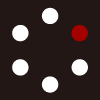
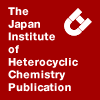
HETEROCYCLES
An International Journal for Reviews and Communications in Heterocyclic ChemistryWeb Edition ISSN: 1881-0942
Published online by The Japan Institute of Heterocyclic Chemistry
e-Journal
Full Text HTML
Received, 28th July, 2008, Accepted, 30th September, 2008, Published online, 2nd October, 2008.
DOI: 10.3987/COM-08-S(F)69
■ Construction of Cyclopentyl Carbinols from ω-Tosyloxy-1-alkenyl Boronate Esters and Grignard Reagents
Yuichi Kobayashi,* Moriteru Asano, and Yohei Kiyotsuka
Graduate School of Bioscience and Biotechnology, Tokyo Institute of Technology, 4259 Nagatsuta-cho, Midori-ku, Yokohama 226-8501, Japan
Abstract
Addition of RMgCl (R = n-Bu, Ph) to pinacol esters of 6-tosyloxy-1-alkenyl boronic acids at -78 °C gave the borates, which upon warming to room temperature underwent migration of R on boron to C(1) carbon and concomitant ring construction C-C bond formation between C(2) and C(6), eventually producing cyclopentyl alkyl (or aryl) carbinols after oxidative workup of the borane intermediates with 35% H2O2. Eight examples are presented and the reaction was applied to construction of a cyclohexyl carbinol.Esters of organoboronic acids with a diol (organoboronate esters) are chemically stable neutral compounds that are easily purified by chromatography and/or by distillation, whereas organoborates derived by reaction with organometallics are reactive anions that undergo coupling reactions with allylic acetates, alkenyl bromides, and aryl halides.1 Examples of such coupling reactions are shown in eqs (1)2,3 and (2)4,5 of Scheme 1.6 Importantly, low reactivity of the substrates due to steric reasons are compensated by the high reactivity of the borates. We then envisioned another reaction delineated in Scheme 2, in which borate B undergoes migration of R3 to the olefinic carbon and concomitant cyclization as illustrated in E to produce cyclopentyl alkyl (or aryl) carbinol D after oxidative workup of the borane intermediate C. Previously, an anion and a radical, generated at the C-L (L = I, Br) carbon of organoboranes of similar structures to A, have been shown to undergo cyclization.7,8 Herein, we report preliminary results of the migration/cyclization.
First, effect of metal cations (M+) of n-Bu-M on the proposed reaction was examined using boronate
esters 2a–d (L = OTs, OMs, Br, I) possessing a 2,2-dimethylpropane-1,3-diol ligand (examination of diol ligands is described in a later section). The esters 2a and 2b were synthesized through hydroboration of acetylenes 1a and 1b with HBBr2, hydrolysis, and subsequent esterification of the resulting boronic acid with the diol in good yields. The TsO group in 2a was replaced by halogen atoms to afford bromide 2c and iodide 2d, respectively. Addition of n-Bu-M (M = Li, ZnCl, MgCl) to 2a–d were carried out at –78 °C for 30–60 min. The resulting borates were warmed slowly to room temperature and, after stirring overnight, the products were treated with 35% H2O2 in order to produce alcohol 3. As summarized in Table 1, reaction of tosylate 2a with slightly excess n-BuLi (1.2 equiv) in THF and Et2O gave unidentified products (entries 1, 2). On the other hand, 2a and excess n-BuZnCl (6 equiv) in THF produced the tosylate of 5-hexen-1-ol (entry 3), indicating that the zinc borate was indeed formed, but remained unchanged before the aqueous work up. Next, n-BuMgCl (6 equiv) in THF was investigated
to afford the desired alcohol 3 in 69% yield after oxidative workup (entry 4). Reaction in Et2O proceeded with a comparable yield (entry 5). Use of 2.5 equiv of n-BuMgCl in THF resulted in decrease in yield (53%, entry 6). In contrast to tosylate 2a, mesitylate 2b, bromide 2c, and iodide 2d produced alcohol 3 in lower yields (entries 7–9).
Further optimization of the reaction was executed with boronate esters 2e–g, which were prepared by a method similar to 2a. Reactions with n-BuMgX (3.2 equiv) are summarized in Table 2. The pinacol ester 2g gave product 3 in good yield (entry 3), while 2e and 2f showed comparable reactivities to 2a (entries 1, 2; cf. Table 1, entry 6 with 2.5 equiv of BuMgCl). We then tested n-BuMgBr, which demonstrated no advantage over n-BuMgCl (entry 4).
