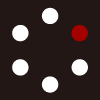
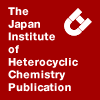
HETEROCYCLES
An International Journal for Reviews and Communications in Heterocyclic ChemistryWeb Edition ISSN: 1881-0942
Published online by The Japan Institute of Heterocyclic Chemistry
e-Journal
Full Text HTML
Received, 7th June, 2008, Accepted, 6th August, 2008, Published online, 7th August, 2008.
DOI: 10.3987/COM-08-S(F)24
■ Rhodium-Catalyzed Diastereoselective Coupling of Propargylic Oxiranes with Arylboronic Acids in Water
Masahiro Yoshida,* Maiko Hayashi, Kennosuke Matsuda, and Kozo Shishido
Graduate of School of Pharmaceutical Sciences, University of Tokushima, 1-78-1 Sho-machi, Tokushima 770-8505, Japan
Abstract
A diastereoselective coupling of propargylic oxiranes with arylboronic acids in aqueous condition is described. 4-Aryl-substituted 2,3-allenols having syn-geometries were selectively synthesized by the rhodium-catalyzed reactions in water.INTRODUCTION
Functionalized allenes are versatile building blocks for organic synthesis because of their inherent reactivity as axially chiral backbones.1 Furthermore, of the large number of natural products containing an allene moiety that have been isolated, most have axial chirality.2 For these reasons, extensive studies on the synthesis of allenes have been undertaken,1 and the SN2’-type substitution reaction of propargylic oxiranes with organometallic reagents has emerged as one of the most successful procedures to afford 2,3-allenols.3 For example, we have developed a palladium-catalyzed coupling reaction of propargylic oxiranes with arylboronic acids4 that proceeds in aqueous media to give the aryl-substituted 2,3-allenols with excellent anti-diastereoselectivity. Murakami also recently reported a similar coupling reaction using a rhodium catalyst, in which syn-configured aryl-substituted 2,3-allenols were synthesized in a highly diastereoselective manner.5 In recent years, in view of current environmental concerns, the use of organic reactions in aqueous media has increased considerably, and the development of reactions proceeding in water has become one of the most exciting research endeavors in chemistry.7 Consequently, during the course of our studies on transition metal-catalyzed reactions in aqueous media,4,6 we decided to investigate the use of water as a solvent in the rhodium-catalyzed coupling of propargylic oxiranes with arylboronic acids. Herein, we describe our results.
RESULTS AND DISCUSSION
The initial reactions were carried out using the butyl-substituted propargylic oxirane 1a.8 When 1a was treated with 5 mol % of [RhOH(cod)]2 and 2 equiv NaHCO3 in neat water at 50 ºC for 1 h, the syn- and anti-configured 4-aryl- 2,3-allenols syn-3aa and anti-3aa were produced in a 7.5:1 ratio and 55% yield (entry 1 Table 1). The stereochemistry of 3aa was determined unambiguously by NOESY correlation of the dihydrofuran 4, which was stereoselectively derived from the iodine-induced cyclization9 of syn-3aa (Scheme 1). Further attempts revealed that the presence of the water-soluble ligand TPPDS enhanced the diastereoselectivity. Thus, the reaction with TPPDS was successful and afforded the product 3aa in a 9.5:1 ratio and 57% yield (entry 2). The ratios and yields of 3aa were influenced by the reaction temperature and the reaction time (entries 3–5), and the best result was obtained by carrying out the reaction at 80 ºC for 0.5 h (syn:anti = 11:1, 58% yield in entry 4). The reaction also proceeded when other water soluble ligands, TPPMS and Cy-amphos, were used to produce 3aa in a 6.3:1 ratio in 50% yield and in an 18:1 ratio in 43% yield, respectively (entries 6 and 7).
A series of substituted arylboronic acids 2b–2e was next subjected to the reaction (Table 2). The corresponding products 3ab and 3ac were obtained with high syn-selectivities in moderate yields when the reactions of 1a with 2-methoxy- and 4-methylphenylboronic acids (2b and 2c) were carried out (entries 1 and 2). The selectivity and yield of the product 3ad were lower in the reaction with naphthalen-1-ylboronic acid (2d) (syn:anti = 9:1, 38% yield in entry 3). However, when 2-methylnaphthalen-1-ylboronic acid (2e) was employed, the corresponding product 3ae was obtained with high diastereoselectivity (entry 4).
