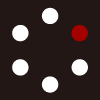
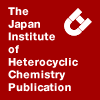
HETEROCYCLES
An International Journal for Reviews and Communications in Heterocyclic ChemistryWeb Edition ISSN: 1881-0942
Published online by The Japan Institute of Heterocyclic Chemistry
e-Journal
Full Text HTML
Received, 31st July, 2008, Accepted, 15th October, 2008, Published online, 16th October, 2008.
DOI: 10.3987/COM-08-S(F)83
■ Efficient Synthesis of [2]Rotaxanes Based on Sequential Acetylene-Dicobalt Hexacarbonyl Complexation and Stopper Modification
Yuji Tokunaga,* Norihiro Ohiwa, Go Ohta, Yuji Yamauchi, Tatsuhiro Goda, Nobuhiko Kawai, Takumichi Sugihara, Youji Shimomura, and Tomonori Hoshi
Department of Materials Science and Engineering, Faculty of Engineering, Fukui University, Bunkyo, Fukui 910-8507, Japan
Abstract
This paper describes an efficient end-capping method for the preparation of [2]rotaxanes, using acetylene–dicobalt hexacarbonyl complexation and subsequent transformation of the complexes into a series of vinylsilanes though hydrosilylation.INTRODUCTION
Rotaxanes have potential applicability as components within molecular machine and devices.1 Because the types of functional groups present in rotaxanes can affect their physical properties, one effective means of functionalization of rotaxanes is their construction with a transformable group as a stopper that is subsequently modified.2–5 Smithrud et al. used dicyclohexylcarbodiimide (DCC) to lock the ring-shaped component onto a capped-tether; the DCC-[2]rotaxane was then combined with amines to give corresponding rotaxanes in high yields.6 This methodology has been applied to the synthesis of rotaxanes possessing intracellular transporting abilities.7
Acetylene–dicobalt hexacarbonyl complexes8 are useful as intermediates in organic synthesis because they are substrates for Pauson–Khand,9 Nicholas,10 and hydrosilylation reactions.11 Furthermore, these complexes are practical protecting groups for acetylenes.12
Recently, modification of acetylene units was useful for syntheses of rotaxanes, including 1,3-dipolar cycloadditon,13 transition metal-catalyzed hydrosilylation,14 and hydroruthenation.15 We also demonstrated the efficient synthesis of rotaxanes based on acetylene–Co2(CO)6 complexation and the self-assembly of secondary ammonium ions and crown ethers.16 In this present paper, we describe in detail an efficient method for the preparation of rotaxanes using acetylene–Co2(CO)6 complexation and subsequent stopper-modification though hydrosilylation and decomplexation of acetylene–Co2(CO)6 complex (Scheme 1).
RESULTS AND DISCUSSION
Scheme 2 outlines the syntheses of the ammonium salts 3 that we used as substrates for the primary step. The condensation of aldehydes17–19 1 and benzylamines 2 gave the corresponding imines. Reduction of these imines, followed by protonation and counterion exchange, produced the corresponding ammonium salts 3, each of which possessed a bulky group at one end and an acetylene unit at the other (Scheme 2). These ammonium salts featured terminal (3a–c), methyl-substituted (3d), and phenyl-substituted (3e and f) acetylene units.
Prior to synthesizing the [2]rotaxanes, we determined the association constants for the assembly of the corresponding [2]pseudorotaxanes are examined. 1H NMR spectra [500 MHz, CD3CN/CDCl3 (1:1), 25 °C] of equimolar mixtures of 3 and dibenzo24crown-8 (DB24C8) (10 mM) revealed the formation of [2]pseudorotaxanes as stand-alone signals. Integration of the signals of the complexes and the ammonium salts 3a (monosubstituted acetylene) and 3d (disubstituted acetylene) afforded apparent association constants (Kexp)20 of 860 ± 190 and 1700 ± 440 M–1, respectively. Having confirmed the formation of [2]pseudorotaxanes, we attempted the synthesis of the corresponding [2]rotaxanes through complexation of acetylene and dicobalt octacarbonyl. Treatment of the acetylenes 3 with dicobalt octacarbonyl in the presence of two equivalents of DB24C8 afforded the corresponding [2]rotaxanes 4 (Scheme 3). The monosubstituted and disubstituted acetylenes provided their corresponding [2]rotaxanes 4 in excellent (Table 1, entries 1–3) and moderate (Table 1, entries 4–6) NMR spectroscopic yields, respectively.21
Next, we investigated the transformations of the [2]rotaxanes 4 into the [2]rotaxanes 5 and 6 through hydrosilylation and decomplexation of the acetylene–Co2(CO)6 complexes (Scheme 4, Table 2). In each case, the hydrosilylation reaction—heating with a trialkylsilane and bis(trimethylsilyl)acetylene10a—was complete within 3 h to afford the corresponding vinylsilanes as a mixture of regioisomers in moderate yield,22 The syn addition reaction predominantly proceeded, anti adducts were not observed in the NMR spectra of crude prducts.
We used NMR spectroscopy to determine the regiochemistry of the products (Figure 1). The coupling constants of olefinic protons of the major isomer 5a (run1) and minor isomer 6d (run 4) were 19 and 2.8 Hz, respectively. Irradiation of the protons of the olefinic protons of 5b and 5e resulted in NOE enhancements to the aromatic protons, indicating that these compounds were the external silylation products (runs 2 and 5). We also observed the enhancement the signal for aromatic protons on di-substituted benzene part of 5c, when the methylene protons net to silicon atoms were irradiated.
