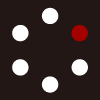
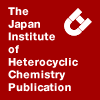
HETEROCYCLES
An International Journal for Reviews and Communications in Heterocyclic ChemistryWeb Edition ISSN: 1881-0942
Published online by The Japan Institute of Heterocyclic Chemistry
e-Journal
Full Text HTML
Received, 30th July, 2008, Accepted, 26th September, 2008, Published online, 29th September, 2008.
DOI: 10.3987/COM-08-S(F)81
■ Ether Formation in the Hydrogenolysis of Hydroxymethylfurfural over Palladium Catalysts in Alcoholic Solution
Gerard C. A. Luijkx, Nina P. M. Huck, Gred van Rantwijk, Leendert Maat,* and Herman van Bekkum*
Faculty of Chemical Engineering, Delft University of Technology, Julianalaan 136, 2628 BL Delft, The Netherlands
Abstract
5-Hydroxymethylfurfural, a product from renewable feedstock, was subjected to hydrogenolysis over palladium catalysts in 1-propanol aiming at the synthesis of 2,5-dimethylfuran, a potential transportation fuel enhancer. Intermediates are 5-hydroxymethyl-2-(propyloxymethyl)furan, formed with high selectivity, and 5-methyl-2-(propyloxymethyl)furan. Acetals are assumed to be initially formed. Acetalisation and hydrogenolysis are catalysed by traces of a Brønsted acid. Important variables are the palladium support and the solvent. In 2-propanol also ether formation takes place. In 1,4-dioxane mainly 2,5-bis(hydroxymethyl)furan is formed, in water ring opening becomes a major reaction. The formation of some side-products is discussed.INTRODUCTION
2,5-Dimethylfuran receives a growing interest as a liquid transportation fuel component. It is an excellent octane enhancer with an exceptionally high blending research octane number of 215,1 whereas, for example, ethanol has a number of 142 and methyl tert-butyl ether has an octane number of 118.2 2,5-Dimethylfuran is a stable compound, not miscible with water, it possesses a favourable energy density and a higher boiling point compared to ethanol, nowadays a well known fuel additive.
The rationale behind our investigations was to develop a novel synthesis of 2,5-dimethylfuran from 5-hydroxymethylfurfural (HMF). This renewable compound is the key chemical in our synthesis and can be obtained in good yields by acid catalysed dehydration of fructose. In the framework of a growing interest in renewable feedstock for the chemical industry, fructose is an attractive starting material because of its ample availability, easy purification and relatively low price. It is produced by isomerisation of glucose, obtainable from starch or cellulose, by hydrolysis of sucrose, both followed by glucose/fructose separation, or by hydrolysis of inulin (Scheme 1). One-pot hydrolysis/dehydration of inulin3 might constitute a direct route to 5-hydroxymethylfurfural. Its preparation from carbohydrates and the chemistry of 2,5-disubstituted furans have been reviewed by Moreau et al.4 These authors and a recent overview5 also point out the potentials of HMF derivatives such as furan-2,5-dicarboxylic acid6 and 2,5-bis(hydroxymethyl)tetrahydrofuran as monomers for polyester syntheses.
Descotes et al. have studied carefully7 the hydrogenation of 5-hydroxymethylfurfural aiming at the selective preparation of 2,5-bis(hydroxymethyl)furan or of 2,5-bis(hydroxymethyl)tetrahydrofuran. Raney nickel and copper chromites turned out to be suitable catalysts. A careful control of reaction conditions allowed reaction to stop at the 2,5-bis(hydroxymethyl)furan stage. In the analogous hydrogenation of furfural to furfuryl alcohol copper chromites are industrially used as catalysts. Here also copper-on-carbon8 and modified Raney nickel9 catalysts gave good results.
As to the hydrogenation/hydrogenolysis of 5-hydroxymethylfurfural into 2,5-dimethylfuran we have investigated two approaches: the direct multistep conversion of HMF and a route via 5-chloromethylfurfural. In the latter synthesis10 this compound was hydrogenated over Pd-on-C to give first 5-methylfurfural and subsequently 2,5-dimethylfuran. For the direct hydrogenation/hydrogenolysis of HMF Dumesic et al. recently designed11 RuCu-on-C catalysts within a detailed liquid phase process involving conversion of fructose into 2,5-dimethylfuran. In this process fructose-to-HMF selectivities up to 84% and HMF-to-2,5-dimethylfuran selectivities up to 71% were obtained.
