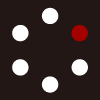
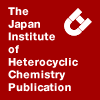
HETEROCYCLES
An International Journal for Reviews and Communications in Heterocyclic ChemistryWeb Edition ISSN: 1881-0942
Published online by The Japan Institute of Heterocyclic Chemistry
e-Journal
Full Text HTML
Received, 11th June, 2008, Accepted, 30th July, 2008, Published online, 31st July, 2008.
DOI: 10.3987/COM-08-S(F)26
■ A Transannular Diels-Alder Strategy to the Construction of the CDE Ring System of Nakiterpiosin
Hiroyoshi Takamura,* Yuji Yamagami, Tomonori Ito, Masahiro Ito, Hirokazu Arimoto, Isao Kadota, and Daisuke Uemura
Division of Chemsitry and Biochemistry, Graduate School of Natural Science and Technology, Okayama University
Abstract
The transannular Diels-Alder (TADA) reaction was applied to the synthesis of the CDE ring system of nakiterpiosin (1). TADA product 28 is a key intermediate toward the total synthesis of 1.INTRODUCTION
Coral reefs are good sources of medicines such as antimicrobial and anticancer agents.1,2 However, many reefs are now increasingly threatened due to overfishing, pollution, typhoons, and global warming. In addition, the overgrowth of other organisms in coral reefs has been recognized as another factor of their destruction.3 One of the authors (D. U.) previously reported the isolation and structural determination of nakiterpiosin (1) and nakiterpiosinone (2) from the marine sponge Terpios hosinota, which overgrew coral communities in waters off the Ryukyu Islands (Figure 1).4 Compounds 1 and 2 exhibit potent cytotoxicity against mouse lymphocytic leukemia cells (P388). However, the limited availability of 1 and 2 (1 of 0.4 mg and 2 of 0.1 mg isolated from Terpios hosinota of 30 kg) has hampered further biological studies. Thus, supply of these compounds from chemical syntheses is strongly demanded. We describe herein the results of our study toward the synthesis of 1 by a transannular Diels-Alder (TADA) strategy
which is the most useful method for the construction of the fused carbocyclic compounds.5,6
RESULTS AND DISCUSSION
Scheme 1 shows the retrosynthetic analysis of 1. The carbon framework of 1 can be broken down into the pentacyclic core 3 and C20-C26 fragment 4. Compound 3 can be synthesized from 5 through oxidative functionalization. Rings C and D of 5 can be constructed by a TADA reaction of 6. Lactone 6 would be synthesized via a Stille coupling reaction of the vinyl stannane 7 and the benzyl bromide 8.
Scheme 2 describes the preparation of the vinyl stannane 14. Treatment of (R)-(+)-glycidol (9) with TBDPSCl/imidazole gave the TBDPS ether 10. Two-carbon was elongated with (trimethylsilyl)acetylene,7 followed by removal of silyl protecting groups with TBAF to afford the diol 11. The alkyne 11 was subjected to Negishi’s carbometalation conditions8 to provide the vinyl iodide 12. The vicinal diol moiety of 12 was protected with 2,2-dimethoxypropane to give the acetonide 13. Treatment of 13 with t-BuLi, and subsequent trapping of the resulting vinyl lithium with Bu3SnCl gave the vinyl stannane 14.
Next, we examined the synthesis of the aromatic part (Scheme 3). 2-Methyl-3-nitrobenzoic acid (15) was esterified with sulfuric acid in methanol to give the methyl ester 16. Hydrogenation of the nitro functional group of 16 followed by Sandmeyer reaction with sodium nitrite and sulfuric acid provided the phenol 17. 17 was brominated with Br2 to exclusively give the 6-bromo isomer 18.9 Hydroxy group was protected with TBSCl/imidazole, then ester moiety was reduced with DIBALH to afford the benzyl alcohol 19. Protection of the alcohol 19 with DHP/PPTS gave the THP ether 20 in 70% yield from 17. After formyl moiety was introduced, subsequent four-carbon elongation was performed by the Horner-Wadsworth-Emmons reaction of 20 and triethyl 4-phosphonocrotonate 21 to provide the coupling product 22, which possesses an E,E-diene moiety. THP ether 22 was converted to the benzyl bromide 23 with CBr4/PPh3 in CH2Cl2 at 40 °C.10
With coupling precursors 14 and 23 in hand, next task was preparing the precursor of the TADA reaction. Vinyl stannane 14 and benzyl bromide 23 were connected by Stille coupling reaction to give the desired product 24 in 80% yield (Scheme 4). After the acetonide protecting group was removed with 1,3-propanedithiol and BF3·OEt2, the resulting primary hydroxy group was selectively protected with TBSCl/imidazole, and the ester moiety was reduced to give the diol 25. Chemoselective oxidation of the allylic alcohol moiety of 25 with MnO2, and subsequent Pinnic oxidation11 gave the seco-acid 26. As a
result of our investigation in macrolactonization of 26, Shiina’s protocol12 was found to be suitable for the synthesis of 27. Thus, treatment of 27 with 2-methyl-6-nitrobenzoic anhydride (MNBA) and DMAP gave the desired lactone 27, which is the precursor of the TADA reaction, in 56% yield from 25.
Next, investigation of the TADA reaction of 27 was carried out (Table 1). Cyclization did not proceed, either in refluxing toluene (entry 1) or under the conditions of 5.0 M LiClO4 in diethyl ether, reported by Grieco et al.13 (entry 2). Finally, heating in 1,2,4-trichlorobenzene at 160 °C gave the TADA products 28 and 29 in respective yields of 50% and 35%. Unfortunately, the TADA product 30, which possesses the desired stereochemistries, was not obtained.