The bulk of the silyl group had an effect on the regioselectivity: generally the yields of the internal silylation products were slightly higher when we performed the reactions with diphenylmethylsilane (cf. runs 1 and 4, and 2 and 5). Isobe’s group has reported the hydrosilylation of the phenyl acetylene–Co2(CO)6 complex with triethylsilane;22 their selectivity (74:26) was close to ours (72:28, run 1).
Although the hydrosilylation mechanism has not been clarified, Isobe et al. suggested that the first step involved the hydride approaching to the carbon atom of the complex.22 Therefore, we might expect the hydride to react predominantly at the benzylic carbon atoms of the [2]rotaxanes 4a and 4d to afford the external vinylsilanes 5a, 5b, 5d, and 5e (runs 1, 2, 4, and 5, respectively). Because the substrate 4d possesses a hindering methyl group at the external carbon atom, the hydrosilylations of 4d proceeded more selectively (runs 2 and 5). The reactions of 4e (runs 3 and 6) favored the hydride reacting at the internal carbon atom to form the [2]rotaxanes 5c and 5f; because both sites are reactive benzylic carbon atoms, bulky silyl groups avoided the internal carbon atom which is hindered by the presence of the bulky DB24C8 unit.
In summary, we have synthesized several [2]rotaxanes through sequential acetylene–Co2(CO)6 complexation and stopper-modification via hydrosilylation/decomplexation. Because alkenylsilanes are particularly important functional groups in organic synthesis,23 these [2]rotaxanes will be useful for constructing related interlocked molecules.
EXPERIMENTAL
General Methods: Infrared spectra were recorded using a Shimadzu FTIR-8600PC spectrometer 1H NMR spectra were recorded using JEOL AL-300, EX-400, and LA-500 spectrometers; TMS was the internal standard. Mass spectra were recorded using a JMS-700T instrument. All reactions were performed under a positive atmosphere of dry N2, unless otherwise indicated. All extracts were dried over MgSO4 and the solvent removed through rotary evaporation under reduced pressure. Silica gel column chromatography was performed on Kanto Chemical silica gel 60N. Thin-layer chromatography was performed using Merck Kieselgel 60PF254. Melting points are uncorrected.
N-(3,5-Dimethylbenzyl)-N-(4-ethynylbenzyl)ammonium Hexafluorophosphate (3a). Triethylamine (3.04 g, 30.0 mmol) was added at rt to a suspension of 3,5-dimethylbenzylammonium chloride (1.72 g, 10.0 mmol), 4-ethynylbenzaldehyde (1.30 g, 10.0 mmol), and magnesium sulfate (1.20 g, 10.0 mmol) in CHCl3 (20 mL). The reaction mixture was stirred for 14 h at rt and then it was filtered. The filtrate was concentrated and the residue dissolved in EtOH and THF (1:1, 60 mL). Sodium borohydride (0.765 g, 20.0 mmol) was added to the solution at 0 °C and then the mixture was heated at 40 °C for 19 h. After cooling to 0 °C, 10% HCl was added to the reaction mixture, which was then neutralized with sat. aq. NaHCO3. The organic solvents were evaporated under reduced pressure and then the aqueous phase was extracted with AcOEt. The organic extract was washed with sat. aq. NaCl, dried, and concentrated. The residue was chromatographed (AcOEt/toluene, 1:1) to give the amine, which was dissolved in THF (20mL) and treated with 10% HCl (8 mL). After evaporation of the solvent, the residue was washed with toluene to afford the chloride salt as a solid. This salt was dissolved in a mixture of acetone and water (1:1, 70 mL), ammonium hexafluorophosphate (4.19 g, 260 mmol) was added, and the mixture stirred for 1 h at rt. After evaporation of the acetone, the precipitate was collected and washed with water to give 3a (2.01 g, 51%) as a white solid; mp 186–187 °C. IR ν max (KBr) cm–1 3253, 2921, 2120, 1611, 1411, 558. 1H NMR (300 MHz, DMSO-d6) δ 9.25–8.97 (br, 2H), 7.60–7.46 (m, 4H), 7.15–7.03 (m, 3H), 4.27 (s, 1H), 4.19 (br s, 2H), 4.08 (br s, 2H), 2.28 (s, 6H). 13C NMR (75 MHz, DMSO-d6) δ 138.54, 133.20, 132.59, 132.20, 131.01, 128.25, 123.06, 83.61, 82.26, 21.42. MS (FAB) m/z: 250 [M – PF6]+. Anal. Calcd for C18H20F6NP: C, 54.69; H, 5.10; N, 3.54. Found: C, 54.46; H, 5.17; N, 3.60.
N-(4-tert-Butylbenzyl)-N-(4-ethynylbenzyl)ammonium Hexafluorophosphate (3b). Yield: 84%; mp 189–191 °C. IR ν max (KBr) cm–1 3452, 2963, 1416, 559. 1H NMR (300 MHz, DMSO-d6) δ 9.24–9.03 (br, 2H), 7.62–7.33 (m, 8H), 4.29 (s, 1H), 4.21 (br s, 2H), 4.14 (br s, 2H), 1.28 (s, 9H). 13C NMR (75 MHz, DMSO-d6) δ 151.70, 132.66, 131.93, 130.29, 129.75, 128.93, 125.51, 122.33, 82.95, 81.79, 49.92, 49.77, 34.43, 31.03. MS (FAB) m/z: 278 [M – PF6]+. Anal. Calcd for C20H24F6NP·0.5H2O: C, 55.56; H, 5.83; N, 3.24. Found: C, 55.58; H, 5.73; N, 3.20.