Table 3 shows our attempts using the propargylic oxiranes 1b–1d having various substituents at the alkynyl position. When the reaction of the silyloxyethyl-substituted substrate 1b with 2a was examined, the corresponding coupled allene 3ba was produced in a 5:1 ratio in 48% yield (entry 1). The diastereoselectivity of the corresponding product 3ca was lower when the propargylic oxirane 1c having a methoxymethyl group was examined (syn:anti = 3:1, 53% yield in entry 2). In contrast, the phenyl-substituted substrate 1d reacted with 2a to deliver the coupled allene 3da with high syn-selectivity (syn:anti = >20:1, 41% yield in entry 3).
A plausible mechanism for the reaction is shown in Scheme 2. It is presumed that the (hydroxo)rhodium(I) complex 5 is the active species in this reaction, and a catalytic cycle would involve the transmetalation of the arylboronic acid to the complex 5 yielding the arylrhodium species 6 as the initial step. The coordination of the alkyne and oxiranyl oxygen of the substrate to rhodium 7, followed by regioselective insertion into the Rh-C bond would give the alkenylrhodium complex 8. Subsequent β-oxygen elimination occurs in a syn mode to open the oxirane ring. Finally, the hydrolysis of the resulting complex 9 with water affords the syn-4-aryl-substituted 2,3-allenol 3 along with the regenerated rhodium complex 5. The minor anti-substituted products would be produced by the β-oxygen elimination in anti mode.10 As the cause of the increased selectivity at the elevated temperature (entries 3–5 in Table 1), it is presumed that the isomerization of the resulting allene11 syn-3 to anti-3 could be suppressed as the result of short reaction time.
In conclusion, we have developed a coupling reaction of propargylic oxiranes with arylboronic acids in aqueous media. A variety of 4-aryl-substituted 2,3-allenols having the syn configuration were synthesized in neat water by a rhodium catalyst in combination with the water-soluble ligand TPPDS.
ACKNOWLEDGEMENTS
This study was supported in part by a Grant-in-Aid for the Encouragement of Young Scientists (B) from the Japan Society for the Promotion of Science (JSPS), Takeda Science Foundation and Astellas Foundation for Research on Medicinal Resources.
‡ This paper is dedicated to Professor Emeritus Keiichiro Fukumoto on the occasion of his 75th birthday.
References
1. For selected reviews, see: (a) J. A. Marshall, Chem. Rev., 2000, 100, 3163; CrossRef (b) R. Zimmer, C. U. Dinesh, E. Nandanan, and F. A. Khan, Chem. Rev., 2000, 100, 3067; CrossRef (c) S. Ma, Acc. Chem. Res., 2003, 36, 701; CrossRef (d) A. S. K. Hashmi, Angew. Chem. Int. Ed., 2000, 39, 3590; CrossRef (e) A. Hoffmann-Röder and N. Krause, Angew. Chem. Int. Ed., 2002, 41, 2933; CrossRef (f) N. Krause, A. Hoffmann-Röder, and J. Canisius, Synthesis, 2002, 1759; CrossRef (g) A. Hoffmann-Röder and N. Krause, Angew. Chem. Int. Ed., 2004, 43, 1196. CrossRef
2. N. Krause and A. Hoffmann-Röder, Allenic Natural Products and Pharmaceuticals. In Modern Allenic Chemistry; ed. by N. Krause and A. S. K. Hashmi, Wiley-VCH, Weinheim, 2004; p 997. CrossRef
