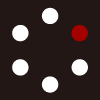
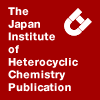
HETEROCYCLES
An International Journal for Reviews and Communications in Heterocyclic ChemistryWeb Edition ISSN: 1881-0942
Published online by The Japan Institute of Heterocyclic Chemistry
e-Journal
Full Text HTML
Received, 30th July, 2008, Accepted, 11th September, 2008, Published online, 11th September, 2008.
DOI: 10.3987/COM-08-S(D)7
■ Design and Synthesis of a Conformationally Restricted Analogue of the EFGH-ring System of Ciguatoxin
Nayoung Lee, Masayuki Inoue,* and Masahiro Hirama*
1Department of Chemistry, Graduate School of Science, Tohoku University, Sendai 980-8578, Japan
Abstract
The fused-hexahydrooxonine (F-ring) of ciguatoxin (CTX), as well as its EFGH-ring fragment, undergoes a slow conformational change between two conformers in solution. In this paper, we designed and synthesized a conformationally restricted analogue of the EFGH-ring system of ciguatoxin. Chemo- and stereoselective epoxidation of the F-ring successfully impeded the dynamic behavior without affecting the overall three-dimensional shape of the molecule.Ciguatoxin (CTX, 1, Figure 1) is one of the principal causative agents of ciguatera seafood poisoning in the Pacific.i,ii Ingestion of ciguateric seafood causes human gastrointestinal, neurological, and cardiovascular disturbances.iii Pharmacological studies indicated that 1 exerts its potent toxicity by binding and activating voltage-sensitive sodium channels (VSSC) in excitable membranes.iv Brevetoxin-A (2),v which is a structurally related red-tide toxin, shares the specific binding site with 1 on VSSC.vi Both toxins possess a conformationally flexible hexahydrooxonine in the center of the molecule (F-ring of 1 and E-ring of 2). As shown in Figure 2, the F-ring of 1 is known to undergo a slow conformational change between the UP and DOWN conformers, which differ only in orientation of the olefinic bond with regards to the ring plane (Figure 2).1,vii,viii It has been speculated that the flexibility of the toxins plays an important role in their biological activity.8
To evaluate the precise role of this unique equilibrium, we planned to synthesize a conformationally restricted analogue of 1 with minimum perturbation of the parent structure. In this context, we designed new epoxide analogue 3 (Figure 2). The addition of merely one oxygen atom to 1 was proposed to impede the dynamic motion of the nine-membered ring of 1. The α-epoxide of 3 would force the UP conformer to be predominant without affecting the overall three-dimensional shape, because the corresponding DOWN conformer would be significantly destabilized through the electronic repulsion of the two oxygen lone pairs. Here, we report model studies to prove these concepts. The mono-epoxidated analog of the EFGH-ring system of ciguatoxin (CTX) was synthesized, and its restricted conformation was assessed by dynamic 1H-NMR.
Synthesis of the middle portion of 1 started with the previously reported EGH-ring fragment 4 (Scheme 1).2 DIBAL reduction of methyl ester 4 to the aldehyde, followed by Wittig methylenation produced triene 5. Treatment of 5 with catalytic first-generation Grubbs reagent resulted in closure of the nine-membered F-ring to form 6. Lastly, reductive removal of the five benzyl groups, and subsequent protection of the two terminal 1,3-diols using PhCH(OEt)2 under acidic conditions, gave rise to the EFGH-ring system 7.x
Interestingly, the UP conformer was observed by X-ray crystallographic analysis of 7 as the sole isomer (Figure 3). On the other hand, 1H-NMR signals of 7 were significantly broadened at 30 °C, indicating slow conformational change between the UP and DOWN conformers (Figure 4). When the temperature was lowered to -30 °C, these signals sharpened to reveal that 7 predominantly exists as one conformation, which is consistent with the conformational characteristics of ciguatoxin 1. Thus, 7 was shown to reproduce the dynamic behavior of 1.
Chemo- and stereoselective epoxidation of diene 7 was realized using dimethyldioxirane (DMDO)xii (Scheme 2). DMDO selectively functionalized the α-face of 7 (α : β = 4 : 1), and pure α-epoxide 8 was isolated in 63% yield after chromatographic separation of the minor β-epoxide.xiii Both chemo- and stereoselectivities may be explained by the intrinsic three-dimensional structure (Figure 3). The α-face of the C26-27 olefin of the UP conformer is more spatially exposed, thus more reactive, than the corresponding β-face and the C21-22 olefin.