N-(9-Anthryl)-N-(4-ethynylbenzyl)ammonium Hexafluorophosphate (3c). Yield: 51%; mp 179–180.5 °C. IR ν max (KBr) cm–1 3433, 3285, 3054, 2924, 2853, 2340, 1408, 559. 1H NMR (300 MHz, DMSO-d6) δ 9.45–9.26 (br, 2H), 8.80 (s, 1H), 8.36–8.25 (m, 2H), 8.22–8.16 (m, 2H), 7.72–7.57 (m, 8H), 5.19 (br s, 2H), 4.58 (br s, 2H), 4.32 (s, 1H). 13C NMR (75 MHz, DMSO-d6) δ 133.07, 132.34, 131.24, 131.13, 130.93, 130.23, 129.51, 127.36, 125.90, 124.49, 123.29, 122.88, 83.41, 82.16, 51.07, 42.54. MS (FAB) m/z: 322 [M – PF6]+. Anal. Calcd for C24H20F6NP: C, 61.67; H, 4.31; N, 3.00. Found: C, 61.75; H, 4.56; N, 2.86.
N-(3,5-Dimethylbenzyl)-N-{4-(1-propynyl)benzyl}ammonium Hexafluorophosphate (3d). Yield: 46%; mp 193–194 °C. IR ν max (KBr) cm–1 3429, 3251, 2918, 2220, 1612, 1414, 559. 1H NMR (300 MHz, DMSO-d6) δ 9.19–8.80 (m, 2H), 7.50–7.38 (m, 4H), 7.12–7.03 (m, 3H), 4.15 (br s, 2H), 4.07 (br s, 2H), 2.29 (s, 6H), 2.05 (s, 3H). 13C NMR (75 MHz, DMSO-d6) δ 137.78, 131.56, 131.33, 130.26 .130.18, 127.50, 125.80, 123.99, 87.67, 79.13, 50.15, 49.82, 20.72, 3.80. MS (FAB) m/z: 264 [M – PF6]+. Anal. Calcd for C19H22F6NP: C, 55.75; H, 5.42; N, 3.42. Found: C, 55.55; H, 5.38; N, 3.41.
N-(3,5-Dimethylbenzyl)-N-{4-(phenylethynyl)benzyl}ammonium Hexafluorophosphate (3e). Yield: 50%; mp 179–180.5 °C. IR ν max (KBr) cm–1 3251, 2920, 2225, 1611, 1417, 559. 1H NMR (300 MHz, DMSO-d6) δ 9.12–8.82 (br, 2H), 7.30–7.13 (m, 9H), 7.10–7.03 (m, 3H), 4.10 (br s, 2H), 4.04 (br s, 2H), 2.28 (s, 6H). 13C NMR (75 MHz, DMSO-d6) δ 137.86, 132.31, 131.78, 131.56, 131.42, 130.41, 130.35, 129.02, 128.83, 127.59, 122.91, 122.00, 90.22, 88.76, 50.25, 49.87, 20.78. MS (FAB) m/z: 326 [M – PF6]+. Anal. Calcd for C24H24F6NP: C, 61.15; H, 5.13; N, 2.97. Found: C, 61.43; H, 5.06; N, 3.04.
N-9-Anthryl-N-{4-(phenylethynyl)benzyl}ammonium Hexafluorophosphate (3f). Yield: 52%; mp 179–180.5 °C. IR ν max (KBr) cm–1 3432, 3223, 3056, 2925, 2214, 1414, 559. 1H NMR (300 MHz, DMSO-d6) δ 9.59–9.43 (br, 2H), 8.80 (s, 1H), 8.38–8.30 (m, 2H), 8.23–8.15 (m, 2H), 7.76–7.55 (m, 10H), 7.48–7.42 (m, 3H), 5.20 (br s, 2H), 4.59 (br s, 2H). 13C NMR (75 MHz, DMSO-d6) δ 133.18, 132.38, 132.23, 131.67, 131.59, 131.38, 130.67, 129.93, 129.81, 129.60, 127.79, 126.30, 124.92, 123.87, 123.67, 122.87, 91.10, 89.65, 51.54, 43.01. MS (FAB) m/z: 398 [M – PF6]+. HRMS (FAB) Calcd for C30H24N [M – PF6]+: m/z 398.1909. Found: 398.1925.
[2]-[(Dibenzo-24-crown-8){μ-[N-(3,5-dimethylbenzyl)-N-(4-ethynylbenzyl)ammonium]hexacarbonydicobalt}]rotaxane Hexafluorophosphate (4a). To a suspension of ammonium hexafluorophosphate 3a (100mg, 0.253mmol) and DB24C8 (227mg, 0.506mmol) in CH2Cl2 (2mL) was added dicobalt octacarbonyl (104mg, 0.304mmol) in CH2Cl2 (2.5mL) at 0 °C and stirred for 3 d at the same temperature. To the reaction mixture was added CHCl3, which was filtered though Celite. The filtrate and the mixture was concentrated, the residue was washed with toluene/hexane (1:1), and chromatographed (AcOEt/toluene) to give the rotaxane. The crude rotaxane was dissolved in a mixture of acetone and water (2:1, 30 mL) ammonium hexafluorophosphate (206mg, 1.27mmol) was added, and the mixture stirred for 1 h at rt. After evaporation of the acetone, the precipitate was collected and washed with water to give rotaxane 4a (214mg, 75%) as a red solid; Yield: 75%; mp 85–86 °C. IR ν max (KBr) cm–1 3455, 2930, 2056, 1124, 1053, 557. 1H NMR (300 MHz, CDCl3) δ 7.70–7.46 (br, 2H), 7.46–7.30 (m, 4H), 6.98–6.72 (m, 11H), 6.42 (s, 1H), 4.70–4.57 (m, 2H), 4.49–4.39 (m, 2H), 4.22–4.00 (m, 8H), 3.85–3.66 (m, 8H), 3.54–3.38 (m, 8H), 2.15 (s, 6H). 13C NMR (75MHz, CDCl3) δ 199.17, 147.45, 138.79, 138.37, 131.64, 131.22, 130.71, 130.24, 130.16, 126.65, 121.76, 112.70, 88.57, 88.43, 70.56, 70.10, 68.25, 52.71, 52.20, 21.13. MS (FAB) m/z: 984 [M – PF6]+. Anal. Calcd for C48H52Co2NF6O14P: C, 51.03; H, 4.64; N, 1.24. Found: C, 50.75; H, 4.68; N, 1.36.
