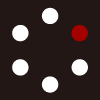
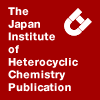
HETEROCYCLES
An International Journal for Reviews and Communications in Heterocyclic ChemistryWeb Edition ISSN: 1881-0942
Published online by The Japan Institute of Heterocyclic Chemistry
e-Journal
Full Text HTML
Received, 30th July, 2008, Accepted, 24th September, 2008, Published online, 25th September, 2008.
DOI: 10.3987/COM-08-S(F)77
■ Synthesis of Piperazine Derivatives by Cp*Ir Complex-Catalyzed N-Alkylative Reactions of Ethanolamines
Kein-ichi Fujita, Yasushi Kida, and Ryohei Yamaguchi*
Graduate School of Human and Environmental Studies, Kyoto University, Kyoto 606-8501, Japan
Abstract
A new method for the synthesis of piperazine derivatives catalyzed by a Cp*Ir complex has been developed. Cp*Ir complex-catalyzed N-alkylative homocoupling reactions of N-benzylethanolamines give N,N’-dibenzylpiperazine derivatives. For example, the reaction of N-benzylethanolamine in the presence of [Cp*IrCl2]2 (2.5 mol%) and NaHCO3 (15 mol%) in toluene at 110 °C for 17 h gives N,N’-dibenzylpiperazine in 66% yield. Cp*Ir complex-catalyzed N-alkylative cross coupling reactions of Boc-protected diethanolamines with benzylamine also give N-benzyl-N’-Boc-piperazine derivatives in moderate to good yields.INTRODUCTION
Piperazine and its derivatives are synthetically important and valuable because of their roles as indispensable structures of a variety of pharmaceutical compounds.1 For example, some of piperazine derivatives having benzyl substituent on nitrogen are known to be promising as anticancer agents or peptidomimetic drugs.1a,c Generally, synthesis of piperazines has been carried out by heterocyclization using primary amines and bis(haloalkyl)amines,2 reductive coupling of diimines,3 and reduction of keto- or diketopiperazines.4 In such reactions, formation of wasteful byproducts (hydrogen chloride or metal salt) and/or use of strong reductant can not be avoided.
Recently, we and other groups have developed atom-economical catalytic systems for carbon-nitrogen bond formation using amines and alcohols, which are based on the high catalytic performance of iridium5,6 and ruthenium6,7 complexes in hydrogen transfer reactions. In such catalytic reactions, only water is generated as a stoichiometric co-product. When this methodology is extended to the synthesis of piperazine, two strategies can be considered.8 The first one is the double N-alkylation of primary amines with diethanolamines (the left arrow in eq 1). This type of reaction was actually studied by Watanabe et al.9 and van Koten et al.10 Several N-arylpiperazines were synthesized by the catalytic system using ruthenium complexes. However, these reactions had to be conducted under harsh conditions (180 °C), and the yields of the piperazines were relatively low (<44%).
In this paper, we wish to report the synthesis of piperazines according to the second strategy (i.e. double N-alkylative homocoupling of ethanolamines, the right arrow in eq 1). By using a Cp*Ir catalyst (Cp* = pentamethylcyclopentadienyl), the reaction proceeds under relatively mild conditions (110 °C) to give a series of benzylpiperazine derivatives. We also disclose an effective catalytic system according to the first one (the left arrow in eq 1), in which use of Cp*Ir catalyst makes them possible to lower the reaction temperature and to improve the yields.