NOE experiments in CDCl3 clearly indicated that compound 8 had the UP conformation, similar to 7 (Scheme 2). Most importantly, in contrast to the broadened 1H-NMR signals of hexahydrooxonine 7, 1H-NMR spectra of epoxide 8 were constant over a broad temperature range (-30 °C ~ 60 °C), clearly indicating no slow conformational equilibrium (Figure 5). These two results proved that 8 acted as a conformationally frozen analogue of the UP conformer of 7. Significantly, this one-step chemical transformation successfully suppressed the dynamic behavior without affecting the overall three-dimensional structure of the parent compound.
In summary, we designed and synthesized a conformationally restricted analogue of the EFGH-ring system of ciguatoxin (CTX). Application of the developed methodology to ciguatoxins for elucidation of flexibility-activity relationships is currently underway in our laboratory.
ACKNOWLEDGEMENTS
This work was supported financially by SORST, Japan Science and Technology Agency (JST), and a Grant-in-Aid for Scientific Research (S) from the Japan Society for the Promotion of Science (JSPS).
References
1. (a) M. Murata, A.-M. Legrand, Y. Ishibashi, M. Fukui, and T. Yasumoto, J. Am. Chem. Soc., 1990, 112, 4380; CrossRef (b) M. Satake, A. Morohashi, H. Oguri, T. Oishi, M. Hirama, N. Harada, and T. Yasumoto, J. Am. Chem. Soc., 1997, 119, 11325. CrossRef
2. Total synthesis of CTX was recently achieved by our group. M. Inoue, K. Miyazaki, Y. Ishihara, A. Tatami, Y. Ohnuma, Y. Kawada, K. Komano, S. Yamashita, N. Lee, and M. Hirama, J. Am. Chem. Soc., 2006, 128, 9352. CrossRef
3. (a) P. J. Scheuer, Tetrahedron, 1994, 50, 3; CrossRef (b) R. J. Lewis, Toxicon, 2001, 39, 97; CrossRef (c) L. Lehane and R. J. Lewis, Int. J. Food. Microbiol., 2000, 61, 91. CrossRef
4. (a) J.-N. Bidard, H. P. M. Vijverberg, C. Frelin, E. Chungue, A.-M. Legrand, R. Bagnis, and M. Lazdunski, J. Biol. Chem., 1984, 259, 8353; (b) A. Lombet, J.-N. Bidard, and M. Lazdunski, FEBS Lett., 1987, 219, 355; CrossRef (c) M.-Y. Dechraoui, J. Naar, S. Pauillac, and A.-M. Legrand, Toxicon, 1999, 37, 125; CrossRef (c) K. Yamaoka, M. Inoue, H. Miyahara, K. Miyazaki, and M. Hirama, Br. J. Pharmacol., 2004, 142, 879. CrossRef
5. (a) Y. Shimizu, H.-N. Chou, H. Bando, G. Van Duyne, and J. C. Clardy, J. Am. Chem. Soc., 1986, 108, 514; CrossRef (b) J. Paulak, M. S. Tempesta, J. Golik, M. G. Zagorski, M. S. Lee, K. Nakanishi, T. Iwashita, M. L. Gross, and K. B. Tomer, J. Am. Chem. Soc., 1987, 109, 1144. CrossRef