[2]-[(Dibenzo-24-crown-8){μ-[N-(4-tert-butylbenzyl)-N-(4-ethynylbenzyl)ammonium]hexacarbonydicobalt}]rotaxane Hexafluorophosphate (4b). Yield: 65%; mp 97–99 °C. IR ν max (KBr) cm–1 3450, 3070, 2054, 1121, 1056, 558. 1H NMR (300 MHz, CDCl3) δ 7.74–7.52 (br, 2H), 7.41–7.34 (m, 2H), 7.33–7.16 (m, 6H), 6.92–6.86 (m, 4H), 6.80–6.73 (m, 4H), 6.40 (s, 1H), 4.71–4.60 (m, 2H), 4.57–4.49 (m, 2H), 4.16–4.02 (m, 8H), 3.83–3.72 (m, 8H), 3.47 (br s, 8H), 1.25 (s, 9H). 13C NMR (75 MHz, CDCl3) 199.19, 152.46, 147.35, 138.62, 131.64, 130.16, 129.94, 128.83, 128.31, 125.57, 121.69, 112.54, 88.43, 72.96, 70.46, 70.06, 68.09, 52.32, 52.07, 34.57, 31.14. MS (FAB) m/z: 1012 [M – PF6]+. Anal. Calcd for C50H56Co2NF6O14P·H2O: C, 51.07; H, 4.97; N, 1.19. Found: C, 50.85; H, 5.18; N, 1.32.
[2]-[(Dibenzo-24-crown-8){μ-[N-9-anthryl-N-(4-ethynylbenzyl)ammonium]hexacarbonydicobalt}]rotaxane Hexafluorophosphate (4c). Yield: 53%; mp > 200 °C. IR ν max (KBr) cm–1 3440, 2930, 2094, 2056, 2025, 1595, 1503, 1208, 1124, 1104, 1057, 557. 1H NMR (300 MHz, CDCl3) δ 8.50–8.40 (m, 2H), 8.12 (s, 1H), 7.86–7.38 (m, 12H), 6.73–6.60 (m, 4H), 6.42 (s, 1H), 6.40–6.25 (m, 4H), 5.59–5.46 (m, 2H), 5.34–5.20 (m, 2H), 3.96–3.53 (m, 20H), 3.47–3.34 (m, 4H). 13C NMR (75 MHz, DMSO-d6) δ 199.04, 145.85, 136.51, 132.47, 129.93, 129.83, 129.69, 129.30, 128.70, 127.62, 126.57, 124.49, 122.90, 120.57, 120.35, 111.32, 73.65, 69.88, 69.39, 68.66, 68.24, 67.06, 51.53, 44.33. MS (FAB) m/z: 1056 [M – PF6]+. Anal. Calcd for C54H52Co2NF6O14P: C, 53.97; H, 4.36; N, 1.17. Found: C, 53.65; H, 4.34; N, 1.22.
[2]-[(Dibenzo-24-crown-8){μ-[N-(3,5-dimethylbenzyl)-N-{4-(1-propynyl)benzyl}ammonium]hexacarbonydicobalt}]rotaxane Hexafluorophosphate (4d). Yield: 55%; mp 97–98 °C. IR ν max (KBr) cm–1 3436, 2919, 2260, 2052, 2018, 1596, 1506, 1253, 1124, 1108, 1057, 558. 1H NMR (300 MHz, CDCl3) δ 7.71–7.46 (br, 2H), 7.46–7.26 (m, 4H), 6.91–6.70 (m, 11H), 4.65–4.53 (m, 2H), 4.50–4.37 (m, 2H), 4.18–4.03 (m, 8H), 3.83–3.70 (m, 8H), 3.50–3.35 (m, 8H), 2.86 (s, 3H), 2.13 (s, 6H). 13C NMR (75 MHz, DMSO-d6) δ 199.12, 148.06, 146.66, 137.03, 131.05, 129.86, 128.38, 127.68, 126.10, 120.70, 113.76, 111.97, 78.67, 69.86, 69.60, 69.23, 68.68, 68.27, 67.30, 20.37, 20.20. MS (FAB) m/z: C49H54Co2NO14 998 [M – PF6]+. Anal. Calcd for C43H54Co2F6NO14P·0.5H2O: C, 50.66; H, 4.86; N, 1.21. Found: C, 50.93; H, 5.12; N, 1.25.
[2]-[(Dibenzo-24-crown-8){μ-[N-(3,5-dimethylbenzyl)-N-{4-(phenylethynyl)benzyl}ammonium]hexacarbonydicobalt}]rotaxane Hexafluorophosphate (4e). Yield: 73%; mp 100–101 °C. IR ν max (KBr) cm–1 3451, 2923, 2091, 2054, 2024, 1505, 1253, 1124, 1107, 1056, 558. 1H NMR (300 MHz, CDCl3) δ 7.75–7.18 (m, 11H), 7.00–6.76 (m, 11H), 4.74–4.65 (m, 2H), 4.50–4.40 (m, 2H), 4.19–4.05 (m, 8H), 3.82–3.72 (m, 8H), 3.53–3.42 (m, 8H), 2.15 (s, 6H). 13C NMR (75 MHz, CDCl3) δ 199.00, 166.02, 147.45, 139.67, 138.44, 137.80, 131.57, 131.22, 130.78, 130.26, 129.10, 129.07, 128.17, 126.72, 126.66, 121.79, 112.78, 112.67, 70.61, 70.16, 68.25, 52.77, 52.22, 21.13. MS (FAB) m/z: 1060 [M – PF6]+. Anal. Calcd for C54H56Co2NF6O14P·2.5H2O: C, 51.85; H, 4.92; N, 1.12. Found: C, 51.87; H, 4.62; N, 1.22.
