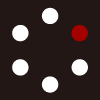
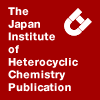
HETEROCYCLES
An International Journal for Reviews and Communications in Heterocyclic ChemistryWeb Edition ISSN: 1881-0942
Published online by The Japan Institute of Heterocyclic Chemistry
e-Journal
Full Text HTML
Received, 28th July, 2008, Accepted, 26th September, 2008, Published online, 29th September, 2008.
DOI: 10.3987/COM-08-S(F)63
■ Preparation of Unsymmetric Phthalocyanines with Benzylchalcogeno and Butoxy Groups
Takeshi Kimura,* Takeru Obonai, and Toshiharu Namauo
Center for Instrumental Analysis, Iwate University, 4-3-5 Ueda, Morioka, Iwate 020-8551, Japan
Abstract
A mixture of 4,5-dibenzylchalcogeno-3,6-diethylphthalonitrile and 4,5-dibutoxyphthalonitrile was treated with lithium in n-pentanol at 110 °C to give unsymmetric phthalocyanines. Optical and electrochemical properties of them were examined by UV-vis spectroscopy and cyclic voltammetry.Phthalocyanines have a variety of actual and/or potential applications as catalysts, optical disks, charge-generating materials, and sensitizers for photodynamic therapy.1 Although there are many procedures for preparing phthalocyanines, three approaches have been used to obtain unsymmetric ones.2-4 We recently reported the preparation of octakis(benzylchalcogeno)phthalocyanines [PcSBn and PcSeBn] and their related compounds.5a In the UV-vis spectra of PcSBn and PcSeBn, the difference of their wavelengths of the Q-band absorption is small (Δ λmax = 2 nm) although the peripheral chalcogen atoms are different each other. It is likely that the π-electronic system of symmetric phthalocyanines is not strongly affected by the peripheral benzylthio and benzylseleno groups. To estimate the influence of different chalcogen atoms, phthalocyanines with the butoxy, benzylthio, and benzylseleno groups at the β-positions were prepared, and their absorption spectra and electrochemical properties were examined by UV-vis spectroscopy and cyclic voltammetry.
Typically, phthalonitriles (1) and (2a) were mixed in a 1:1 ratio and treated with lithium in n-pentanol at 110 °C for 1 h (Scheme 1 and Table 1).5 The blue-green precipitate was purified by column chromatography to produce six phthalocyanines; 3 (16%), 4a (20%), 5a (12%), 6a (trace), 7a (trace), and PcSBn (5%). Compounds (4a), (5a), and (7a) easily dissolved in CHCl3, respectively, while the solubility of 6a was low in the solvent. By the similar reaction at 100 °C, the products were obtained in the following distribution; 3 (21%), 4a (17%), 5a (12%), 6a (8%), and 7a (14%). When the reaction was performed at 110 °C (1:2a = 5:1), 3 (27%) was obtained together with 4a (17%), 5a (17%), 6a (2%), and 7a (trace), and PcSBn could not be determined in the reaction mixture.
In the 1H NMR spectrum of 4a, the integral ratio of CH3 was 3:1 for the butyl and the ethyl groups.6 In contrast, the ratio of CH3 of 7a was observed as 1:3 for the butyl and the ethyl groups. The results suggest that 4a has three A units and one B unit while 7a has one A unit and three B units. The 1H NMR spectrum of 5a showed two triplet signals for CH2 linked to the oxygen atom and two singlet peaks for CH2 connected to the sulfur atom in which the integral ratio of two types of CH2 was 1:1. To observe the signal of 6a with 1H NMR spectroscopy, the sample was measured in CDCl3 at 55 °C. In the spectrum, two types of CH2, which are bonded to the oxygen and sulfur atoms, were observed as one triplet and one singlet peak, respectively, in a 1:1 ratio. The results reveal that 5a is the cis isomer and 6a is the trans isomer. FABMS showed the molecular ion peaks of 4a, 5a, and 7a while the structure of 6a was determined with ESIMS.6 As shown in Figure 1, UV-vis spectra of the products were measured in CHCl3 (c = 1.0 x 10-5 mol/L). The Q-bands of 3 and 5a were observed as split signals while the wavelength of the absorption of 5a (λmax = 724 nm) was longer than that of 3 (λmax = 704 nm). The split and broad absorption of 4a was found at 621, 654, 683, 702, and 721 nm, which may show the both characters of the absorption spectra of 3 and 5a. The absorption of 6a was observed as a sharp signal at 703 nm together with a weak signal at λ max = 741 nm. It seems that the Q-band absorptions of these phthalocyanines are shifted to longer wavelength when the number of the B unit is increasing. Although the λ max value of 7a was found at 715 nm, the wide Q-band should contain the absorption peak lying at around 740 nm.