The stereostructures of 28 and 29 were determined by 1H-1H coupling constants and NOE experiments (Figure 2). Concerning the TADA product 28, Ha-1 and Hb-1 were assigned by coupling constants (J1a,2 = 3.6 Hz and J1b,2 = 12.6 Hz). The observation of NOEs for 19-Me/Ha-1, 19-Me/H-2, and 19-Me/H-5 indicated that 19-Me, Ha-1, H-2, and H-5 were in syn orientation to each other. Based on the NOE observations for Hb-1/H-8 and H-8/H-9, these three protons were considered to be in syn relationships. Thus, the stereochemistries of 28 were determined as shown in Figure 2. The stereostructure of 29 was examined in a similar way. Based on the NOEs observed for Ha-1/H-2, Ha-1/H-8, and H-8/H-9, these protons were found to be in syn relationships. Furthermore, NOEs for 19-Me/Hb-1 and 19-Me/H-5 suggested that 19-Me, Hb-1, and H-5 were oriented in a syn arrangement. Therefore, the stereochemistries of 29 were elucidated to be as shown in Figure 2.
There is a possibility that compounds 28 and 29 could be formed by the isomerization of the C7-C8 (E)-alkene of 27 to the (Z)-alkene, followed by the TADA reaction. However, when the (Z)-alkene compound at C7-C814 was subjected to heating in 1,2,4-trichlorobenzene at 160 °C (conditions of entry 3 in Table 1), the TADA products were not obtained. Thus, reaction pathways to 28 and 29 are thought to be as described in Scheme 5. The TADA reaction of 27 proceeded through the transition states TS1 and TS2 to form the cyclized products 30 and 31. The epimerization at C8 center under the heating reaction conditions occurred to give 28 and 29.15 We are planning to convert 28 to nakiterpiosin (1) by the epimerization of C8 center.
In conclusion, we constructed the CDE ring framework of nakiterpiosin by the TADA reaction. Further studies toward the total synthesis of nakiterpiosin are underway in our laboratory.
EXPERIMENTAL
General Method. Reagents and starting materials were obtained from commercial suppliers and used without further purification. Reactions requiring anhydrous conditions were performed under an argon atmosphere. The melting point was measured with YAZAWA BY-2. The NMR spectra were recorded on JEOL JNM-EX270, JEOL JNM-AL400, and JEOL JNM-A600 (1H, 13C NMR). Chemical shifts were reported in delta units (δ) relative to chloroform (7.24), benzene (7.15). IR spectra were recorded on a JASCO FT/IR-460 plus. Optical rotations were measured by a JASCO DIP-1000. Mass spectra were measured by JEOL JMS-LG2000 (FAB) and Micromass LCT (ESI). Thin layer chromatography (TLC) was performed on Merck silica gel 60F-254 plates. Column chromatography was performed with Kanto Chemical silica gel 60N (40-100 µm, spherical, neutral) and Fuji Silysia silica gel BW-820MH.
TBDPS Ether 10. To a solution of (R)-(+)-glycidol (9) (5.00 g, 67.5 mmol) in CH2Cl2 (200 mL) were added imidazole (9.20 g, 0.14 mol) and TBDPSCl (18.4 mL, 74.3 mmol) at 0 °C. After stirring at the same temperature, the mixture was diluted with EtOAc. The organic layer was washed by satd aq. NH4Cl, H2O, and brine. Dried over MgSO4, concentration and column chromatography (hexane/EtOAc, 8:1) gave the TBDPS ether 10 (19.8 g, 94%): colorless oil; Rf = 0.65 (hexane/EtOAc, 3:1); [α]22D +4.8° (c 1.55, CH2Cl2); IR (neat) 2959 cm-1; 1H NMR (400 MHz, CDCl3) δ 7.75-7.73 (m, 4 H), 7.49-7.41 (m, 6 H), 3.90 (dd, J = 11.8, 3.1 Hz, 1 H), 3.76 (dd, J = 11.8, 4.7 Hz, 1 H), 3.18-3.14 (m, 1 H), 2.77 (t, J = 4.3 Hz, 1 H), 2.66-2.63 (m, 1 H), 1.12 (s, 9 H); 13C NMR (100 MHz, CDCl3) δ 135.5, 133.0, 129.8, 102.6, 87.1, 70.2, 66.4, 26.8, 19.3.