[2]-[(Dibenzo-24-crown-8){μ-[N-9-anthryl)-N-{4-(phenylethynyl)benzyl}ammonium]hexacarbonyl-dicobalt}]rotaxane Hexafluorophosphate (4f). Yield: 53%; mp > 200 °C. IR ν max (KBr) cm–1 3448, 2920, 2092, 2054, 2024, 1505, 1123, 1105, 1057, 558. 1H NMR (300 MHz, CDCl3) δ 8.47–8.41 (m, 2H), 8.10 (s, 1H), 7.87–7.67 (m, 4H), 7.66–7.33 (m, 13H), 6.68–6.62 (m, 4H), 6.37–6.28 (m, 4H), 5.58–5.48 (m, 2H), 5.35–5.25 (m, 2H), 3.94–3.58 (m, 20H), 3.47–3.36 (m, 4H). 13C NMR (75 MHz, CDCl3) δ 197.18, 175.50, 146.64, 139.02, 137.89, 136.09, 132.87, 130.86, 130.78, 130.76, 129.50, 129.27, 129.16, 128.22, 128.13, 127.50, 127.46, 125.24, 123.56, 121.48, 120.92, 112.03, 71.10, 70.47, 68.03, 55.36, 52.58. MS (FAB) m/z: 1132 [M – PF6]+. Anal. Calcd for C60H56Co2F6NO14P: C, 56.39; H, 4.42; N, 1.10. Found: C, 56.18; H, 4.45; N, 1.13.
[2]-[(Dibenzo-24-crown-8){N-(3,5-dimethylbenzyl)-N-(4-{2-(triethylsilyl)ethenyl}benzyl)ammonium}]rotaxane Hexafluorophosphate (5a) and [2]-[(Dibenzo-24-crown-8){N-(3,5-dimethyl-benzyl)-N-(4-{1-(triethylsilyl)ethenyl}benzyl)ammonium}]rotaxane Hexafluorophosphate (6a). A solution of the cobalt complex 4a (100 mg, 88.5 μmol), bis(trimethylsilyl)acetylene (75.4 mg, 0.443 mmol), triethylsilane (103 mg, 0.885 mmol) in dichloroethane (2.5 mL) was heated at 65 °C for 3 h. After concentration of the reaction mixture, the residue was washed with iPr2O and then purified through chromatography (SiO2; AcOEt) to yield a crude mixture of the vinylsilanes. The crude product was suspended in a mixture of water and acetone [1:1 (v/v), 15 mL] and then ammonium hexafluorophosphate (72.1 mg, 0.443 mmol) was added. The mixture was stirred at rt for 30 min and then the acetone was evaporated; the solid precipitate was filtered off and washed with water to afford a mixture of the vinylsilanes 5a and 6a (45.1 mg, 54%; 5a:6a = 72:28) as a solid. IR ν max (KBr) cm–1 3427, 3145, 2952, 1595, 1455, 1253, 1125, 557. 1H NMR (500 MHz, DMSO-d6) δ 7.64–7.48 (br, 2H), 7.48–7.40 (m, 3H), 7.15–7.11 (m, 0.56H), 7.05–6.82 (m, 12.16H), 6.51 (d, J = 19.2 Hz, 0.72H), 5.91 (d, J = 2.5 Hz, 0.28H), 5.66 (d, J = 2.5 Hz, 0.28H), 4.73–4.65 (m, 2H), 4.57–4.49 (m, 2H), 4.19–4.07 (m, 8H), 3.83–3.73 (m, 8H), 3.60–3.47 (m, 8H), 2.11 (s, 4.32H), 2.09 (s, 1.68H), 1.02 (t, J = 7.9 Hz, 6.48H), 0.92 (t, J = 7.9 Hz, 2.52H), 0.74–0.64 (m, 6H). 13C NMR (75 MHz, CDCl3) δ 147.38, 147.34, 143.59, 139.12, 138.24, 138.20, 131.28, 131.23, 131.11, 130.56, 129.74, 129.61, 129.41, 129.25, 127.54, 126.81, 126.65, 126.58, 126.37, 121.57, 112.53, 70.58, 70.52, 70.06, 68.13, 68.06, 52.56, 52.18, 21.10, 7.36, 7.22, 3.35, 3.15. MS (FAB) m/z: 814 [M – PF6–]+. Anal. Calcd for C48H68F6NO8PSi: C, 60.05; H, 7.14; N, 1.46. Found: C, 59.84; H, 7.02; N, 1.35.