To prepare phthalocyanines with two benzylseleno groups predominantly, 1 and 2b were mixed in a 6:1 ratio, which was reacted with lithium in n-pentanol at 110 °C for 2 h (Scheme 1). After purification, phthalocyanines (4b), (5b), and (6b) were obtained in low yields together with 3 (Table 1). 1H NMR of 4b, 5b, and 6b are similar to those of 4a, 5a, and 6a, respectively.7 UV-vis spectrum of 4b shows a similar absorption pattern to that of 4a. In the 77Se NMR spectrum, the signal of 4b was observed at δ = 326.2 ppm, which is a value shifted upperfield from that of PcSeBn (δ = 356.0 ppm).5a
Meanwhile, 4a was treated with lithium in THF/NH3 at –78 °C and then with elemental sulfur at room temperature.5a However, the phthalocyanine skeleton was decomposed under the reaction condition and 2,5-diethyl-3,4-trithiolophthalimide (8) was obtained in low yield.8
The redox potentials of 4a, 5a, 7a, and 4b were measured by cyclic voltammetry using Ag/AgNO3 as a reference electrode; solvent: CH2Cl2 (Table 2). The voltammogram of 4a showed one reversible, one irreversible, and one quasi-reversible peak for the oxidation potential while two reversible couples were observed for the reduction potential. Compound (7a) exhibited two reversible oxidation and two reversible reduction potentials. One reversible and one irreversible peak were observed for the oxidation potential of 4b while the reduction potential of 4b was found as one reversible and one quasi-reversible couple. It appeared that the oxidation potential of 5a is extremely lower than those of 4a, 7a, PcSBn, 4b, and PcSeBn. In addition, although the first oxidation potential of 4a is higher than that of PcSBn, the selenium derivatives (4b) and PcSeBn show the opposite results.5a
* Dedicated to Professor Emeritus Keiichiro Fukumoto on the occasion of his 75th birthday.
References
1. “The Porphyrin Handbook,” Vols. 15-20, ed. by K. M. Kadish, K. M. Smith, and R. Guilard, Academic Press, San Diego, 2003; “Phthalocyanines: Properties and Applications,” Vols. 1-4, ed. by C. C. Leznoff and A. B. P. Lever, VCH, New York, 1989-1996.
2. H. Kliesch, A. Weitemeyer, S. Müller, and D. Wöhrle, Liebigs Ann., 1995, 1269; CrossRef U. Michelsen, H. Kliesch, G. Schnurpfeil, A. K. Sobbi, and D. Wöhrle, Photochem. Photobiol., 1996, 64, 694. CrossRef
3. N. Kobayashi, R. Kondo, S. Nakajima, and T. Osa, J. Am. Chem. Soc., 1990, 112, 9640; CrossRef N. Kobayashi, T. Ishizaki, K. Ishii, and H. Konami, J. Am. Chem. Soc., 1999, 121, 9096. CrossRef