Diol 11. To a solution of trimethylsilylacetylene in THF (150 mL) was added n-BuLi (83 mL, 0.14 mol, 1.6 M in hexane) at –78 °C. After stirring at the same temperature for 30 min, BF3·OEt2 (16.9 mL, 0.13 mol) and a solution of 10 (19.8 g, 63.5 mmol) in THF (50 mL) were added to the mixture. The mixture was stirred at the same temperature for 2 h, then diluted with EtOAc. The organic layer was washed with satd aq. NH4Cl, H2O, and brine. Dried over MgSO4, concentration gave the corresponding crude alcohol (23.5 g). This crude alcohol was used for next reaction without further purification. To a solution of the alcohol (23.5 g) obtained above in THF (200 mL) was added TBAF (158 mL, 0.158 mol, 1.0 M in THF) at 0 °C. After stirring at the same temperature for 1 h, the mixture was diluted with EtOAc. The organic layer was washed with H2O and brine. Dried over MgSO4, concentration and column chromatography
(hexane/EtOAc, 4:1 to 1:1) gave the diol 11 (4.60 g, 72% in 2 steps): colorless oil; Rf = 0.10
(hexane/EtOAc, 1:1); [α]21D +7.2° (c 1.01, CH2Cl2); IR (CHCl3) 3366, 3291, 2940 cm-1; 1H NMR (400 MHz, CDCl3) δ 3.89 (ddd, J = 6.4, 6.4, 3.5 Hz, 1 H), 3.75 (dd, J = 11.3, 3.5 Hz, 1 H), 3.60 (dd, J = 11.3, 6.4 Hz, 1 H), 2.51 (brs, 2 H), 2.44 (dd, J = 2.7, 1.0 Hz, 1 H), 2.43 (dd, J = 2.7, 1.0 Hz, 1 H), 2.07 (t, J = 2.7 Hz, 1 H); 13C NMR (100 MHz, CDCl3) δ 80.1, 71.0, 70.1, 65.4, 23.4.
Vinyl Iodide 12. To a solution of Cp2ZrCl2 (5.84 g, 20 mmol) in CH2Cl2 (200 mL) were added AlMe3 (85 mL, 0.12 mol, 1.4 M in hexane) and a solution of 11 (4.00 g, 40.0 mmol) in CH2Cl2 (20 mL). The mixture was stirred at 50 °C for 20 h. To the mixture a solution of I2 (35.0 g, 0.14 mol) in THF (70 mL) was added at –35 °C. After stirring at the same temperature for 30 min, the mixture was diluted with EtOAc. The organic layer was washed with satd aq. potassium sodium tartrate, H2O, and brine. Dried over MgSO4, concentration and column chromatography (EtOAc) gave the vinyl iodide 12 (5.32 g, 55%): colorless oil; Rf = 0.45 (hexane/EtOAc, 1:2); [α]22D –45.4° (c 1.00, CHCl3); IR (neat) 3366, 2928, 1660 cm-1; 1H NMR (400 MHz, CDCl3) δ 6.04 (t, J = 1.2 Hz, 1 H), 3.89-3.83 (m, 1 H), 3.65 (dd, J = 11.2, 3.6 Hz, 1 H), 3.45 (dd, J = 11.2, 6.8 Hz, 1 H), 2.43-2.32 (m, 2 H), 1.88 (d, J = 1.2 Hz, 3 H); 13C NMR (100 MHz, CDCl3) δ 144.6, 70.0, 66.6, 43.7, 24.6.
Acetonide 13. To a solution of 12 (40.9 mg, 0.169 mmol) in 2,2-dimethoxypropane (1.6 mL) was added TsOH·H2O (3.2 mg, 16.9 µmol). The mixture was stirred at rt before the reaction was quenched with Et3N. Concentration and column chromatography (hexane to hexane/EtOAc, 20:1) gave the acetonide 13 (47.1 mg, 99%): colorless oil; Rf = 0.55 (hexane/EtOAc, 4:1); [α]24D +2.3° (c 1.00, CHCl3); IR (neat) 2985, 1616 cm-1; 1H NMR (400 MHz, CDCl3) δ 6.00-5.99 (m, 1 H), 4.24-4.17 (m, 1 H), 4.00 (dd, J = 8.1, 6.0 Hz, 1 H), 3.53 (dd, J = 8.1, 6.8 Hz, 1 H), 2.52 (ddd, J = 14.1, 6.8, 1.2 Hz, 1 H), 2.37 (ddd, J = 14.1, 6.0, 1.2 Hz, 1 H), 1.86 (d, J = 0.80 Hz, 3 H), 1.39 (s, 3 H), 1.33 (s, 3 H); 13C NMR (100 MHz, CDCl3) δ 144.1, 109.2, 74.0, 69.0, 43.5, 27.0, 25.7, 24.6.
Vinyl Stannane 14. To a solution of 13 (37.8 mg, 0.134 mmol) in Et2O (1.0 mL) was added t-BuLi (0.17 mL, 0.268 mmol, 1.58 M in pentane) at –78 °C. After stirring at the same temperature for 1 h, Bu3SnCl (0.11 mL, 0.402 mmol) was added. The reaction temperature was gradually raised to 0 °C for 1 h. The reaction was quenched with satd aq. NH4Cl, and the mixture was diluted with Et2O. The organic layer was separated and washed with H2O and brine. Dried over Na2SO4, concentration and column chromatography (hexane including 0.5% Et3N) gave the vinyl stannane 14 (45.8 mg, 77%): colorless oil; Rf = 0.60 (hexane/EtOAc, 10:1); [α]23D +5.6° (c 1.00, CHCl3); IR (neat) 2926, 1604 cm-1; 1H NMR (400 MHz,
CDCl3) δ 5.68 (d, J = 0.8 Hz, 1 H), 4.21-4.15 (m, 1 H), 3.87 (dd, J = 7.8, 5.9 Hz, 1 H), 3.50 (dd, J = 7.8,
7.8 Hz, 1 H), 2.51 (dd, J = 13.5, 6.6 Hz, 1 H), 2.24 (ddd, J = 13.5, 7.8, 0.8 Hz, 1 H), 1.79 (s, 3 H), 1.70-1.50 (m, 6 H), 1.45-1.30 (m, 6 H), 1.44 (s, 3 H), 1.35 (s, 3 H), 1.09-0.85 (m, 15 H); HRMS (ESI) calcd for C21H42O2SnNa (M+Na)+ 469.2108, found 469.2112.