[2]-[(Dibenzo-24-crown-8){N-(3,5-dimethylbenzyl)-N-(4-{2-(triethylsilyl)-1-propenyl)}benzyl)ammonium}]rotaxane Hexafluorophosphate (5b) and [2]-[(Dibenzo-24-crown-8){N-(3,5-dimethyl- benzyl)-N-(4-{1-(triethylsilyl)-1-propenyl)}benzyl)ammonium}]rotaxane Hexafluorophosphate (6b). Yield: 68% (5b:6b = 97:3). IR ν max (KBr) cm–1 3440, 3146, 2912, 1506, 1253, 1123, 1107, 558. 1H NMR (500 MHz, CDCl3) δ 7.67–7.49 (br, 2H), 7.37–7.31 (m, 2H), 7.18–7.12 (m, 2H), 6.95–6.75 (m, 11H), 6.59 (br s, 0.97H), 4.66–4.58 (m, 2H), 4.48–4.40 (m, 2H), 4.18–4.07 (m, 8H), 3.84–3.73 (m, 8H), 3.53–3.42 (m, 8H), 2.14 (s, 5.82H), 2.11 (s, 0.18H), 1.87 (d, J = 1.5 Hz, 2.91H), 0.98 (t, J = 7.9 Hz, 8.73H), 0.89 (t, J = 7.9 Hz, 0.27H), 0.68 (q, J = 7.9 Hz, 5.82H), 0.51 (q, J = 7.9 Hz, 0.18H). 13C NMR (75 MHz, CDCl3) δ 147.39, 139.26, 138.96, 138.26, 137.18, 131.31, 130.60, 129.65, 129.18, 129.00, 126.64, 121.63, 112.57, 70.59, 70.08, 68.12, 52.59, 52.26, 21.13, 17.08, 7.45, 2.47. MS (FAB) m/z: 828 [M – PF6]+. Anal. Calcd for C49H70F6NO8PSi: C, 60.42; H, 7.24; N, 1.44. Found: C, 60.12; H, 7.13; N, 1.35.
[2]-[(Dibenzo-24-crown-8){N-(3,5-Dimethylbenzyl)-N-(4-{2-triethylsilyl-2-phenylethenyl}benzyl)ammonium}]rotaxane Hexafluorophosphate (5c) and [2]-[(Dibenzo-24-crown-8){N-(3,5-Dimethyl- benzyl)-N-(4-{1-triethylsilyl-2-phenylethenyl}benzyl)ammonium}]rotaxane Hexafluorophosphate (6c). Yield: 53% (5c:6c = 68:32). IR ν max (KBr) cm–1 3429, 2925, 1505, 1253, 1124, 558. 1H NMR (300 MHz, CDCl3) δ 7.67–7.50 (br, 2H), 7.50–7.30 (m, 3H), 7.20–6.68 (m, 17H), 4.70–4.23 (m, 4H), 4.20–3.97 (m, 8H), 3.85–3.21 (m, 8H), 3.55–3.40 (m, 8H), 2.20–2.02 (m, 6H), 1.02–0.89 (m, 6.12H), 0.85–0.73 (m, 2.88H), 0.70–0.58 (m, 4.08H), 0.44–0.30 (m, 1.92H). 13C NMR (75 MHz, CDCl3) δ 147.44, 147.38, 145.86, 144.33, 142.93, 142.72, 139.63, 138.43, 138.17, 137.90, 137.25, 131.11, 130.88, 130.66, 130.56, 130.19, 129.65, 129.52, 129.41, 129.33, 128.88, 128.47, 127.89, 127.79, 127.23, 127.13, 126.90, 126.73, 126.59, 125.58, 121.73, 121.64, 112.77, 112.68, 70.56, 70.47, 70.01, 69.95, 68.31, 68.20, 52.79, 52.54, 52.18, 52.09, 21.07, 7.26, 7.25, 2.71, 2.61. MS (FAB) m/z: 890 [M – PF6–]+. HRMS (FAB) m/z: Calcd for C54H72NO8Si [M–PF6]+: 890.5027. Found: 890.5042.
[2]-[(Dibenzo-24-crown-8){(3,5-dimethylbenzyl)(4-{2-(diphenylmethylsilyl)ethenyl}benzyl)ammonium}]rotaxane Hexafluorophosphate (5d) and [2]-[(Dibenzo-24-crown-8){(3,5-dimethyl- benzyl)(4-{1-(diphenylmethylsilyl)ethenyl}benzyl)ammonium}]rotaxane Hexafluorophosphate (6d). Yield: 64% (5d:6d = 63:37). IR ν max (KBr) cm–1 3450, 2922, 1506, 1457, 1253, 1111, 558. 1H NMR (500 MHz, CDCl3) δ 7.65–7.27 (m, 14.52H), 7.25–7.20 (m, 0.74H), 7.12–7.07 (m, 0.74H), 6.92–6.70 (m, 12.26H), 6.12 (d, J = 2.7 Hz, 0.37H), 5.63 (d, J = 2.7 Hz, 0.37H), 4.66–4.61 (m, 1.26H), 4.57–4.52 (m, 0.74H), 4.45–4.33 (m, 2H), 4.17–4.01 (m, 8H), 3.82–3.65 (m, 8H), 3.53–3.27 (m, 8H), 2.14 (s, 3.78H), 2.11 (s, 2.22H), 0.72 (s, 1.89H) 0.66 (s, 1.11H). 13C NMR (75MHz, CDCl3) δ 147.45, 147.37, 145.84, 144.80, 138.67, 138.28, 138.20, 136.08, 135.39, 135.09, 134.82, 132.95, 131.68, 131.22, 131.18, 130.62, 130.56, 130.06, 129.50, 129.40, 129.35, 127.88, 127.27, 126.72, 126.60, 126.51, 121.77, 121.65, 112.61, 112.58, 70.59, 70.44, 70.06, 69.96, 68.16, 68.09, 52.61, 52.54, 52.14, 21.12, 21.09, –3.23, –3.86. MS (FAB) m/z: 896 [M – PF6–]+. Anal. Calcd for C55H66F6NO8PSi: C, 63.39; H, 6.38; N, 1.34. Found: C, 63.23; H, 6.41; N, 1.29.