3. (a) A. Alexakis, I. Marek, P. Mangeney, and J. F. Normant, Tetrahedron, 1991, 47, 1677; CrossRef (b) P. Vermeer, J. Meijer, C. De Graaf, and H. Schreures, Rec. Trav. Chim. Pays-Bas, 1974, 93, 46; CrossRef (c) A. C. Oehlschlager, and E. Czyzewska, Tetrahedron Lett., 1983, 24, 5587; CrossRef (d) C. R. Johnson and D. S. Dhanoa, J. Org. Chem., 1987, 52, 1885; CrossRef (e) J. A. Marshall and K. G. Pinney, J. Org. Chem., 1993, 58, 7180; CrossRef (f) F. Bertozzi, P. Crotti, F. Macchia, M. Pineschi, A. Arnold, and B. L. Feringa, Tetrahedron Lett., 1999, 40, 4893; CrossRef (g) A. Fürstner and M. Méndez, Angew. Chem. Int. Ed., 2003, 42, 5355; CrossRef (h) K. Mori, Tetrahedron, 1974, 30, 1065; CrossRef (i) M. Ito, Y. Hirata, K. Tsukida, N. Tanaka, K. Hamada, R. Hino, and T. Fujiwara, Chem. Pharm. Bull., 1988, 36, 3328; (j) A. Braumeler, W. Brade, A. Haag, and C. H. Eugster, Helv. Chim. Acta, 1990, 73, 700; CrossRef (k) H. Kleijn, J. Meijer, G. C. Overbeek, and P. Vermeer, Rec. Trav. Chim. Pays-Bas, 1982, 101, 97; CrossRef (l) J. Kjellgren, H. Sundén, and K. J. Szabó, J. Am. Chem. Soc., 2005, 127, 1787. CrossRef
4. M. Yoshida, H. Ueda, and M. Ihara, Tetrahedron Lett., 2005, 46, 6705. CrossRef
5. T. Miura, M, Shimada, S.-Y. Ku, T. Tamai, and M. Murakami, Angew. Chem. Int. Ed., 2007, 46, 7101. CrossRef
6. (a) M. Lautens and M. Yoshida, Org. Lett., 2002, 4, 123; CrossRef (b) M. Lautens and M. Yoshida, J. Org. Chem., 2003, 68, 762; CrossRef (c) M. Yoshida, T. Ishii, T. Gotou, and M. Ihara, Heterocycles, 2004, 64, 41; CrossRef (d) M. Yoshida, T. Okada, and K. Shishido, Tetrahedron, 2007, 63, 6996. CrossRef
7. C.-J. Li, Chem. Rev., 2005, 105, 3095. CrossRef
8. General procedure for the rhodium-catalyzed coupling reaction (Entry 4, Table 1). To a stirred solution of the propargylic oxirane 1a (21.2 mg, 0.119 mmol) in H2O (2 mL) were added 2-methylphenylboronic acid (2a) (32.3 mg, 0.238 mmol), NaHCO3 (20.0 mg, 0.238 mmol), [RhOH(cod)]2 (2.7 mg, 5.95 μmol) and TPPDS (10.9 mg, 23.8 μmol) at rt, and stirring was continued for 0.5 h at 80 °C. After addition of AcOEt to the reaction mixture, the mixture was stirred vigorously for 1 h at rt and then extracted with AcOEt. The combined extracts were washed with 10% NaOH aq. and brine, and the residue upon workup was chromatographed on silica gel with hexane-AcOEt (95 : 5 v/v) as eluent to give a diastereomeric mixture of arylallenes syn-3aa and anti-3aa (18.6 mg, 58%, syn : anti = 11 : 1) as a colorless oil. syn-3aa: Colorless oil; IR (neat) 3426, 2929, 1959, 1598 cm-1; 1H-NMR (400 MHz, CDCl3) δ 0.90 (t, J = 7.0 Hz, 3H), 1.32-1.43 (m, 7H), 1.61-1.68 (m, 1H), 1.74-1.79 (m, 1H), 1.74-1.79 (m, 1H), 1.96-1.99 (m, 1H), 2.06-2.13 (m, 1H), 2.26-2.34 (m, 2H), 2.36 (s, 3H), 2.44-2.48 (m, 1H), 4.09-4.11 (m, 1H), 7.12-7.21 (m, 4H); 13C-NMR (100 MHz, CDCl3) δ 14.0, 20.6, 22.3, 23.3, 26.7, 29.6, 30.1, 34.2, 35.6, 69.4, 107.6, 108.5, 125.7, 126.7, 128.1, 130.4, 135.5, 138.5, 194.2; MS (EI) m/z 270 [M+]; HRMS (EI) m/z calcd for C19H26O 270.1984 (M+), found 290.1990. anti-3aa: Colorless crystals. mp 31-32 °C; IR (neat) 3416, 2857, 1958, 1597 cm-1; 1H-NMR (400 MHz, CDCl3) δ 0.91 (3H, t, J = 7.0 Hz), 1.50-1.35 (7H, m), 1.89-1.69 (3H, m), 2.13-1.98 (2H, m), 2.33 (3H, s), 2.49-2.24 (3H, m), 3.99-3.93 (1H, m), 7.19-7.09 (4H, m); 13C-NMR (100 MHz, CDCl3) δ 14.0, 20.5, 22.4, 23.9, 26.9, 30.1, 34.0, 36.2, 69.1, 108.5, 109.4, 125.7, 126.7, 127.9, 130.3, 135.4, 138.4, 193.4; MS (EI) m/z 270 (M+); HRMS (EI) m/z calcd for C19H26O 270.1984 (M+), found 270.1972.
9. C. Schultz-Fademrecht, M. Zimmermann, R. Fröhlich, and D. Hoppe, Synlett, 2003, 13, 1969. CrossRef
10. M. Murakami and H. Igawa, Helv. Chim. Acta, 2002, 85, 4182. CrossRef
11. (a) M. Yoshida, M. Hayashi, and K. Shishido, Org. Lett., 2007, 9, 1643; CrossRef (b) M. Yoshida, M. Al-Amin, and K. Shishido, Synthesis, 2008, 1099. CrossRef