4. D. Wöhrle and G. Krawczyk, Polymer Bull., 1986, 15, 193; C. C. Leznoff, P. I. Svirskaya, B. Khouw, R. L. Cerny, P. Seymour, and A. B. P. Lever, J. Org. Chem., 1991, 56, 82. CrossRef
5. Compounds (1) and (2) were prepared by the methods described in the literature; a) T. Kimura, A. Yomogita, T. Matsutani, T. Suzuki, I. Tanaka, Y. Kawai, Y. Takaguchi, T. Wakahara, and T. Akasaka, J. Org. Chem., 2004, 69, 4716; CrossRef b) M. Kohn, J. Am. Chem. Soc., 1951, 73, 480. CrossRef
6. 4a: mp 240-242 °C; 1H NMR (400 MHz, CDCl3) δ -3.72 (s, 2H, NH), 1.20-1.26 (m, 18H, CH3), 1.78 (t, J = 7.3 Hz, 6H, CH3), 1.81-1.92 (m, 12H, CH2), 2.12-2.24 (m, 12H, CH2), 4.42-4.56 (m, 12H, OCH2), 4.45-4.52 (m, 4H, CH2), 4.63 (s, 4H, SCH2), 7.29-7.64 (m, 10H, ArH), 8.15 (s, 2H, ArH), 8.20 (s, 2H, ArH), 8.21 (s, 2H, ArH); FAB MS (m/z) 1247.6 (MH+); 5a: mp 224-226 °C; 1H NMR (400 MHz, CDCl3) δ -2.66 (s, 2H, NH), 1.09-1.21 (m, 12H, CH3), 1.70-1.84 (m, 8H, CH2), 1,93 (t, J=7.1 Hz, 12H, CH3), 2.08-2.22 (m, 8H, CH2), 4.47 (t, J = 6.6 Hz, 4H, OCH2), 4.54 (t, J = 6.5 Hz, 4H, OCH2), 4.65 (s, 4H, SCH2), 4.67 (s, 4H, SCH2), 4.78-4.93 (m, 8H, CH2), 7.20-7.61 (m, 20H, ArH), 8.30 (s, 2H, ArH), 8.55 (s, 2H, ArH); FAB MS (m/z) 1403.6 (MH+); 6a: mp 78-80 °C; 1H NMR (400 MHz, CDCl3) δ -2.02 (s, 2H, NH), 1.18 (t, J = 7.4 Hz, 12H, CH3), 1.74-1.83 (m, 8H, CH2), 1.86 (t, J = 7.3 Hz, 12H, CH3), 2.10-2.19 (m, 8H, CH2), 4.53 (s, 8H, SCH2), 4.57 (t, J = 6.5 Hz, 8H, OCH2), 4.71-4.79 (m, 8H, CH2), 7.16-7.39 (m, 12H, Ar), 7.46 (d, J = 7.2 Hz, 8H, Ar), 8.67 (s, 4H, Ar), ESIMS (m/z) 1403.68 (MH+); 7a: mp 212-214 °C; 1H NMR (400 MHz, CDCl3) δ -0.41 (s, 2H, NH), 1.17 (t, J = 7.4 Hz, 6H, CH3), 1.57 (t, J = 7.4 Hz, 12H, CH3), 1.78 (sext, J = 7.4 Hz, 4H, CH2), 1.94 (t, J = 7.3 Hz, 6H, CH3), 2.11-2.19 (m, 4H, CH2), 4.47 (s, 4H, SCH2), 4.50 (s, 8H, SCH2), 4.59 (t, J = 6.6 Hz, 4H, OCH2), 4.62-4.86 (m, 4H, CH2), 7.14-7.43 (m, 30H, ArH), 8.79 (s, 2H, Ar); FABMS (m/z) 1559.6 (MH+).
7. 4b: mp 228-231 °C; 1H NMR (400 MHz, CDCl3) δ -3.33 (br, 2H, NH), 1.18-1.30 (m, 18H, CH3), 1.76 (t, J = 7.1 Hz, 6H, CH3), 1.79-1.94 (m, 12H, CH2), 2.11-2.26 (m, 12H, CH2), 4.41-4.63 (m, 16H, OCH2, ArCH2), 4.64 (s, 4H, SCH2), 7.21-7.28 (m, 2H, ArH), 7.32 (t, J = 7.2 Hz, 4H, ArH), 7.52 (d, J = 7.2 Hz, 4H, ArH), 8.24 (s, 2H, ArH), 8.28 (s, 2H, ArH), 8.31 (s, 2H, ArH); 77Se NMR (76 MHz, CDCl3) δ 236.2; FAB MS (m/z) 1342.57 (MH+); UV-vis (CHCl3) λmax = 621, 654, 683, 702, and 721 nm