[2]-[(Dibenzo-24-crown-8){(3,5-dimethylbenzyl)(4-{2-(diphenylmethylsilyl)-1-propenyl)}benzyl)ammonium}]rotaxane Hexafluorophosphate (5e) and [2]-[(Dibenzo-24-crown-8){(3,5-dimethyl- benzyl)(4-{1-(diphenylmethylsilyl)-1-propenyl)}benzyl)ammonium}]rotaxane Hexafluorophosphate (6e). Yield: 56% (5e:6e = 92:8). IR ν max (KBr) cm–1 3429, 2925, 1506, 1253, 1109, 812, 558. 1H NMR (500 MHz, CDCl3) δ 7.63–7.50 (m, 6H), 7.44–7.12 (m, 10H), 6.94–6.73 (m, 11.08H), 6.68–6.63 (m, 0.92H), 4.66–4.59 (m, 1.84H), 4.57–4.50 (m, 0.16H), 4.48–4.40 (m, 1.84H), 4.39–4.32 (m, 0.16H), 4.17–4.03 (m, 8H), 3.84–3.72 (m, 7.36H), 3.71–3.66 (m, 0.64H), 3.54–3.40 (m, 7.36H), 3.40–3.33 (m, 0.32H), 3.30–3.21 (m, 0.32H), 2.14 (m, 5.52H), 2.10 (m, 0.48H), 1.95 (d, J = 1.5 Hz, 2.76H), 1.87 (d, J =1.5 Hz, 0.24H), 0.72 (s, 2.76H), 0.55 (s, 0.24H). 13C NMR (75 MHz, CDCl3) δ 147.34, 140.37, 138.88, 138.27, 137.67, 135.50, 135.11, 131.27, 130.60, 130.10, 129.37, 129.20, 129.03, 127.86, 126.60, 121.61, 112.54, 70.58, 70.07, 68.07, 52.59, 52.21, 21.13, 17.33, –4.53. MS (FAB) m/z: 910 [M – PF6–]+. Anal. Calcd for C56H68F6NO8PSi·H2O: C, 62.61; H, 6.57; N, 1.30. Found: C, 62.89; H, 6.49; N, 1.28.
[2]-[(Dibenzo-24-crown-8){N-(3,5-dimethylbenzyl)-N-(4´-(2-diphenylmethylsilyl)-2-phenylethynyl)enzylammonium}]rotaxane Hexafluorophosphate (5f) and [2]-[(Dibenzo-24-crown-8){N-(3,5- dimethylbenzyl)-N-(4´-(1-diphenylmethylsilyl)-2-phenylethynyl)benzylammonium}]rotaxane Hexafluorophosphate (6f). Yield: 55% (5f:6f = 70:30). IR ν max (KBr) cm–1 3430, 2932, 1506, 1453, 1253, 1109, 558. 1H NMR (500 MHz, DMSO-d6) δ 7.68–7.40 (m, 12H), 7.32–7.12 (m, 4H), 7.07–6.70 (m, 17H), 4.72–4.64 (m, 0.60H), 4.59–4.52 (m, 1.40H), 4.50–4.41 (m, 1.40H), 4.40–4.34 (m, 0.60H), 4.21–4.00 (m, 8H), 3.79–3.63 (m, 8H), 3.54–3.25 (m, 8H), 2.10 (s, 4.20H), 2.08 (s, 1.80H), 0.68 (br s, 0.9H), 0.66 (s, 2.1H), 0.68 (s, 0.9H), 0.66 (s, 2.1H). 13C NMR (75 MHz, CDCl3) δ 147.44, 147.37, 144.46, 141.63, 140.93, 138.22, 136.90, 135.27, 134.91, 131.06, 130.87, 130.67, 130.62, 129.83, 129.72, 129.65, 129.50, 129.46, 129.18, 128.93, 128.63, 128.41, 127.93, 127.84, 127.66, 127.54, 126.83, 126.75, 126.59, 125.93, 121.77, 121.68, 112.86, 112.70, 70.48, 69.96, 68.37, 68.22, 52.62, 52.56, 52.06, 21.10, –4.27. MS (FAB) m/z: 972 [M – PF6–]+. Anal. Calcd for C61H70F6NO8PSi·2H2O: C, 63.47; H, 6.46; N, 1.21. Found: C, 63.58; H, 6.31; N, 1.20.
ACKNOWLEDGMENT
We thank Professor K. Isa (Fukui University) for performing the spectroscopic measurements.