The present paper reports results on the Pd-catalyzed hydrogenation/hydrogenolysis of 5-hydroxymethylfurfural. Palladium is a well known catalyst for the hydrogenolysis of benzyloxy compounds which are often applied as hydroxyl group protecting intermediates. The HMF hydrogenation experiments were carried out in the liquid phase using alcoholic solvents. To our surprise the monoethers of 2,5-bis(hydroxymethyl)furan were formed with high selectivity. This was not mentioned in earlier publications on the production of furan derivatives.4,12,13
RESULTS AND DISCUSSION
Hydrogenolysis of 5-chloromethylfurfural (6) – 5-Chloromethylfurfural was subjected to Pd-catalysed hydrogenolysis using 1-propanol as the solvent at 60 °C. After 90 min reaction time 5-chloromethylfurfural conversion was 98% and the selectivity to 2,5-dimethylfuran was 67.3%. Moreover, the first hydrogenolysis product, compound 7, (Scheme 2) was present (9.2%) and also the hydrolysis product of 5, the dione 8 (14.3%). Compound 4 was not observed in the reaction product indicating that the hydrogenation of 7 is the slow step in the 5-chloromethylfurfural hydrogenolysis. It may be noted that the hydrochloride acid, formed in the first step will accelerate the hydrogenolysis of 4.
These results are in good agreement with data in a Sumitomo patent,10 in two experiments in ethanol at 20 °C selectivities to 5 of 64.7 and 70.0% are reported.
Hydrogenolysis of 5-hydroxymethylfurfural in organic solvents – The hydrogenation sequence of HMF in 1-propanol using 10% Pd/C catalyst led to unexpected intermediate compounds. Using GC-MS, we found that the monopropyl ether of 2,5-bis(hydroxymethyl)furan (10) was the main product (>80% yield) in the initial stage of the reaction (Figure 1a).
The formation of ethers from ketones under hydrogenation conditions has been reported before by Verzele et al.,14 who applied PtO2 as a catalyst precursor and lower alcohols, 2.5 M in HCl, as reactant and solvent. A possible mechanism for this phenomenon was presented by Kieboom and Van Rantwijk15 who proposed the formation of an intermediate hemiacetal, which is subsequently reduced to the corresponding ether (Scheme 3). This reaction mechanism would require the presence of acid. In our case, it is assumed that the palladium on activated carbon used, contains some Brønsted acid at the carbon surface, which presumably originates from the reduction of PdCl2 into Pd(0) and HCl during preparation of the catalyst. The fact that no dipropyl ether of 2,5-bis(hydroxymethyl)furan was found, indicates that these ethers are indeed only formed at the aldehyde group and not produced by reaction of 1-propanol with the hydroxymethyl groups of 2 under these conditions (although an acid-catalysed mechanism can easily be imagined). It was found that the ether (10) is first hydrogenolysed at the alcohol function, followed by hydrogenolysis of the ether bond to produce 2,5-dimethylfuran. Because only very small amounts of 2,5-bis(hydroxymethyl)furan were found, we conclude that the propyl ethers are the major intermediates in the hydrogenolysis of HMF (Scheme 3). When 2-propanol was used instead of 1-propanol, a similar reaction sequence was found, with intermediate isopropyl ethers. Hydrogenolysis of the isopropyl ether function, however, is slower than that of the propyl ethers. Consequently, compound 11 (R = iPr) becomes the major product here.
When 5% palladium on alumina catalyst was applied, no formation of the ethers was observed (Figure 1b); at the same time the yield of 2,5-dimethylfuran decreased drastically. The main product was the diol 2. The hydrogenation of HMF to 2 ceased almost completely after 100 min. Oligomeric products formed on the alumina might be the reason for this. By adding a small amount of concentrated HCl, the formation of the propyl ethers 10 and 11 could be induced again (Figure 1c, Table I, Entry 4). This shows that the presence of a trace of acid is absolutely necessary for the hydrogenolysis of HMF to 5.
Selectivities to 5 did not exceed 40% and passed through an optimum due to the formation of over-hydrogenated products. Thus, prolonged reaction times caused ring hydrogenation of 5 to 12 and hydrolytic ring opening of 5, resulting in the formation of 8. Small amounts of the hydrogenation product of 8, 2,5-hexanediol, were also detected. Furthermore, (partial) ring hydrogenation of the ethers 10 and 11 resulted in the formation of a large number of unquantified products (Table I). From Figure 1 it can be concluded that Pd/C is the most active catalyst but also increased the consecutive conversion of 5 towards non-wanted compounds.
Hydrogenolysis proceeded slowly in 1,4-dioxane and, as might be expected,16 2 became the main product in this medium.
Hydrogenolysis of 5-hydroxymethylfurfural in water – Since HMF is produced by acid catalysed dehydration of fructose in aqueous mixtures, the direct hydrogenolysis in water over Pd/C under acidic conditions would be interesting. Hydrogenolysis of HMF in water at pH 2.5 and 60 °C (Table I, Entry 6) resulted in the formation of 2 and of 1-hydroxy-2,5-hexanedione (13), the latter formation was mentioned earlier7 and ascribed to the hydrogenation of one of the intermediates in the conversion of HMF into levulinic acid. Alternatively, 13 could be formed via hydrogenation and hydrolysis of HMF.
