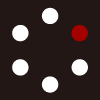
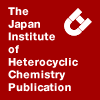
HETEROCYCLES
An International Journal for Reviews and Communications in Heterocyclic ChemistryWeb Edition ISSN: 1881-0942
Published online by The Japan Institute of Heterocyclic Chemistry
e-Journal
Full Text HTML
Received, 28th July, 2008, Accepted, 9th September, 2008, Published online, 11th September, 2008.
DOI: 10.3987/COM-08-S(F)67
■ Synthesis of Sundiversifolide and Diversifolide via a Diastereoselective [3+2] Nitrile Oxide Cycloaddition Reaction
Hiroyuki Sasaki, Hiromasa Yokoe, Mitsuru Shindo, Masahiro Yoshida, and Kozo Shishido*
Faculty of Pharmaceutical Sciences, University of Tokushima, 1-78 Sho-machi, Tokushima 770-8505, Japan
Abstract
The enantioselective synthesis of the unnatural enantiomers (-)-sundiversifolide and (+)-diversifolide has been accomplished employing a diastereoselective intramolecular [3+2] nitrile oxide cycloaddition reaction as the key step.In our previous papers, we reported the enantioselective total synthesis1 and absolute structure determination2 of an allelochemical sundiversifolide isolated from the exudate of Helianthus annuus L.3 Moreover, in our recent synthetic studies on the total synthesis of diversifolide,4 isolated from Tithonia T. diversifolia (Hernsl.) A. Gray (Compositae),5 we disclosed the error of the assigned structure and established the real structure of the natural product to be 11-epi-sundiversifolide.1 In the course of our program directed toward the search for new lead compounds for the developments of antibacterial drugs, particularly anti-MRSA drugs,6 and new allelochemicals, we decided to undertake the synthesis of the unnatural enantiomers of sundiversifolide (1), diversifolide (2), as well as a wide variety of derivatives for biological evaluation. Herein we report the synthesis of (–)-1 and (+)-2, the antipodes of the natural products, using a diastereoselective 1,3-dipolar [3+2] cycloaddition reaction7 as the key step.
Approaching the synthesis from a retrosynthetic perspective, we envisioned the following scheme: (–)-1 and (+)-2 would be derived from the bicyclic lactone 3 via the synthetic route we previously developed. The lactone 3 would be prepared from the isoxazoline 4 with the R configuration at C7 (sundiversifolide numbering), which would be made by the 1,3-dipolar [3+2] cycloaddition of the nitrile oxide 5, via a sequential reductive hydrolysis, stereoselective reduction of the C8 ketone and deoxygenation at C11. We were especially interested in determining the diasteroselectivity of the construction of the isoxazoline fused to the seven membered carbocycle during the key cycloaddition reaction.8 The nitrile oxide 5 could be generated from an appropriate precursor, potentially synthesized from the Evans aldol product 6, which is known in the literature.9 (Scheme 1)
The aldol product 6 was converted to the cyanide 7 via a standard three-step manipulation. Sequential DIBAL reduction, acetalization, debenzylation and Dess-Martin oxidation of 7 produced the aldehyde, which was subjected to the Wittig reaction to give 8 (4:1 inseparable mixture at the acetal carbon) in good overall yield. Acidic hydrolysis followed by treatment of the resulting aldehyde with hydroxylamine provided the oxime which was treated with TBSOTf to give the bis-TBS ether of alcohol and the oxime moieties. On brief exposure to a solution of TBAF in THF, selective desilylation was carried out to furnish 9. At this point in the synthesis, the isoxazoline fused to the cycloheptane by the key [3+2] cycloaddition reaction of the nitrile oxide 5, generated from the oxime 9, could now be constructed. Treatment of 9 with a 7% aqueous solution of NaOCl10 in dichloromethane at room temperature provided the diastereoisomeric isoxazolines 10 and 11 as a chromatographically separable 7:1 mixture in 71% yield. Although the stereochemistry of the cycloadducts could not be determined at this stage, the major adduct was deduced to be the desired 10 with the (R) configuration at C7 from the following mechanistic point of view. The diastereoselectivity can be explained by comparing the possible transition states T1 and T2, in which the sterically less demanding transition state T1 might be predominant and the desired compound 10 would be generated as the major product. (Scheme 2)
The isoxazoline 10 thus obtained was treated with trimethyl borate and Raney nickel (W2) in aqueous methanol11 under an atmosphere of hydrogen to give the β-hydroxy ketone 12, reduced with Zn(BH4)212 and the resulting crude mixture was treated with p-TsOH to provide the α-hydroxy lactone 13 as a 4:1 mixture of diastereoisomers at C11. Upon separation by column chromatography, the stereochemistry of the major isomer was firmly established by extensive NOESY experiments (Scheme 3). Removal of the hydroxy group in 13 was realized by reduction with samarium iodide13 to give the bicyclic lactone 3, the spectral properties of which are identical with those for the enantiomer we previously synthesized, except for the sign of the optical rotation. Conversion of 3 to (+)-2 and (–)-1 was achieved according to the procedure1 developed for the natural antipodes. Following protection of the lactone carbonyl in 3 as the acetal, desilylation and oxidation, the seven-membered ketone was obtained and submitted to Peterson olefination14 to provide 14 (4:1 inseparable mixture at the acetal carbon). This compound was exposed to the methylaluminum bis(2,6-diphenylphenoxide)-mediated carbonyl ene-reaction conditions15 using trioxane to furnish the alcohol 15. After protection of the primary hydroxy group and regeneration of the lactone moiety, the resulting 17 was treated with lithium tetramethylpiperizide and methyl iodide to give 18 diastereoselectively. The stereochemistry at C11 was determined by NOESY spectroscopy, and turned out to be the S configuration. Desilylation with TBAF produced (+)-diversifolide (2), which was subjected to kinetic protonation conditions using LDA and phenol as a proton source at –78 °C to give a chromatographically separable mixture of (–)-sundiversifolide (1) and (+)-2 in a ratio of 2:1 in 85% yield. The spectral properties of the synthetic 2 {[α]D +31.4 (c 0.70, CHCl3)} and 1 {[α]D –34.4 (c 0.51, CHCl3); lit.2 [α]D –33.7 (c 0.46, CHCl3)} were identical with those for the natural products except for the sign of optical rotations {enantiomer of 2: [α]D –30.5 (c 0.10, CHCl3)1 and [α]D –32.4 (c 0.37, CHCl3)4; enantiomer of 1: [α]D +34.0 (c 0.40, CHCl3)1}.