Dedicated to Professor Emeritus Keiichiro Fukumoto on the occasion of his 75th birthday
References
1. For recent reviews on rotaxanes, see: a) V. Balzani, A. Credi, F. M. Raymo, and J. F. Stoddart, Angew. Chem. Int. Ed., 2000, 39, 3348; CrossRef b) R. Ballardini, V. Balzani, A. Credi, M. T. Gandolfi, and M. Venturi, Acc. Chem. Res., 2001, 34, 445; CrossRef c) A. Harada, Acc. Chem. Res., 2001, 34, 456; CrossRef d) C. A. Schalley, K. Beizai, and F. Vögtle, Acc. Chem. Res., 2001, 34, 465; CrossRef e) J.-P. Collin, C. Dietrich-Buchecker, P. Gavina, M. C. Jimenez-Molero, and J.-P. Sauvage, Acc. Chem. Res., 2001, 34, 477; CrossRef f) J.-P. Sauvage and C. Dietrich-Buchecker, Molecular Catenanes, Rotaxanes and Knots, Wiley-VCH, Weinheim, 1999; CrossRef g) J. F. Stoddart, Acc. Chem. Res., 2001, 34, 410; CrossRef h) N. Yui and T. Ooya, Chem. Eur. J., 2006, 12, 6730; CrossRef i) E. R. Kay, D. A. Leigh, and F. Zerbetto, Angew. Chem. Int. Ed., 2007, 46, 72. CrossRef
2. a) S. J. Rowan and J. F. Stoddart, J. Am. Chem. Soc., 2000, 122, 164; CrossRef b) S. J. Rowan, S. J. Cantrill, J. F. Stoddart, A. J. P. White, and D. J. Williams, Org. Lett., 2000, 2, 759; CrossRef c) S.-H. Chiu, S. J. Rowan, S. J. Cantrill, L. Ridvan, P. R. Ashton, R. L. Garrell, and J. F. Stoddart, Tetrahedron, 2002, 58, 807. CrossRef
3. J. S. Hannam, S. M. Lacy, D. A. Leigh, C. G. Saiz, A. M. Z. Slawin, and S. G. Stitchell, Angew. Chem. Int. Ed., 2004, 43, 3260. CrossRef
4. N. Kihara, S. Motoda, T. Yokozawa, and T. Takata, Org. Lett., 2005, 7, 1199. CrossRef
5. We have also developed efficient syntheses of a variety of rotaxanes using ruthenium-catalyzed propargylic substitution. The reactions proceeded via ruthenium-allenylidene complexes as intermediates, formed from the catalyst and the propargylic alcohol; subsequently, various nucleophiles attack the reactive Cδ atom in the intermediate regioselectively. See: Y. Tokunaga, N. Kawai, and Y. Shimomura, Tetrahedron Lett., 2007, 48, 4995. CrossRef
6. D. W. Zehnder II and D. B. Smithrud, Org. Lett., 2001, 3, 2485. CrossRef
7. a) X. Wang, X. Bao, M. McFarland-Mancini, I. Isaacsohn, A. F. Drew, and D. B. Smithrud, J. Am. Chem. Soc., 2007, 129, 7284; CrossRef b) X. Bao, I. Isaacsohn, A. F. Drew, and D. B. Smithrud, J. Org. Chem., 2007, 72, 3988. CrossRef
8. H. Greenfield, H.W. Sternberg, R.A. Friedel, J. H. Wotiz, R. Markby, I. Wender, J. Am. Chem. Soc., 1956, 78, 120. CrossRef
9. For recent reviews on the Pauson-Khand reaction, see: a) K. M. Brummonda and J. L. Kentb, Tetrahedron, 2000, 56, 3263; CrossRef b) A. J. Fletcher and S. D. R. Christie, J. Chem. Soc., Perkin Trans. 1, 2000, 1657; CrossRef c) Y. K. Chung, Coord. Chem. Rev., 1999, 188, 297; CrossRef d) T. Sugihara, M. Yamaguchi, and M. Nishizawa, Chem. Eur. J., 2001, 7, 1589; CrossRef e) L. V. R. Boñaga and M. E. Krafft, Tetrahedron, 2004, 60, 9795. CrossRef
10. For reviews on the Nicolas reaction, see: a) K. M. Nicholas, Acc. Chem. Res., 1987, 20, 207; CrossRef b) B. J. Teobald, Tetrahedron, 2002, 56, 4133. CrossRef
11. a) K. Kira, H. Tanda, A. Hamajima, T. Baba, S. Takai, and M. Isobe, Tetrahedron, 2002, 58, 6485; CrossRef b) S. Hosokawa and M. Isobe, Tetrahedron Lett., 1998, 39, 2609. CrossRef
12. a) K. M. Nicholas and R. Pettit, Tetrahedron Lett., 1971, 37, 3475; CrossRef b) D. Seyferth, M. O. Nestle, and A. T. Wehman, J. Am. Chem. Soc., 1975, 97, 7417. CrossRef
13. I. Aprahamian, O. S. Miljanic, W. R. Dichtel, K. Isoda, T. Yasuda, T. Kato, and J. F. Stoddart, Bull. Chem. Soc. Jpn., 2007, 80, 1856. CrossRef
14. H. Sasabe, N. Kihara, K. Mizuno, A. Ogawa, and T. Takata, Tetrahedron Lett., 2005, 46, 3851. CrossRef
15. H. Sasabe, N. Kihara, K. Mizuno, A. Ogawa, and T. Takata, Chem. Lett., 2006, 35, 212. CrossRef
16. Y. Tokunaga, G. Ohta, Y. Yamauchi, T. Goda, N. Kawai, T. Sugihara, and Y. Shimomura, Chem. Lett., 2006, 35, 766. CrossRef
17. A. Lembo, P. Tagliatesta, and D. M. Guldi, J. Phys. Chem. A, 2006, 110, 11424. CrossRef
18. A. Elangovan, Y.-H. Wang, and T.-I. Ho, Org. Lett., 2003, 5, 1841. CrossRef
19. W. Zhang, S. Kraft, and J. S. Moore, J. Am. Chem. Soc., 2004, 126, 329. CrossRef
20. Kexp = [pseudorotaxane]/[ammonium ion + ammonium salt][DB24C8].
21. The 1H NMR spectra of the crude products of the disubstituted acetylenes revealed the formation of the other products, which we presume were produced from the reaction of 3 with dicobalt octacarbonyl.
22. S. Tojo and M. Isobe, Tetrahedron Lett., 2005, 46, 381. CrossRef
23. a) S. C. Archibald, D. J. Barden, J. F. Y. Bazin, I. Fleming, C. F. Foster, F. Colin, A. K. Mandal, A. K. Mandal, K. Amit, D. Parker, K. Takaki, A. C. Ware, A. R. B. Williams, and A. B. Zwicky, Org. Biomol. Chem., 2004, 2, 1051; CrossRef b) K. Miura and A. Hosomi, Synlett, 2003, 143; c) T. A. Blumenkopf and L. E. Overman, Chem. Rev., 1986, 86, 857 CrossRef