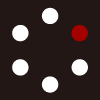
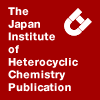
HETEROCYCLES
An International Journal for Reviews and Communications in Heterocyclic ChemistryWeb Edition ISSN: 1881-0942
Published online by The Japan Institute of Heterocyclic Chemistry
e-Journal
Full Text HTML
Received, 31st July, 2008, Accepted, 7th October, 2008, Published online, 9th October, 2008.
DOI: 10.3987/COM-08-S(F)86
■ A New Chiral Synthesis of Wieland-Miescher Ketone Catalyzed by a Combination of (S)-N-Benzyl-N-(2-pyrrolidinylmethyl)amine Derivative and Brønsted Acid
Yuichi Akahane, Kohei Inomata,* and Yasuyuki Endo
Tohoku Pharmaceutical University, 4-4-1 Komatsushima, Aoba-ku, Sendai 981-8558, Japan
Abstract
New or known N-benzyl-N-(2-pyrrolidinylmethyl)amine derivatives bearing a variety of substituents on the aromatic ring were easily prepared from N-Boc-proline or N-Boc-prolinol. The enantioselectivity of the intramolecular asymmetric aldol reaction mediated by a combination of the amine derivative and Brønsted acid to prepare Wieland-Miescher ketone was examined in detail. During the examination, remarkable substitutional effects on the aromatic ring were observed. Development of a catalytic version of the reaction was successfully achieved by the use of N-[(9-anthracenyl)methyl]-N-(2-pyrrolidinylmethyl)amine in the presence of dichloroacetic acid.Wieland-Miescher ketone (2), which was prepared by L-proline (L-Pro)-mediated asymmetric intramolecular aldol reaction of the trione (1), has been a highly useful synthon in total syntheses of a variety of natural products.1,2 This asymmetric aldol reaction has become known as the Hajos-Parrish-Eder-Sauer-Wiechert (HPESW) reaction,3 and has been widely recognized to involve an enamine-based mechanism. In the reaction, a hydrogen bond between an oxygen atom on the cyclohaxane and a carboxylic acid in L-Pro has played a very important role to stabilize a transition state to achieve a highly enantioselective process.4-6 Recently, we have reported that a combination of (S)-2-(pyrrolidinylmethyl)pyrrolidine (4) and TFA successfully mediated the HPESW reaction to afford (R)-Wieland-Miescher ketone [(R)-2] accompanied with 81% ee.7 Strikingly, the process was characterized by an inversion of enantioselectivity when compared with the similar reaction mediated by L-Pro (3). Also, the reaction using 4 without a Brønsted acid afforded (S)-2 with lower enantioselectivity (Scheme 1). These results suggested us that an ammonium moiety in 5 which was produced from 4 and TFA has played a very important role to stabilize a transition state by hydrogen bonds between a mediator and a substrate. This means that an ammonium counterpart in the chiral amine mediator becomes a powerful hydrogen bonding donor working like a carboxylic acid in an amino acid.
In the area of organocatalysis,8 (S)-2-(pyrrolidinylmethyl)pyrrolidine (4) has been employed as a new catalyst to achieve highly efficient asymmetric reactions, including intra- and intermolecular aldol reaction.9 Amine-Brønsted acid catalysis has also been utilized in asymmetric Diels-Alder reaction and 1,4-addition reaction to construct new chiral centers.10, 11 However, to our knowledge, there have been few attempts to use amine catalysts in the intramolecular aldol reaction to construct Wieland-Miescher ketone,12 so our goal was to use some known or new chiral amines based on a structure of L-Pro to synthesize 2 more efficiently. Specifically, we attempted to use known N-benzyl amines (6), which were easily prepared from L-Pro, containing a secondary and/or a tertiary amine for the HPESW reaction (Figure 1). We report here the new reaction conditions mediated by a combination of Brønsted acid and several known or new N-benzylamines.
N-Benzyl-N-(2-pyrrolidinylmethyl)amine derivatives (6) were prepared from N-Boc-L-Pro (7). Thus, amide (8) was obtained by DCC-mediated coupling reaction with 7 and a corresponding N-benzylamine and a followed deprotection of Boc-moiety in the presence of TFA. Lithium aluminium hydride (LAH) reduction of amide group afforded 6a13 and 6b14 respectively. N-Methylpyrrolidine derivative (6c)15 or (6d)16 was easily prepared from 8 by a simple reductive alkylation and a followed LAH reduction (Scheme 2).