RESULTS AND DISCUSSION
First, we investigated the homocoupling reaction of N-benzylethanolamine (1a) giving N,N’-dibenzylpiperazine (2a) catalyzed by [Cp*IrCl2]2 under various conditions (Table 1). When the reaction of 1a was carried out at 110 °C for 17 h in toluene in the presence of [Cp*IrCl2]2 (2.5 mol%) as a catalyst, 2a was formed in 20% yield (entry 1). The reaction was considerably accelerated by the addition of a weak base (entries 2-5). When the reaction was carried out in the presence of 15 mol% of NaHCO3, the yield of 2a increased up to 66% (entry 2). Other weak bases, such as Na2CO3, NaOAc, and K2CO3 were also effective (entries 3-5), while stronger bases Cs2CO3 and NaOtBu were not (entries 6 and 7). When the reaction was carried out using a smaller amount of catalyst (1.0 mol%), the yield of 2a was lowered (entry 8). The reaction could be conducted under the solvent-free conditions, resulting in a slightly low yield (entry 9). The optimum reaction temperature was 110 °C: the reactions at higher (130 °C) or lower (90 °C) gave inferior results (entries 10 and 11).
On the basis of the optimization of the catalytic conditions, the homocoupling reactions of a series of N-benzylethanolamines having functional groups on aromatic ring were conducted. The results are summarized in Table 2. Methyl, methoxy, chloro, bromo, and trifluoromethyl substituents were tolerant in the present heterocyclization system to give the corresponding piperazine derivatives in moderate to good yields.11
Furthermore, we examined the cross coupling reactions of Boc-protected diethanolamines (3a-c) with benzylamine (4) on the basis of the strategy shown in the left side in eq 1. These reactions gave N-benzyl-N’-Boc-piperazine derivatives (5a-c) under relatively mild conditions (110 °C) in moderate to high yields (eq 2).
In the previous papers, we have proposed mechanisms for intermolecular N-alkylation of primary amines with alcohols,5b,g intramolecular N-alkylation of amino alcohols,5a and cycloalkylation of primary amines with diols5c,e catalyzed by a Cp*Ir complex. On the basis of those, a possible mechanism for the present N-alkylative homocoupling of ethanolamines is described in Scheme 1. The first stage of the reaction would involve an intermolecular carbon-nitrogen bond formation through hydrogen transfer processes and condensation to afford a diaminoethanol intermediate A. Subsequent intramolecular carbon-nitrogen bond formation would give a piperazine product.
In summary, we have developed a new method for the synthesis of piperazine derivatives catalyzed by a Cp*Ir complex using ethanolamines as starting materials. Furthermore, we have disclosed an effective catalytic system using Cp*Ir complex for the double N-alkylation of primary amines with diethanolamines to afford piperazines.
EXPERIMENTAL
General: All reactions and manipulations were carried out under an atmosphere of argon by means of standard Schlenk techniques. 1H and 13C NMR spectra were recorded on JEOL A-500 and EX-270 spectrometers. Gas chromatography (GC) analyses were performed on a Shimadzu GC-14A gas chromatograph and on a GL-Sciences GC353B gas chromatograph with a capillary column (Shimadzu CBP1-M25-025 or GL-Science TC-17). Column chromatography was carried out by using Wako-gel C-200. Solvents were dried by using standard procedures and distilled prior to use. The catalyst [Cp*IrCl2]2 was prepared according to the literature method.12
General procedure for the N-alkylative homocoupling of N-benzylethanolamine (1a) giving N,N’-dibenzylpiperazine (2a) catalyzed by Cp*Ir complex shown in Table 1: Under an atmosphere of argon in a heavy-walled glass reactor, 1a (1.0 mmol), [Cp*IrCl2]2 (0.025 mmol, 2.5 mol%), base (0.15 mmol, 15 mol%), and toluene (0.1 mL) were placed. The reactor was sealed, and the mixture was stirred at 110 °C for 17 h. The yield of 2a was determined by GC analysis using decane as an internal standard.
General procedure for the N-alkylative homocoupling of N-benzylethanolamines (1a-g) giving N,N’-dibenzylpiperazine derivatives (2a-g) catalyzed by Cp*Ir complex shown in Table 2: Under an atmosphere of argon in a heavy-walled glass reactor, 1 (1.0 mmol), [Cp*IrCl2]2 (0.025 mmol, 2.5 mol%), base (0.15 mmol, 15 mol%), and toluene (0.1 mL) were placed. The reactor was sealed, and the mixture was stirred at 110 °C for 17 h. After analysis by GC, the products were isolated by silica-gel column chromatography (eluent: hexane-EtOAc).