In summary, we have completed the enantiocontrolled total synthesis of ent-diversifolide, which is the first one reported, and ent-sundiversifolide using a diastereoselective intramolecular [3+2] nitrile oxide cycloaddition reaction as the key step. The wide variety of compounds prepared here will be submitted for biological evaluation in the search for new antibacterial drug and allelochemical leads.
ACKNOWLEDGEMENTS
This work was supported financially by a Grant-in-Aid for Scientific Research (A) (No. 16209001) from the Japan Society for the Promotion of Science.
* This paper is dedicated to Professor Emeritus Keiichiro Fukumoto on the occasion of his 75th birthday.
References
1. H. Yokoe, H. Sasaki, T. Yoshimura, M. Shindo, M. Yoshida, and K. Shishido, Org. Lett., 2007, 9, 969. CrossRef
2. K. Ohtsuki, K. Matsuo, T. Yoshikawa, C. Moriya, K. Tomita-Yokotani, K. Shishido, and M. Shindo, Org. Lett., 2008, 10, 1247. CrossRef
3. S. Ohno, K. Tomita-Yokotani, S. Kosemura, M. Node, T. Suzuki, M. Amano, K. Yasui, T. Goto, S. Yamamura, and K. Hasegawa, Phytochemistry, 2001, 56, 577. CrossRef
4. K. Matsuo, H. Yokoe, K. Shishido, and M. Shindo, Tetrahedron Lett., 2008, 49, 4279. CrossRef
5. Y.-H. Kuo and B.-Y. Lin, Chem. Pharm. Bull., 1999, 47, 428.
6. Y. Sato, H. Oketani, T. Yamada, K. Shingyouchi, T. Ohtsubo, M. Kihara, H. Shibata, and. T. Higuti, J. Pharm. Pharmacol., 1997, 49, 1042; H. Yokoe, M. Yoshida, and K. Shishido, Tetrahedron Lett., 2008, 49, 3504. CrossRef
7. For reviews, see: P. N. Confalone and E. M. Huie, Org. React., 1988, 36, 1420; V. Nair and T. D. Suja, Tetrahedron, 2007, 63, 12247. CrossRef
8. (a) O. Duclos, A. Dureault, and J. C. Depezay, Tetrahedron Lett., 1992, 33, 1059; CrossRef (b) K. Shishido, K. Umimoto, and M. Shibuya, Heterocycles, 1994, 38, 641; CrossRef (c) A. Nivlet, L. Dechoux, T. L. Gall, and C. Mioskowski, Eur. J. Org. Chem., 1999, 3251; CrossRef (d) M.-C. P. Yeh, C.-F. Jou, W.-T. Yeh, D.-Y. Chiu, and N. R. K. Reddy, Tetrahedron, 2005, 61, 493. CrossRef
9. H. Kiyota, Y. Furuya, S. Kuwahara, and T. Oritani, Biosci. Biotechnol. Biochem., 2001, 65, 2630. CrossRef
10. G. A. Lee, Synthesis, 1982, 508. CrossRef
11. D. P. Curran, J. Am. Chem. Soc., 1982, 104, 4024. CrossRef
12. T. Nakata, Y. Tani, M. Hatozaki, and T. Oishi, Chem. Pharm. Bull., 1984, 32, 1411.
13. L. A. Paquette, C. K. Seekamp, A. L. Kahane, D. G. Hilmey, and J. Gallucci, J. Org. Chem., 2004, 69, 7442. CrossRef
14. A. Vazquez and R. M. Williams, J. Org. Chem., 2000, 65, 7865. CrossRef
15. K. Maruoka, A. B. Concepcion, N. Murase, M. Oishi, N. Hirayama, and H. Yamamoto, J. Am. Chem. Soc., 1993, 115, 3943 CrossRef