6. M. A. Poli, T. J. Mende, and D. G. Baden, Mol. Pharmacol., 1986, 30, 129.
7. (a) M. Inoue, M. Sasaki, and K. Tachibana, Tetrahedron Lett., 1997, 38, 1611; CrossRef (b) M. Inoue, M. Sasaki, and K. Tachibana, J. Org. Chem., 1999, 64, 9416; CrossRef (c) M. Inoue, M. Sasaki, and K. Tachibana, Tetrahedron, 1999, 55, 10949; CrossRef (d) H. Imai, H. Uehara, M. Inoue, H. Oguri, T. Oishi, and M. Hirama, Tetrahedron Lett., 2001, 42, 6219; CrossRef (e) M. Sasaki, T. Noguchi, and K. Tachibana, J. Org. Chem., 2002, 67, 3301; CrossRef (f) S. Takai and M. Isobe, Org. Lett., 2002, 4, 1183; CrossRef (g) S. Takai, N. Sawada, and M. Isobe, J. Org. Chem., 2003, 68, 3225. CrossRef
8. T. Yasumoto and M. Murata, Chem. Rev., 1993, 93, 1897. CrossRef
9. P. Schwab, R. H. Grubbs, and J. W. Ziller, J. Am. Chem. Soc., 1996, 118, 100. CrossRef
10. Physicochemical data of 7: [α]D 27 -3.5 (c 0.34, CHCl3); IR (film) 2954, 2924, 2854, 2359, 1732, 1456, 1377, 1274, 1096, 698 cm-1; 1H-NMR (500 MHz, CDCl3) δ 7.49-7.36 (10H, m, Ph), 5.97 (1H, d, J =12 Hz, H22), 5.77 (2H, brd, H26, H27), 5.72 (1H, ddd, J = 12, 2.5, 2.5 Hz, H21), 5.52 (1H, s, Ph acetal), 5.45 (1H, s, Ph acetal), 4.30 (1H, d, J = 2.5 Hz, H20), 4.25(1H, dd, J = 10.5, 4 Hz, H18), 4.23 (1H, dd, J = 10.5, 5 Hz, H18), 3.85 (1H, d, J = 9 Hz, H24), 3.84 (1H, d, J = 8 Hz, H30), 3.61 (5H, m, H23, H29, H38x2, OH32), 3.51 (2H, m, H34, H36), 3.43 (2H, m, H19, H37), 3.26 (1H, dd, J = 11.5, 4 Hz, H32), 2.81 (1H, brd, H25), 2.77 (1H, brd, H28), 2.23 (3H, m, H31, H35x2), 2.10 (2H, brd, H25, H28), 1.87 (1H, dd, J = 23.5, 11.5 Hz, H31), 1.22 (3H, s, Me); 13C-NMR (125 MHz, CDCl3) δ 137.5, 137.3, 135.9, 131.0, 129.1, 129.0, 128.3, 128.3, 127.8, 126.2, 126.1, 101.8, 100.8, 86.2, 83.9, 83.5, 83.1, 81.4, 80.9, 79.9, 78.1, 74.6, 73.6, 69.8, 69.5, 66.0, 38.2, 32.3, 31.9, 31.7, 29.7, 9.8; HRMS (FAB), calcd. for C36H42O9Na 641.2721 (M+Na+), found 641.2721.
11. Ciguatoxins and closely related model compounds exhibit a preference for the UP conformation over the DOWN one. See refs 1 and 7.
12. (a) R. W. Murray, Chem. Rev., 1989, 89, 1187; CrossRef (b) W. Adam, R. Curci, and J. O. Edwards, Acc. Chem. Res., 1989, 22, 205. CrossRef
13. Physical data of 8: [α]D 29 1.2 (c 0.12, CHCl3); IR (film) λ 2923, 2360, 2341, 1458, 1380, 1094, 1020, 696 cm-1; 1H-NMR (500 MHz, CDCl3) δ 7.50-7.48 (5H, m, Ph), 7.39-7.37 (5H, m, Ph), 6.02 (1H, ddd, J = 12, 2, 2 Hz, H22), 5.79 (1H, ddd, J = 12, 2, 2 Hz, H21), 5.46 (1H, s, Ph acetal), 5.43 (1H, s, Ph acetal), 4.35 (1H, dd, J = 9.5, 2 Hz, H20), 4.26 (1H, dd, J = 10, 4 Hz, H18), 4.24 (1H, dd, J = 10, 5 Hz, H18), 4.01 (1H, dd, J =9.5, 2 Hz, H23), 3.87 (1H, dd, J = 8.5, 8.5 Hz, H30), 3.69 (1H, ddd, J = 8, 2.5, 2.5 Hz, H24), 3.61 (5H, m, H38x2, H34, H36), 3.53 (1H, m, H29), 3.45 (2H, m, H37, H19), 3.30 (1H, ddd, J = 9, 9, 3 Hz, H26), 3.28 (1H, dd, J = 9, 4 Hz, H27), 2.28 (1H, s, OH32), 2.37-2.24 (3H, m, H35x2, H31), 2.20 (1H, d, J = 14 Hz, H25), 2.15 (1H, d, J = 14 Hz, H28), 1.88 (1H, dd, J = 23.5, 11.5 Hz, H31), 1.68 (1H, dddd, J = 9, 9, 4.5, 4.5 Hz, H25), 1.67 (1H, dddd, J = 9, 9, 4.5, 4.5 Hz, H28), 1.3 (3H, s, Me); 13C-NMR (125 MHz, CDCl3) δ 137.5, 137.3, 135.9, 131.0, 129.1, 129.0, 128.3, 128.3, 127.8, 126.2, 126.1, 101.8, 100.8, 86.2, 83.9, 83.5, 83.1, 81.4, 80.9, 79.9, 78.1, 74.6, 73.6, 69.8, 69.5, 66.0, 38.2, 32.3, 31.9, 31.7, 29.7, 9.8; HRMS (FAB), calcd for C36H42O10Na 657.2670 (M+Na+), found 657.2670