N,N’-Dibenzylpiperazine (2a):13 1H NMR (270 MHz, CDCl3): δ 7.35-7.20 (m, 10H), 3.51 (s, 4H), 2.48 (s, 8H); 13C NMR (67.8 MHz, CDCl3): δ 138.2, 129.2, 128.2, 127.0, 63.1, 53.1.
N,N’-Bis(p-methylbenzyl)piperazine (2b): 1H NMR (270 MHz, CDCl3): δ 7.19 (d, J = 8 Hz, 4H), 7.10 (d, J = 8 Hz, 4H), 3.46 (s, 4H), 2.46 (s, 8H), 2.32 (s, 6H); 13C NMR (67.8 MHz, CDCl3): δ 136.5, 135.0, 129.2, 128.8, 62.7, 53.0, 21.0. Anal. Calcd for C20H26N2: C, 81.59; H, 8.90; N, 9.51. Found: C, 81.19; H, 8.71; N, 9.15.
N,N’-Bis(o-methylbenzyl)piperazine (2c): 1H NMR (270 MHz, CDCl3):δ 7.26-7.20 (m, 2H), 7.14-7.07 (m, 6H), 3.43 (s, 4H), 2.44 (s, 8H), 2.34 (s, 6H); 13C NMR (67.8 MHz, CDCl3): δ 137.4, 136.6, 130.1, 129.7, 126.9, 125.4, 60.8, 53.3, 19.2. Anal. Calcd for C20H26N2: C, 81.59; H, 8.90; N, 9.51. Found: C, 81.48; H, 9.01; N, 9.37.
N,N’-Bis(p-methoxybenzyl)piperazine (2d): 1H NMR (270 MHz, CDCl3): δ 7.21 (d, J = 9 Hz, 4H), 6.84
(d, J = 9 Hz, 4H), 3.79 (s, 6H), 3.44 (s, 4H), 2.45 (s, 8H); 13C NMR (67.8 MHz, CDCl3): δ 158.9, 130.6, 128.5, 113.6, 61.9, 55.1, 52.1. Anal. Calcd for C20H26N2O2: C, 73.59; H, 8.03; N, 8.58. Found: C, 73.37; H, 7.99; N, 8.40.
N,N’-Bis(p-chlorobenzyl)piperazine (2e): 1H NMR (270 MHz, CDCl3): δ 7.28-7.21 (m, 8H), 3.45 (s, 4H), 2.44 (s, 8H); 13C NMR (67.8 MHz, CDCl3): δ 136.7, 132.6, 130.3, 128.3, 62.1, 52.9. Anal. Calcd for C18H20Cl2N2: C, 64.48; H, 6.01; N, 8.36. Found: C, 64.18; H, 6.04; N, 8.21.
N,N’-Bis(p-bromobenzyl)piperazine (2f): 1H NMR (270 MHz, CDCl3): δ 7.42 (d, J = 9 Hz, 4H), 7.18 (d, J = 9 Hz, 4H), 3.44 (s, 4H), 2.44 (s, 8H); 13C NMR (67.8 MHz, CDCl3): δ 137.2, 131.2, 130.7, 120.7, 62.2, 52.9. Anal. Calcd for C18H20Br2N2: C, 50.97; H, 4.75; N, 6.60. Found: C, 50.94; H, 4.67; N, 6.45.