Phenol 17. To a solution of 2-Methyl-3-nitrobenzoic acid (15) (10.0 g, 55.2 mmol) in MeOH (50 mL) was added H2SO4 (1.0 mL). The mixture was stirred at reflux for 20 h, then concentrated. To the residue was added EtOAc, the organic layer was washed with H2O and brine. Dried over Na2SO4, concentration gave the crude methyl ester 16 (10.5 g) as a pale yellow solid. This methyl ester was used for next reaction without further purification. To a solution of the above ester (10.5 g) and Pd/C (500 mg, 10%wt) in EtOH (78 mL) was stirred under H2 atmosphere at room temperature for 3 h. The catalyst was filtered off, and the filtrate was concentrated to give the crude amino ester (9.52 g) as a black oil. This amino ester was used for next reaction without further purification. To a solution of the above amino ester (9.52 g) in 5% H2SO4 (150 mL) was gradually added NaNO2 (4.19 g, 60.7 mmol) in H2O (10 mL) at 0 °C. The mixture was stirred at rt for 1 h. After stirring at reflux for 1 h, the mixture was cooled to rt. The mixture was diluted with EtOAc, then organic layer was separated and washed with brine. Dried over Na2SO4, concentration and column chromatography (hexane/EtOAc, 20:1 to 10:1 to 5:1) gave the phenol 17 (4.27 g, 47% in 3 steps): yellow crystal; mp 66-67 °C (hexane); Rf = 0.62 (hexane/EtOAc, 2:1); IR (neat) 3293, 2978 cm-1; 1H NMR (400 MHz, CDCl3) δ 7.40 (d, J = 7.8 Hz, 1 H), 7.09 (t, J = 7.8 Hz, 1 H), 6.92 (d, J = 7.8 Hz, 1 H), 5.00 (s, 1 H), 3.87 (s, 3 H), 2.44 (s, 3 H); 13C NMR (100 MHz, CDCl3) δ 168.3, 154.2, 131.8, 126.1, 125.3, 122.7, 118.3, 52.0, 12.6.
THP Ether 20. To a solution of 17 (1.70g, 10.2 mmol) in CH2Cl2 was added Br2 solution (14.9 mL, 11.2 mmol, 0.75 M in CH2Cl2) at -45 °C. After stirring at the same temperature for 2 h, the mixture was quenched with satd aq. Na2S2O3. The mixture was diluted with EtOAc, then the organic layer was separated and washed with satd aq. NaHCO3, H2O, and brine. Dried over Na2SO4, concentration gave the crude bromobenzene 18 (2.61 g) as a light brown solid. This compound was used for next reaction without further purification. To a solution of the above crude 18 (2.61 g) in DMF (50 mL) were added imidazole (1.39 g, 20.4 mmol) and TBSCl (2.31 g, 15.3 mmol) at 0 °C. The mixture was stirred at rt for 1 h. The mixture was diluted with EtOAc, then the organic layer was washed with satd aq. NH4Cl, H2O and brine. Dried over Na2SO4, concentration gave the corresponding TBS ether (3.59 g). This TBS ether was used for next reaction without further purification. To a solution of the above TBS ether (3.59 g) in CH2Cl2 (50 mL) was added DIBALH (30.0 mL, 30.6 mmol, 1.02 M in hexane) at –78 °C. After the
mixture was stirred at the same temperature for 1 h, the reaction was quenched with MeOH. The mixture was diluted with EtOAc, then the gel residue was removed with a Celite pad and washed with EtOAc. The filtrate was concentrated to give the crude alcohol 19 (3.21 g) as a pale yellow oil. This alcohol was used for next reaction without further purification. To a solution of crude 19 (3.21 g) in CH2Cl2 (50 mL) were added 3,4-dihydro-2H-pyran (1.9 mL, 20.4 mmol) and PPTS (256 mg, 1.02 mmol) at 0 °C. The mixture was stirred at rt for 2 h before the reaction was quenched with Et3N. The mixture was diluted with EtOAc, and the organic layer was separated and washed with satd aq. NaHCO3, H2O, and brine. Dried over Na2SO4, concentration and column chromatography (hexane to hexane/EtOAc, 100:1 to 20:1) gave the THP ether 20 (2.98 g, 70% in 4 steps): pale yellow oil; Rf = 0.64 (hexane/EtOAc, 4:1); IR (neat) 2932 cm-1; 1H NMR (400 MHz, CDCl3) δ 7.25 (d, J = 8.5 Hz, 1 H), 6.66 (d, J = 8.5 Hz, 1 H), 4.93 (d, J = 11.0 Hz, 1 H), 4.74 (t, J = 3.7 Hz, 1 H), 4.61 (d, J = 11.0 Hz, 1 H), 4.01-3.94 (m, 1 H), 3.63-3.52 (m, 1 H), 2.29 (s, 3 H), 1.86-1.43 (m, 6 H), 1.03-0.95 (s, 9 H), 0.19 (s, 6 H); 13C NMR (100 MHz, CDCl3) δ 153.2, 136.3, 131.7, 130.0, 119.8, 117.3, 98.6, 67.3, 62.2, 30.6, 25.8, 25.5, 19.4, 18.3, 13.3, -4.1; HRMS (ESI) calcd for C19H31BrO3SiNa (M+Na)+ 437.1124, found 437.1136.
