention Time; syn major enantiomer = 22.9 min, syn minor enantiomer = 35.2 min, anti major enantiomer = 19.4 min, anti minor enantiomer = 31.7 min, 35 oC, 254 nm.
Ethyl 3-formyl-2-(p-methoxyphenylamino)heptanoate ((2S,3S)-22 and (2S,3R)-23). 1H-NMR (400 MHz, CDCl3): purified 1 : 0.9 mixture of diastereomers, * denotes anti diastereomer δ 0.87~0.92 (3H, m), 1.23 (1.4H*, t, J = 7.2 Hz), 1.24 (1.6H, t, J = 7.2 Hz), 1.29-1.49 (4H, m), 1.53-1.65 (1H, m), 1.68-1.75 (0.5H*, m), 1.83-1.90 (0.5H, m), 2.68-2.77 (1H, m), 3.74 (3H, s), 4.00 (1H, brs), 4.13-4.22 (2H, m), 4.26 (0.5H*, d, J = 6.3 Hz), 4.35 (0.5H, d, J = 4.8 Hz), 6.65 (2H, d, J = 9.2 Hz), 6.78 (2H, dd, J = 8.7, 1.9 Hz), 9.66 (0.5H, d, J = 2.4 Hz), 9.71 (0.5H, d, J = 1.9 Hz); HPLC: CHIRALPAK AS-H, hexane : i-PrOH = 100 : 1, Flow Rate 0.6 mL / min, Retention Time; syn major enantiomer = 45.7 min, syn minor enantiomer = 60.9 min, anti major enantiomer = 37.8 min, anti minor enantiomer = 43.7 min, 32 oC, 254 nm.
ACKNOWLEDGEMENTS
This work was supported in part by High Technology Research Program from Ministry of Education, Culture, Sports, Science and Technology of Japan.
This paper is dedicated to Professor Emeritus Keiichiro Fukumoto on the occasion of his 75th birthday.
References
1. Selected reviews for organocatalysis: (a) A. Dondoni and A. Massi, Angw. Chem. Int. Ed., 2008, 47, 4638; CrossRef (b) G. Guillena, C. Nájera, and D. J. Ramón, Tetrahedron: Asymmetry, 2007, 18, 2249; CrossRef (c) S. Mukherjee, J. W. Yang, S. Hoffmann, and B. List, Chem. Rev., 2007, 107, 5471; CrossRef (d) W. Notz, F. Tanaka, and C. F. Barabas III, Acc. Chem. Res., 2004, 37, 580; CrossRef (e) P. I. Dalko and L. Moisan, Angw. Chem. Int. Ed., 2004, 43, 5138; CrossRef (f) M. J. Gaunt, C. C. C. Johansson, A. McNally, and N. T. Vo, Drug Discov. Today, 2007, 12, 8; CrossRef (g) H. Kotsuki, H. Ikishima, and A. Okuyama, Heterocycles, 2008, 75, 493; CrossRef (h) H. Kotsuki, H. Ikishima, and A. Okuyama, Heterocycles, 2008, 75, 757. CrossRef
2. (a) B. List, R. A. Lerner, and C. F. Barabas III, J. Am. Chem. Soc., 2000, 122, 2395; CrossRef (b) K. Sakthivel, W. Notz, T. Bui, and C. F. Barabas III, J. Am. Chem. Soc., 2001, 123, 5260; CrossRef (c) W. Notz and B. List, J. Am. Chem. Soc., 2000, 122, 7386. CrossRef
3. B. List, P. Pojarliev, W. T. Biller, and H. J. Martin, J. Am. Chem. Soc., 2002, 124, 827. CrossRef
4. (a) T. Bui and C. F. Barbas III, Tetrahedron Lett., 2000, 41, 6951; CrossRef (b) B. List, Pojarliev, and H. J. Martin, Org. Lett., 2001, 3, 2423. CrossRef
5. R. Thayumanavan, B. Dhevalapally, K. Sakthivel, F. Tanaka, and C. F. Barbas III, Tetrahedron Lett., 2002, 43, 3817. CrossRef
6. K. Inomata, M. Barragué, and L. A. Paquette, J. Org. Chem., 2005, 70, 533. CrossRef
7. D. Enders, M. R. M. Hüttl, C. Grondal, and G. Raabe, Nature, 2006, 441, 861. CrossRef
8. H. Torii, M. Nakadai, K. Ishihara, S. Saito, and H. Yamamoto, Angw. Chem. Int. Ed., 2004, 43, 1983. CrossRef
9. (a) C. E. Aroyan, M. M. Vasbinder, and S. J. Miller, Org. Lett., 2005, 7, 3849; CrossRef (b) P. H.-Y. Cheong, H. Zhang, R. Thayumanavan, F. Tanaka, K. N. Houk, and C. F. Barbas III, Org. Lett., 2006, 8, 811. CrossRef
10. (a) T. Imahori, H. Ojima, H. Tateyama, Y. Mihara, and H. Takahata, Tetrahedron Lett., 2008, 49, 265; CrossRef (b) Y. Mihara, H. Ojima, T. Imahori, Y. Yoshimura, H. Ouchi, and H. Takahata, Heterocycles, 2007, 72, 633; CrossRef (c) H. Takahata, Y. Suto, E. Kato, Y. Yoshimura, and H. Ouchi, Adv. Syn. Cat., 2007, 349, 685; CrossRef (d) H. Ouchi, Y. Mihara, and H. Takahata, J. Org. Chem., 2005, 70, 5207; CrossRef (e) N. Asano, K. Ikeda, L. Yu, A. Kato, K. Takebayashi, I. Adachi, I. Kato, H. Ouchi, H. Takahata, and G. W. J. Fleet, Tetrahedron: Asymmetry, 2005, 16, 223; CrossRef (f) A. Kato, N. Kato, E. Kano, I. Adachi, K. Ikeda, L. Yu, T. Okamoto, Y. Banba, H. Ouchi, H. Takahata, and N. Asano, J. Med. Chem., 2005, 48, 2036; CrossRef (g) H. Ouchi, Y. Mihara, H. Watanabe, and H. Takahata, Tetrahedron Lett., 2004, 45, 7053; CrossRef (h) H. Takahata, Y. Banba, M. Sasatani, H. Nemoto, A. Kato, and I. Adachi, Tetrahedron, 2004, 60, 8199; CrossRef (i) H. Takahata, Y. Banba, H. Ouchi, and H. Nemoto, Org. Lett., 2003, 5, 2527; CrossRef (j) Y. Banba, C. Abe, H. Nemoto, A. Kato, I. Adachi, and H. Takahata, Tetrahedron: Asymmetry, 2001, 12, 817. CrossRef
11. (a) C. Ohara, R. Takahashi, T. Miyagawa, Y. Yoshimura, A. Kato, I. Adachi, and H. Takahata, Bioorg. Med. Chem. Lett., 2008, 18, 1810; CrossRef (b) Y. Yoshimura, C. Ohara, T. Imahori, Y. Saito, A. Kato, S. Miyauchi, I. Adachi, and H. Takahata, Bioorg. Med. Chem., 2008, in press and references for the synthesis of 3-hydroxypipecolic acids cited therein.
12. The absolute stereochemistries of the Mannich products were determined by the comparison of retention times of chiral HPLC analysis with the data shown in ref 9b.