N,N’-Bis(p-trifluoromethylbenzyl)piperazine (2g): 1H NMR (270 MHz, CDCl3):δ 7.56 (d, J = 8 Hz, 4H), 7.44 (d, J = 8 Hz, 4H), 3.56 (s, 4H), 2.48 (s, 8H); 13C NMR (67.8 MHz, CDCl3): δ 142.5, 129.3 (q, J = 32 Hz), 129.2, 125.5 (q, J = 4 Hz), 124.2 (q, J = 270 Hz), 62.4, 53.1. Anal. Calcd for C20H20F6N2: C, 59.70; H, 5.01; N, 6.96. Found: C, 59.63; H, 5.00; N, 7.02.
General procedure for the N-alkylative cross coupling of Boc-protected diethanolamines (3a-c) with benzylamine (4) giving N-benzyl-N’-Boc-piperazine derivatives (5a-c) catalyzed by Cp*Ir complex shown in Eq 2: Under an atmosphere of argon in a heavy-walled glass reactor, 3 (0.50 mmol), 4 (1.5 mmol), [Cp*IrCl2]2 (0.025 mmol, 5.0 mol%), base (0.15 mmol, 30 mol%), and toluene (0.1 mL) were placed. The reactor was sealed, and the mixture was stirred at 110 °C for 17 h. After analysis by GC, the products were isolated by silica-gel column chromatography (eluent: hexane-EtOAc).
tert-Butyl 4-benzyl-1-piperazinecarboxylate (5a):14 1H NMR (270 MHz, CDCl3): δ 7.35-7.25 (m, 5H), 3.51 (s, 2H), 3.42 (t, J = 5 Hz, 4H), 2.38 (t, J = 5 Hz, 4H), 1.45 (s, 9H); 13C NMR (67.8 MHz, CDCl3): δ 154.8, 137.8, 129.1, 128.2, 127.1, 79.5, 63.0, 52.8, 28.4.
tert-Butyl 2-methyl-4-benzyl-1-piperazinecarboxylate (5b):15 1H NMR (270 MHz, CDCl3): δ 7.33-7.24 (m, 5H), 4.18 (m, 1H), 3.80 (d, J = 13 Hz, 1H), 3.46 (m, 1H), 3.11 (m, 1H), 2.75 (d, J = 11 Hz, 1H), 2.58 (d, J = 11 Hz, 1H), 2.11 (dd, J = 11, 4 Hz, 1H), 2.00 (m, 1H), 1.47 (s, 9H), 1.24 (d, J = 7 Hz, 3H); 13C NMR (67.8 MHz, CDCl3): δ 154.8, 138.4, 128.7, 128.2, 127.0, 79.3, 62.8, 57.4, 53.2, 28.4, 15.9.
tert-Butyl 3-methyl-4-benzyl-1-piperazinecarboxylate (5c):16 1H NMR (270 MHz, CDCl3): δ 7.34-7.23 (m, 5H), 4.00 (d, J = 14 Hz, 1H), 3.67-3.62 (m, 2H), 3.18 (d, J = 14 Hz, 1H), 3.10-3.02 (m, 1H), 2.88 (br, 1H), 2.66-2.61 (m, 1H), 2.48-2.37 (m, 1H), 2.10-2.04 (m, 1H), 1.45 (s, 9H), 1.12 (d, J = 6 Hz, 3H); 13C NMR (67.8 MHz, CDCl3): δ 154.7, 138.7, 128.9, 128.2, 126.9, 79.4, 58.0, 53.1, 50.0, 28.4, 15.3.
This paper is dedicated to Professor Emeritus Keiichiro Fukumoto on the occasion of his 75th birthday.