Unsaturated Ester 22. To a solution of 20 (20.5 g, 49.5 mmol) in THF (200 mL) was added n-BuLi (38.0 mL, 59.4 mmol, 1.57 M in hexane) at –78 °C. The mixture was stirred at the same temperature for 30 min before DMF (5.74 mL, 74.3 mmol) was added. After stirring at –78 °C for 1 h, the reaction was quenched with satd aq. NH4Cl. The aqueous layer was separated and washed with Et2O. The combined organic layer was washed with H2O and brine, dried over MgSO4. Concentration gave the crude aldehyde (25.1 g) as a colorless oil. This aldehyde was used for next reaction without further purification. To a suspension of NaH (2.40 g, 59.4 mmol, 60% dispersion in mineral oil) was added triethy 4-phosphonocrotonate 21 (13.1 mL, 59.4 mmol) at 0 °C. The mixture was stirred at 0 °C for 10 min before the solution of above aldehyde (25.1 g) in THF (100 mL and 20 mL rinse) was added. The mixture was stirred at 0 °C for 2 h, then the reaction was quenched with satd aq. NH4Cl. The aqueous phase was separated and washed with EtOAc. The combined organic layer was washed with H2O and brine. Dried over MgSO4, concentration and column chromatography (hexane/EtOAc, 19:1 to 9:1 to 6:1) gave the unsaturated ester 22 (17.8 g, 78% in 2 steps): pale yellow oil; Rf = 0.51 (hexane/EtOAc, 4:1); IR (C6H6) 2929, 1713, 1623 cm-1; 1H NMR (600 MHz, C6D6) δ 7.81 (dd, J = 15.0, 11.7 Hz, 1 H), 7.45 (d, J = 15.0 Hz, 1 H), 7.16 (d, J = 8.4 Hz, 1 H), 6.74 (d, J = 8.4 Hz, 1 H), 6.61 (dd, J = 15.0, 11.7 Hz, 1 H), 6.08 (d, J = 15.0 Hz, 1 H), 4.82 (d, J = 11.4 Hz, 1 H), 4.54 (d, J = 11.4 Hz, 1 H), 4.53 (t, J = 3.0 Hz, 1 H), 4.10 (q, J = 7.2 Hz, 2 H), 3.82-3.80 (m, 1 H), 3.37-3.35 (m, 1 H), 2.39 (s, 3 H), 1.59-1.19 (m, 6 H), 1.02 (t, J = 7.2 Hz, 3 H), 1.00 (s, 9 H), 0.11 (s, 6 H); 13C NMR (150 MHz, C6D6) δ 166.7, 154.8, 145,5, 139.1, 136.7, 130.9, 131.1, 127.8, 124.9, 118.9, 97.7, 62.8, 62.1, 60.1, 30.9, 25.9, 25.8, 19.6, 14.4, 12.7, -4.2; HRMS (FAB) calcd for C26H40O5SiNa (M+Na)+ 483.2543, found 483.2522.
Benzyl Bromide 23. To a solution of 22 (1.10 g, 2.39 mmol) in CH2Cl2 (20 mL) were added CBr4 (950 mg, 2.87 mmol) and PPh3 (1.50 g, 5.74 mmol) at 0 °C. The mixture was stirred at reflux for 15 h before the reaction was quenched with satd aq. NaHCO3. The aqueous layer was separated and washed with Et2O. The combined organic layer was washed with H2O and brine. Dried over MgSO4, concentration and column chromatography (hexane/EtOAc, 20:1) gave the benzyl bromide 23 (930 mg, 86%): colorless oil; Rf = 0.58 (hexane/EtOAc, 4:1); IR (neat) 2930, 1710, 1625 cm-1; 1H NMR (400 MHz, C6D6) δ 7.68 (ddd, J = 15.2, 11.2, 0.7 Hz, 1 H), 7.00 (d, J = 15.2 Hz, 1 H), 6.98 (d, J = 8.5 Hz, 1 H), 6.63 (d, J = 8.5 Hz, 1 H), 6.50 (ddd, J = 15.2, 11.2, 0.5 Hz, 1 H), 6.04 (d, J = 15.2 Hz, 1 H), 4.13 (q, J = 7.1 Hz, 2 H), 4.11 (s, 2 H), 2.17 (s, 3 H), 1.5 (t, J = 7.1 Hz, 3 H), 0.98 (s, 9 H), 0.08 (s, 6 H); 13C NMR (100 MHz, C6D6) δ 166.5, 154.8, 144.9, 136.9, 135.9, 129.5, 128.8, 127.9, 125.3,, 121.8, 119.4, 60.3, 28.6, 26.0, 18.5, 14.6, 12.2, -4.1; HRMS (ESI) calcd for C42H62Br2O6Si2Na (2M+Na)+ 901.2335, found 901.2327.