Hydrogenation of the furan ring of 2 to the tetrahydrofuran compound and the hydrogenation of 13 and 14 were not observed under our mild reaction conditions.
The yield of 5 by reduction of HMF in water under the present conditions did not exceed 1%. In an attempt to remove 5 from the water layer during the reaction, toluene was added (Table I, Entry 7). In this way the yield of 5 could be raised slightly to 2.6%, but the main product was 13, resulting from acid catalysed ring opening of HMF followed by hydrogenation.
CONCLUSIONS
5-Hydroxymethylfurfural can be converted with hydrogen over Pd catalysts in 1-propanol into 2,5-dimethylfuran, with selectivity of up to 36%. Intermediate products are 5-hydroxymethyl-2-(propyloxymethyl)furan (selectivity > 80%) and 5-methyl-2-(propyloxymethyl)furan. Acetals are assumed to be initially formed. Acetalysation and hydrogenolysis are catalysed by traces of Brønsted acid.
Hydrogenation of 5-hydroxymethylfurfural in water under slightly acidic conditions produces only small amounts of 2,5-dimethylfuran, the major products are 2,5-bis(hydoxymethyl)furan and the ring opening product 1-hydroxy-2,5-hexanedione.
EXPERIMENTAL
Chemicals – HMF was kindly provided by Südzucker AG and was dried prior to use. 5-Chloromethylfurfural was prepared from HMF.17 The 10% palladium on carbon was a sample from H. Drijfhout NV, Amsterdam. 5% Palladium on alumina powder (Degussa type E211 R/D catalyst) was obtained from Aldrich.
Hydrogenolysis – Hydrogenolysis experiments were performed in a thermostatted glass batch reactor of 50 mL. A mixture of HMF (0.50 g), catalyst (0.25 g) and solvent (20 mL) was magnetically stirred at 60 °C under 1 atm of hydrogen. Consumption of hydrogen was monitored and samples of the reaction mixture were drawn via a septum and analysed by GC and GC-MS.
In case of HMF hydrogenation in water, the aqueous samples were first extracted with chloroform. The chloroform layer was analysed by GC and GC-MS and the water layer was analysed by HPLC and HPLC-MS.
This paper is dedicated to Professor Emeritus Keiichiro Fukumoto on the occasion of his 75th birthday.
References
1. M. J. Papachristos, J. Swithenbank, G. H. Priestman, S. Stournas, P. Polysis, and E. Lois, J. Inst. Energy, 1991, 64, 113.
2. F. H. Palmer, Proc. ImechE, 1986, 11, 33.
3. A. W. Heinen, J. A. Peters, and H. van Bekkum, Carbohydr. Res., 2001, 330, 381. CrossRef
4. C. Moreau, M. N. Belgacem, and A. Gandini, Top. Catal., 2004, 27, 11. CrossRef
5. J. N. Chheda, G. W. Huber, and J. A. Dumesic, Angew. Chem. Int. Ed., 2007, 46, 7164.. CrossRef
6. P. Vinke, H. E. van Dam, and H. van Bekkum, in New Developments in Selective Oxidation, G. Centi and F. Trifiró (Eds.), Elsevier Science Publishers, Amsterdam (1990) p. 147. CrossRef
7. V. Schiavo, G. Descotes, and J. Mentech, Bull. Soc. Chim. Fr., 1991, 128, 704.
8. R. Rao, R. T. K. Baker, and M. A. Venice, Catal. Lett., 1999, 60, 51. CrossRef
9. B. J. Liu, L. H. Lu, B. C. Wang, T. X. Cai, and K. Iwatani, Appl. Catal. A, 1998, 171, 117. CrossRef
10. K. Hamada, H. Yoshihara, and G. Suzukamo, Jpn Pat. JP 58 13,576 (1983) to Sumitomo Chemical Co., Ltd.
11. Y. Roman-Leshkov, C. J. Barrett, Z. Y. Liu, and J. A. Dumesic, Nature, 2007, 447, 982. CrossRef
12. Z. Guo, G. Wang, and F. Zhao, Huaxue Shiji, 1995, 16, 182.
13. K.Hamada, H. Yoshihara, and G. Suzukamo, J. Oleo Sci., 2001, 50, 533..
14. M. Verzele, M. Acke, and M. Anteunis, J. Chem. Soc., 1963, 5598. CrossRef
15. A. P. G. Kieboom and F. van Rantwijk, Hydrogenation and Hydrogenolysis in Synthetic Organic Chemistry, Delft University Press, Delft (1977) p. 114.
16. P. N. Rylander, Catalytic Hydrogenation over Platinum Metals, Academic Press, New York (1967) p. 449.
17. T. Reichstein and H. Zschokke, Helv. Chim. Acta, 1932, 15, 249 CrossRef