References
1. (a) S. Liao, J. Alfaro-Lopez, M. D. Shenderovich, K. Hosohata, J. Lin, X. Li, D. Stropova, P. Davis, K. A. Jernigan, F. Porreca, H. I. Yamamura, and V. J. Hruby, J. Med. Chem., 1998, 41, 4767; CrossRef (b) D. J. Blythin, X. Chen, J. J. Piwinski, N.-Y. Shih, H.-J. Shue, J. C. Anthes, and A. T. McPhail, Bioorg. Med. Chem. Lett., 2002, 12, 3161; CrossRef (c) K. S. Putt, G. W. Chen, J. M. Pearson, J. S. Sandhorst, M. S. Hoagland, J.-T. Kwon, S.-K. Hwang, H. Jin, M. I. Churchwell, M.-H. Cho, D. R. Doerge, W. G. Helferich, and P. J. Hergenrother, Nat. Chem. Biol., 2006, 2, 543. CrossRef
2. K. G. Liu and A. J. Robichaud, Tetrahedron Lett., 2005, 46, 7921. CrossRef
3. (a) G. J. Mercer and M. S. Sigman, Org. Lett., 2003, 5, 1591; CrossRef (b) P. Vairaprakash and M. Periasamy, J. Org. Chem., 2006, 71, 3636. CrossRef
4. M. E. Jung and J. C. Rohloff, J. Org. Chem., 1985, 50, 4909. CrossRef
5. (a) K. Fujita, K. Yamamoto, and R. Yamaguchi, Org. Lett., 2002, 4, 2691; CrossRef (b) K. Fujita, Z. Li, N. Ozeki, and R. Yamaguchi, Tetrahedron Lett., 2003, 44, 2687; CrossRef (c) K. Fujita, T. Fujii, and R. Yamaguchi, Org. Lett., 2004, 6, 3525; CrossRef (d) K. Fujita and R. Yamaguchi, Synlett, 2005, 560; CrossRef (e) K. Fujita, T. Fujii, A. Komatsubara, Y. Enoki, and R. Yamaguchi, Heterocycles, 2007, 74, 673; CrossRef (f) R. Yamaguchi, S. Kawagoe, C. Asai, and K. Fujita, Org. Lett., 2008, 10, 181; CrossRef (g) K. Fujita, Y. Enoki, and R. Yamaguchi, Tetrahedron, 2008, 64, 1943. CrossRef
6. (a) G. Cami-Kobeci, P. A. Slatford, M. K. Whittlesey, and J. M. J. Williams, Bioorg. Med. Chem. Lett., 2005, 15, 535; CrossRef (b) M. H. S. A. Hamid, P. A. Slatford, and J. M. J. Williams, Adv. Synth. Catal., 2007, 349, 1555 and references cited therein. CrossRef
7. (a) T. Naota, H. Takaya, and S.-I. Murahashi, Chem. Rev., 1998, 98, 2599 and references cited therein; CrossRef (b) D. Hollmann, A. Tillack, D. Michalik, R. Jackstell, and M. Beller, Chem. Asian J., 2007, 2, 403. CrossRef
8. Quite recently, Cp*Ir-catalyzed synthesis of piperazines by the coupling of diamines with diols based on another strategy has been reported; L. U. Nordstrøm and R. Madsen, Chem. Commun., 2007, 5034. CrossRef
9. Y. Tsuji, K.-T. Huh, Y. Ohsugi, and Y. Watanabe, J. Org. Chem., 1985, 50, 1365. CrossRef
10. R. A. T. M. Abbenhuis, J. Boersma, and G. van Koten, J. Org. Chem., 1998, 63, 4282. CrossRef
11. Since any other major byproduct has not been detected by GC analyses, any oligomerization or polymerization of the reactants themselves could probably reduce the yields of the products.
12. C. White, A. Yates, and P. M. Maitlis, Inorg. Synth., 1992, 29, 228. CrossRef
13. M. Zhang, J. D. Moore, D. L. Flynn, and P. R. Hanson, Org. Lett., 2004, 6, 2657. CrossRef
14. R. Varala, S. Nuvula, and S. R. Adapa, J. Org. Chem., 2006, 71, 8283. CrossRef
15. J. W. Mickelson, K. L. Belonga, and E. J. Jacobsen, J. Org. Chem., 1995, 60, 4177. CrossRef
16. P. Maity and B. König, Org. Lett., 2008, 10, 1473. CrossRef