Stille Coupling Product 24. To a solution of 14 (97.5 mg, 0.218 mmol) and 23 (80.0 mg, 0.182 mmol) in DMF (5.0 mL) were added Pd(dba)2 (110 mg, 0.191 mmol) and PPh3 (112 mg, 0.427 mmol). The mixture was stirred at 30 °C for 2 h. Concentration and column chromatography (hexane/EtOAc, 19:1) gave the coupling product 24 (74.9 mg, 80%): colorless oil; Rf = 0.45 (hexane/EtOAc, 4:1); [α]23D –6.2° (c 0.50, CHCl3); IR (neat) 2931, 1624 cm-1; 1H NMR (400 MHz, C6D6) δ 7.75 (dd, J = 15.1, 11.1 Hz, 1 H), 7.16 (d, J = 8.5 Hz, 1 H), 7.05 (d, J = 15.1 Hz, 1 H), 6.71 (d, J = 8.5 Hz, 1 H), 6.58 (dd, J = 15.1, 11.1 Hz, 1 H), 6.07 (d, J = 15.1 Hz, 1 H), 5.00 (t, J = 6.6 Hz, 1 H), 4.10 (q, J = 7.1 Hz, 2 H), 4.02-3.95 (m, 1 H), 3.72 (dd, J = 7.8, 6.0 Hz, 1 H), 3.32 (t, J = 7.8 Hz, 1 H), 3.25 (d, J = 6.0 Hz, 2 H), 2.22-2.12 (m, 1 H), 2.09 (s, 3 H), 1.90 (dd, J = 13.2, 5.6 Hz, 1 H), 1.62 (s, 3 H), 1.38 (s, 3 H), 1.31 (s, 3 H), 1.03 (t, J = 7.1 Hz, 3 H), 1.03 (s, 9 H), 0.13 (s, 6 H); 13C NMR (100 MHz, C6D6) δ 166.7, 155.0, 145.4, 140.8, 139.2, 132.7, 129.1, 127.9, 126.6, 125.2, 125.1, 120.8, 117.3, 109.0, 72.3, 69.5, 60.2, 43.9, 29.2, 27.4, 26.1, 18.6, 17.1, 14.6, 12.9, -4.0; HRMS (ESI) calcd for C30H46O5SiNa (M+Na)+ 537.3012, found 537.3008.
Diol 25. To a solution of 24 (85.0 mg, 0.170 mmol) in CH2Cl2 (3.0 mL) were added 1,3-propanedithiol (0.02 mL, 0.181 mmol) and BF3·OEt2 (0.024 mL, 0.181 mmol) at –78 °C. The mixture was stirred at the same temperature for 2 h before the reaction was quenched with satd aq. NaHCO3. To the mixture EtOAc was added, then organic layer was separated and washed with H2O and brine. Dried over MgSO4, concentration and column chromatography (hexane/EtOAc, 4:1) gave the corresponding diol (66.0 mg,
85%) : colorless oil; Rf = 0.25 (hexane/EtOAc, 1:1); [α]20D +23.6° (c 0.01, CH2Cl2); IR (C6H6) 3587, 2928, 1712, 1625 cm-1; 1H NMR (600 MHz, C6D6) δ 8.01 (dd, J = 15.6, 12.0 Hz, 1 H), 7.06 (d, J = 8.4 Hz, 1 H), 6.76 (d, J = 12.0 Hz, 1 H), 6.61 (d, J = 8.4 Hz, 1 H), 6.14 (d, J = 12.0 Hz, 1 H), 6.09 (d, J = 15.6 Hz, 1 H), 5.02-4.90 (m, 1 H), 4.00 (q, J = 7.2 Hz, 2 H), 3.55-3.50 (m, 1 H), 3.31-3.28 (m, 1 H), 3.21 (d, J = 6.6 Hz, 2 H), 3.14-3.08 (m, 1 H), 2.23 (s, 3 H), 1.91 (dd, J = 8.8, 5.6 Hz, 1 H), 1.85 (dd, J = 8.8, 3.4 Hz, 1 H), 1.57 (s, 3 H), 1.02 (s, 9 H), 0.94 (t, J = 7.2 Hz, 3 H), 0.10 (s, 6 H); HRMS (ESI) calcd for C27H42O5SiNa (M+Na)+ 497.2699, found 497.2704. To a solution of the diol (50.0 mg, 0.109 mmol) in DMF (3.0 mL) were added imidazole (35.0 mg, 0.491 mmol) and TBSCl (48.0 mg, 0.490 mmol) at 0 °C. After stirring at 0 °C for 4 h, the mixture was diluted with EtOAc. The organic layer was washed with H2O and brine. Dried over MgSO4, concentration gave the crude mono-TBS ether (55.0 mg). This compound was used for next reaction without further purification. To a solution of the above TBS ether (55.0 mg) in CH2Cl2 (2.0 mL) was added DIBALH (0.36 mL, 0.354 mmol, 0.97 M in hexane) at –78 °C. The mixture was stirred at the same temperature for 3 h before the reaction was quenched with satd aq. sodium potassium tartrate. The aqueous layer was separated and washed with EtOAc. The combined organic layer was washed with H2O and brine. Dried over MgSO4, concentration and column chromatography (hexane/EtOAc. 5:1) gave the diol 25 (40.0 mg, 70% in 2 steps): colorless oil; Rf = 0.31 (hexane/EtOAc, 2:1); [α]20D +9.2° (c 0.05, CH2Cl2); IR (C6H6) 3215, 2976, 1716 cm-1; 1H NMR (600 MHz, C6D6) δ 7.79 (dd, J = 15.6, 11.6 Hz, 1 H), 7.00 (d, J = 8.4 Hz, 1 H), 6.81 (d, J = 11.6 Hz, 1 H), 6.60 (d, J = 8.4 Hz, 1 H), 6.06 (t, J = 11.6 Hz, 1 H), 5.94 (d, J = 15.6 Hz, 1 H), 5.10 (t, J = 6.6 Hz, 1 H), 3.77-3.73 (m, 1 H), 3.45 (dd, J = 9.8, 3.6 Hz, 1 H), 3.36 (dd, J = 9.8, 6.6 Hz, 1 H), 3.27 (m, 2 H), 3.24 (m, 2 H), 2.24 (s, 3 H), 2.11 (m, 2 H), 1.69 (s, 3 H), 1.03 (s, 9 H), 0.90 (s, 9 H), 0.10 (s, 6 H), 0.01 (s, 3 H), 0.00 (s, 3 H).