We first screened the amines (6) on the aldol reaction of trione (1). All of the reactions were carried out under the same conditions in the presence of a stoichiometric amount of 6 with or without 1.5 equiv. of TFA in DMSO at rt.17 The results were compiled in Table 1. First of all, the aldol reaction mediated by 6a afforded (R)-2 in 47% yield accompanied with 69% ee (entry 2). However, the reaction using 6b bearing a tertiary amino moiety on the side chain afforded (R)-2 in 50% yield accompanied with lower ee (entry 4). The reaction using N-methylpyrrolidine derivative (6c) or (6d) hardly proceeded to afford 2. From these results, a secondary amine on a pyrrolidine ring was required to achieve the effective reaction. All of the reactions, except for using 6d, without TFA afforded (S)-2 with moderate or low ee (Table 1). Since the highest ee value was observed in entry 2, 6a was selected for continued optimization.
We next examined the effects of substituents on the aromatic ring in 6a. Thus, N-benzylamine derivatives (12) bearing a variety of substituents shown in Scheme 3 were prepared via a simple reductive alkylation of a known amine 10, which was easily synthesized from N-Boc-prolinol (9),18 with a corresponding benzaldehyde derivative (11)19 in 35-72% yield from 10 (Scheme 3).
Next, the aldol reactions of 1 using a stoichiometric amount of 12 in the presence of 1.5 equiv. of TFA to yield (R)-2 were carried out. The results were compiled in Table 2. All of the reactions mediated by 12 afforded (R)-2 in moderate yield. Amines (12a-12c) bearing a hydroxyl group on a aromatic ring at o-, m- and p-position, respectively, slightly improved the yield of 2, but no remarkable effects on the enatioselectivity were observed. Mono alkyl- or aryl-substituted benzyl derivative, such as 12d and 12e, and naphthyl derivative 12f afforded slightly higher ee than the case using 6a. These results suggested us that the steric hindrance around the aminomethyl moiety improved the enantioselectivity. However, asymmetrical substituted benzene ring in 12d-12f would not be very effective because the substituent might be far away from C-C bond forming site due to the easy rotation of a single bond. From this aspect, we tried to use the symmetrically substituted benzyl derivatives such as 12g-12j. Among those amines, 12g and 12j were very effective mediators because of the high ee value over 80%. In comparison with entries 8 and 9, 2,6-dimethyl-substituted benzyl amine (12g) was more effective than 3,5-dimethyl- substituted one (12h). These results suggested that steric hindrance near the amine moiety obviously affected the enantioselectivity. However, the reaction mediated by 12i bearing dimethoxy substituents at 2,6-positions required the longer reaction time and hardly affected to the ee value. From these aspects, 12j was selected as the mediator for continued optimization (Table 2).
Finally, we tried to extend this process to be a catalytic version. The reaction using 0.3 equiv of 12j in the presence of 0.45 equiv of TFA in DMSO at rt (entry 2) did not complete even after 48h and afforded (R)-2 with lower yield and lower ee than the stoichiometric reaction (entry 1). Since the catalytic reaction using 12j in the presence of TFA was not successful, the effect of an additive was next probed. Thus, the reaction using a stoichiometric amount of 12j in the presence dichloroacetic acid (DCA) improved the yield of (R)-2 without serious loss of the ee value (entry 3). Although the catalytic reaction shown in entry 4 required longer reaction time, the result was almost same as the stoichiometric reaction (entry 3). Therefore, we have established a catalytic process using a chiral N-benzylamine to prepare (R)-2.
In conclusion, we have established a procedure to prepare N-benzyl-N-(2-pyrrolidinylmethyl)amine derivatives bearing a variety of substituents on the aromatic ring. We also have established a new chiral route to provide (R)-Wieland-Miescher ketone [(R)-2] by using the combination of synthetic chiral amine and Brønsted acid. Furthermore, under optimized conditions, we have achieved the reaction to be a catalytic process. These results may enable the creation of efficient organocatalysts for a wide variety of asymmetric reactions. Further work on a detail of the reaction mechanism and the development of a more efficient catalyst for the reactions is currently in progress.