The conditions developed for the migration/cyclization were applied to various cases to clarify the scope and limitations (Scheme 4). Substrates 2h–j were synthesized by hydroboration of the corresponding acetylenes with HB(c-Hex)2 followed by oxidation of the boranes with Me3NO and subsequent ligand exchange with pinacol,9 while 2k was synthesized through hydroboration with HBBr2 similar to 2a and 2g. Reaction of 2g with PhMgCl (3.2 equiv) afforded 4 in 52% yield, while lower yields were obtained with c-HexMgCl and t-BuMgCl, indicating a limitation toward steric hindrance at the reagent side (eq 3). On the other hand, bulkiness at the TsO carbon seems less sensitive as indicated by reaction of 2h with RMgCl (R = n-Bu, Ph), giving 7 and 8, respectively (eq 4). Two more examples are shown in eqs 5 and 6, in which 9 and 10 were formed in moderate to good yields. The reaction was also applicable to cyclohexane ring formation as shown in eq 7.
In summary, we have developed a new construction of cyclopentyl alkyl (or aryl) carbinols by reaction of pinacol alkenylboronate esters and RMgCl through migration of R group and cyclization. The stereochemical outcome was, however, controlled little by the existing chiral centers. We are continuing investigation to control the stereochemistry of the reaction.
ACKNOWLEDGEMENTS
This work was supported by a Grant-in-Aid for Scientific Research from the Ministry of Education, Science, Sports, and Culture, Japan.
† This paper is dedicated to Professor Emeritus Keiichiro Fukumoto on the occasion of his 75th birthday.
References
1. (a) Y. Kobayashi, ‘Modern Organonickel Chemistry,’ ed. by Y. Tamaru, Wiley-VCH, 2005, chapter 3; (b) Y. Kobayashi, ‘Latest Frontiers of Organic Synthesis,’ ed. by Y. Kobayashi, Research Signpost, Kerala, 2002, pp. 103-119.
2. (a) Y. Kobayashi, M. G. Murugesh, M. Nakano, E. Takahisa, S. B. Usmani, and T. Ainai, J. Org. Chem., 2002, 67, 7110; CrossRef (b) Y. Kobayashi, Current Org. Chem., 2003, 7, 133. CrossRef
3. (a) T. Ainai, Y.-G. Wang, Y. Tokoro, and Y. Kobayashi, J. Org. Chem., 2004, 69, 655; CrossRef (b) T. Ainai, M. Matsuumi, and Y. Kobayashi, J. Org. Chem., 2003, 68, 7825. CrossRef
4. Y. Kobayashi, Y. Nakayama, and R. Mizojiri, Tetrahedron, 1998, 54, 1053. CrossRef
5. (a) Y. Kobayashi, M. Asano, S. Yoshida, and A. Takeuchi, Org. Lett., 2005, 7, 1533; CrossRef (b) Y. Kobayashi, S. Yoshida, and Y. Nakayama, Eur. J. Org. Chem., 2001, 1873; CrossRef (c) Y. Nakayama, G. B. Kumar, and Y. Kobayashi, J. Org. Chem., 2000, 65, 707. CrossRef
6. Other reactions: (a) Y. Kobayashi, A. D. William, and R. Mizojiri, J. Organometal. Chem., 2002, 653, 91; CrossRef (b) Y. Kobayashi, Y. Tokoro, and K. Watatani, Eur. J. Org. Chem., 2000, 3825. CrossRef
7. (a) R. E. Merrill, J. L. Allen, A. Abramovitch, and E. Negishi, Tetrahedron Lett., 1977, 1019; CrossRef (b) E. J. Corey and W. L. Seibel, Tetrahedron Lett., 1986, 27, 909; CrossRef (c) M. P. Cooke, Jr. and R. K. Widener, J. Am. Chem. Soc., 1987, 109, 931; CrossRef (d) E. Negishi, T. Nguyen, L. D. Boardman, H. Sawada, J. A. Morrison, H. C. Brown, and R. B. Wetherill, Heteroatom Chem., 1992, 3, 293; CrossRef (e) M. P. Cooke, Jr., J. Org. Chem., 1992, 57, 1495. CrossRef
8. (a) E. Lee, K. Zong, H. Y. Kang, J. Lim, and J. Y. Kim, Bull. Korean Chem. Soc., 2000, 21, 765; (b) N. Guennouni, F. Lhermitte, S. Cochard, and B. Carboni, Tetrahedron, 1995, 51, 6999. CrossRef
9. A. Kamabuchi, T. Moriya, N. Miyaura, and A. Suzuki, Synth. Commun., 1993, 23, 2851 CrossRef