Carboxylic Acid 26. To a solution of 25 (40.0 mg, 0.075 mmol) in CH2Cl2 (3.0 mL) was added MnO2 (65.0 mg, 0.750 mmol) at rt. After stirring for 12 h, the reaction residue was filtered off with a Celite pad, and washed with EtOAc. Concentration gave the crude aldehyde (38.0 mg). This aldehyde was used for next reaction without further purification. To a solution of the above aldehyde (38.0 mg) in THF (1.5 mL), t-BuOH (1.5 mL), and H2O (0.5 mL) were added 2-methyl-2-butene (0.08 mL, 0.75 mmol), NaH2PO4·2H2O (35.0 mg, 0.220 mmol), and NaClO2 (20.0 mg, 0.220 mmol) at 0 °C. After stirring at rt for 7 h, the mixture was diluted with EtOAc. The organic layer was separated and washed with H2O and brine. Dried over MgSO4, concentration gave the crude carboxylic acid 26 (47.0 mg). This compound was used for next reaction without further purification.
Lactone 27. To a solution of 26 obtained above (47.0 mg) in CH2Cl2 (10 mL) were added DMAP (360
mg, 0.300 mmol) and MNBA (1.03 g, 0.300 mmol) at 0 °C. The mixture was stirred at rt for 15 h before the reaction was quenched with satd aq. NH4Cl. The mixture was diluted with EtOAc, then the organic layer was separated and washed with H2O and brine. Dried over MgSO4, concentration and column chromatography (hexane/EtOAc, 9:1 to 4:1) gave the lactone 27 (23.0 mg, 56% in 3 steps): colorless solid; Rf = 0.52 (hexane/EtOAc, 7:1); [α]20D –52.6° (c 0.25, CH2Cl2); IR (C6H6) 2928, 1755 cm-1; 1H NMR (600 MHz, C6D6) δ 7.86 (dd, J = 15.0, 12.1 Hz, 1 H), 6.82 (d, J = 9.0 Hz, 1 H), 6.74 (d, J = 9.0 Hz, 1 H), 6.45 (d, J = 12.1 Hz, 1 H), 6.00 (t, J = 12.1 Hz, 1 H), 5.84 (d, J = 15.0 Hz, 1 H), 5.21 (m, 1 H), 5.14-5.10 (m, 1 H), 3.82 (dd, J = 10.5, 5.1 Hz, 1 H), 3.62 (dd, J = 10.5, 6.0 Hz, 1 H), 3.28-3.24 (m, 1 H), 3.21-3.16 (m, 1 H), 2.37 (m, 1 H), 2.27 (s, 3 H), 2.20-2.17 (m, 1 H), 1.39 (s, 3 H), 1.03 (s, 9 H), 0.94 (s, 9 H), 0.14 (s, 6 H), 0.05 (s, 3 H), 0.03 (s, 3 H); 13C NMR (150 MHz, C6D6) δ 167.7, 154.3, 143.0, 140.3, 138.0, 132.8, 130.7, 129.2, 128.9, 128.8, 127.4, 122.7, 116.9, 72.1, 65.9, 43.1, 30.5, 30.4, 30.2, 26.0, 25.9, 18.4, 15.6, -4.2, -5.2, -5.3; HRMS (ESI) calcd for C31H50O4Si2Na (M+Na)+ 565.3145, found 565.3148.
TADA Reaction of 27. A solution of 27 (5.2 mg, 9.59 µmol) in 1,2,4-trichlorobenzene (1.0 mL) was stirred at 160 °C for 10 h. Concentration and purification with PTLC (hexane/EtOAc, 8:1) gave 28 (2.7 mg, 50%) and 29 (1.9 mg, 35%). For 28: colorless solid; Rf = 0.23 (hexane/EtOAc, 7:1); [α]20D +69.7° (c 0.14, CH2Cl2); IR (CHCl3) 2930, 1717, 1601 cm-1; 1H NMR (400 MHz, C6D6) δ 6.81 (d, J = 7.2 Hz, 1 H), 6.73 (d, J = 7.2 Hz, 1 H), 5.69 (dt, J = 9.6, 2.4 Hz, 1 H), 5.58 (dt, J = 9.6, 2.4 Hz, 1 H), 4.18-4.14 (m, 1 H), 3.62 (dd, J = 11.4, 4.2 Hz, 1 H), 3.46 (dd, J = 11.4, 3.6 Hz, 1 H), 3.38-3.35 (m, 1 H), 2.99-2.97 (m, 1 H), 2.42 (dd, J = 15.6, 7.8 Hz, 1 H), 2.27 (dd, J = 15.6, 10.8 Hz, 1 H), 2.16 (s, 3 H), 2.11-1.97 (m, 2 H), 1.07 (s, 9 H), 0.99 (s, 9 H), 0.96-0.92 (m, 1 H), 0.70 (s, 3 H), 0.17 (s, 3 H), 0.16 (s, 3 H), 0.13 (s, 6 H); 13C NMR (150 MHz, C6D6) δ 171.0, 153.1, 142.6, 137.2, 130.8, 128.4, 123.5, 121.4, 117.6, 76.8, 65.8, 48.8, 46.1, 43.4, 32.6, 32.5, 31.5, 30.2, 26.0, 25.9, 23.7, 18.5, 13.4, -4.2, -5.1, -5.3; HRMS (ESI) calcd for C31H50O4Si2Na (M+Na)+ 565.3145, found 565.3130. For 29: colorless solid; Rf = 0.41 (hexane/EtOAc, 7:1); [α]20D –43.7° (c 0.10, CH2Cl2); IR (CHCl3) 2928, 1729, 1602 cm-1; 1H NMR (400 MHz, CDCl3) δ 6.92 (d, J = 7.8 Hz, 1 H), 6.62 (d, J = 7.8 Hz, 1 H), 5.89 (dt, J = 10.2, 3.0 Hz, 1 H), 5.59 (dt, J = 10.2, 2.4 Hz, 1 H), 4.64-4.60 (m, 1 H), 3.75-3.71 (m, 2 H), 3.69 (dd, J = 10.8, 4.8 Hz, 1 H), 3.13 (dd, J = 5.4, 3.0 Hz, 1 H), 2.82 (dd, J = 15.0, 7.8 Hz, 1 H), 2.67-2.50 (m, 1 H), 2.09 (s, 3 H), 1.95 (dd, J = 14.4, 4.2 Hz, 1 H), 1.71-1.65 (m, 1 H), 1.15 (s, 3 H), 1.02-0.95 (m, 1 H), 1.01 (s, 9 H), 0.91 (s, 9 H), 0.20 (s, 3 H), 0.19 (s, 3 H), 0.09 (s, 6 H); 13C NMR (150 MHz, CDCl3) δ 173.1, 158.9, 152.9, 142.3, 136.4, 132.9, 127.8, 120.8, 117.8, 77.1, 65.0, 48.5, 47.0, 43.5, 37.4, 37.0, 33.0, 32.0, 29.7, 29.5, 25.8, 25.7, 13.1, 0.0, -4.1, -5.3; HRMS (ESI) calcd for C31H50O4Si2Na (M+Na)+ 565.3145, found 565.3132.
ACKNOWLEDGMENTS
This work was supported in part by a Grant-in-Aid for Creative Scientific Research (16GS0206) from the Ministry of Education, Culture, Sports, Science and Technology, Japan.
Dedicated to Professor Emeritus Keiichiro Fukumoto on the occasion of his 75th birthday.
References
. †Present address. Faculty of Science and Technology, Keio University, 3-14-1 Hiyoshi, Kohoku-ku, Yokohama 223-8522.
1. G. M. Cragg and D. J. Newman, Cancer Invest., 1999, 17, 153.
2. B. H. Long, J. M. Carboni, A. J. Wasserman, L. A. Cornell, A. M. Casazza, P. R. Jensen, T. Lindel, W. Fenical, and C. R. Fairchild, Cancer Res., 1998, 58, 1111.
3. T. P. Hughes, Science, 1994, 265, 1547. CrossRef
4. T. Teruya, S. Nakagawa, T. Koyama, K. Suenaga, M. Kita, and D. Uemura, Tetrahedron Lett., 2003, 44, 5171; CrossRef T. Teruya, S. Nakagawa, T. Koyama, H. Arimoto, M. Kita, and D. Uemura, Tetrahedron, 2004, 60, 6989. CrossRef
5. For a preliminary communication, see: T. Ito, M. Ito, H. Arimoto, H. Takamura, and D. Uemura, Tetrahedron Lett., 2007, 48, 5465. CrossRef
6. For recent reviews of the TADA reaction, see: K. Takao, R. Munakata, and K. Tadano, Chem. Rev., 2005, 105, 4779; CrossRef E. Marsault, A. Toró, P. Nowak, and P. Deslongchamps, Tetrahedron, 2001, 57, 4243. CrossRef
7. M. Yamaguchi and I. Hirao, Tetrahedron Lett., 1983, 24, 391. CrossRef
8. E.-i. Negishi, D. E. Van Horn, and T. Yoshida, J. Am. Chem. Soc., 1985, 107, 6639. CrossRef
9. T. M. Cresp, R. G. F. Giles, M. V. Sargent, C. Brown, and D. O. Smith, J. Chem. Soc., Perkin Trans. 1, 1974, 2435. CrossRef
10. A. Wagner, M.-P. Heitz, and C. Mioskowski, Tetrahedron Lett., 1989, 30, 557. CrossRef
11. B. S. Bal, W. E. Childers, Jr., and H. W. Pinnic, Tetrahedron, 1981, 37, 2091. CrossRef
12. I. Shiina, M. Kubota, H. Oshiumi, and M. Hashizume, J. Org. Chem., 2004, 69, 1822. CrossRef
13. P. A. Grieco, J. J. Nunes, and M. D. Gaul, J. Am. Chem. Soc., 1990, 112, 4595. CrossRef
14. Treatment of the benzaldehyde, which was prepared from 20 by the formylation, with (PhO)2P(O)CH2CO2Et/NaH gave the corresponding unsaturated ester possessing the (Z)-alkene moiety at C7-C8. The ester group was reduced to the corresponding aldehyde followed by Wittig reaction with Ph3P=CHCO2Et to give the unsaturated ester, which possesses the Z,E-diene moiety. Further transformation to the TADA precursor was performed according to the synthetic route in Scheme 4.
15. The epimerization at C8 was thought to be proceeded via the radical pathway. Thus, we carried out the TADA reaction of 27 in the presence of Bu3SnD to catch the C8-deuterated TADA products. However, we couldn’t obtain such expected products. So far, reaction mechanism of the epimerization is unclear..
16. .
17.