EXPERIMENTAL
Melting points are uncorrected. IR spectra were recorded on a JASCO-FT-IR-5000 spectrometer. 1H NMR spectra and 13C NMR spectra were recorded on a JEOL-AX-400 (1H 400 MHz, 13C 100 MHz) spectrometer and calibrated using trimethysilane as the internal standard. Mass spectra were recorded on a JEOL-DX-303 or a JEOL-JMS-MS700 spectrometer. Elemental analysis was recorded on a Perkin Elmer CHN-2400 II. Enantiomeric excesses were determined on a Waters-HPLC 600 instrument equipped with a chiral stationary phase column. Optical rotations were measured with a JASCO-DIP-370 digital polarimeter.
Typical procedure of preparation of 6a and 6b
To a stirred solution of N-Boc-L-Proline (7) 5.0 g (23.3 mmol) in CH2Cl2 (50 mL) were added DCC 4.9 g (23.8 mmol) and 1-hydroxybenzotriazole (HOBt) 3.2 g (23.8 mmol) at rt. After stirring at the same temperature for 30 min, N-benzylamine 2.5 g (23.3 mmol) was added and the mixture was further stirred at rt for 24 h. The mixture was filtered through a Celite pad and the filtrate was washed with saturated aqueous NaHCO3 and brine. After drying (Na2SO4), the solvent was removed under reduced pressure to afford crude products. To the stirred solution of the crude products in CH2Cl2 (70 mL) was added TFA 17.7 mL (233 mmol) at rt and the mixture was stirred at the same temperature for 4 h. The mixture was extracted with H2O. The combined aqueous layer was basified by 10% aqueous NaOH and was extracted with CH2Cl2. The combined organic layer was dried (Na2SO4) and the solvent was removed under reduced pressure to afford crude 8a. To a stirred suspension of LAH 1.3 g (35 mmol) in THF (50 mL) was added a solution of crude 8a in THF (20 mL) at 0 oC. The mixture was further heated under reflux for 16 h. 10 % aqueous NaOH (14 mL) was carefully added to the mixture at 0 oC and the mixture was stirred at rt for 2 h. The mixture was filtered through a Celite pad and the filtrate was evaporated under reduced pressure. The residue was chromatographed (CHCl3/MeOH/NH4OH =15/1/0.1) to afford 6a 2.8 g (63% from 7) as yellow oil.
(S)-N-Benzyl-N-(2-pyrrolidinylmethyl)amine (6a)
[α]D20 +14.9 (c 1.01, MeOH), lit.,13 [α]D20 +15.6 (c 1.01, EtOH); IR (film) ν cm-1 3301, 2957, 2871, 1454; 1H-NMR (400 MHz, CDCl3) δ 1.32-1.35 (1H, m), 1.62-1.80 (4H, m), 1.85-1.91 (1H, m), 2.53 (1H, dd, J = 8.8 Hz, 11.4 Hz), 2.63 (1H, dd, J = 4.8 Hz, 11.8 Hz), 2.87-2.92 (2H, m), 3.24 (1H, ddd, J = 4.8 Hz, 7.2 Hz, 15.5 Hz), 3.81 (2H, s), 7.23-7.32 (5H, m); 13C-NMR (100 MHz, CDCl3) δ 25.6, 29.6, 46.4, 54.1, 54.6, 58.2, 126.7, 128.0, 128.2, 140.5; EIMS (m/z) 190 (M+), 70 (100%); HRMS calcd. for C12H18N2 190.1470, found 190.1450.
(S)-N-Benzyl-N-methyl(2-pyrrolidinylmethyl)amine (6b)
Yield 65% (yellow oil); [α]D28 +8.5 (c 1.01, MeOH); IR (film) ν cm-1 3422, 2956, 2788, 1415; 1H-NMR (400 MHz, CDCl3) δ 1.29-1.36 (1H, m), 1.65-1.72 (2H, m), 1.83-1.91 (2H, m), 2.23 (3H, s), 2.26 (1H, dd, J = 4.8 Hz, 12.4 Hz), 2.37 (1H, dd, J = 8.8 Hz, 12.4 Hz), 2.79-2.91 (2H, m), 3.25-3.32 (1H, m), 3.45 (1H, d, J = 13.2 Hz), 3.58 (1H, d, J = 13.2 Hz), 7.23-7.32 (5H, m); 13C-NMR (100 MHz, CDCl3) δ 24.7, 29.5, 42.5, 45.7, 55.8, 62.6, 62.7, 126.8, 128.1, 128.9, 139.2; EIMS (m/z) 204 (M+), 70 (100%); HRMS calcd. for C13H20N2 204.1626, found 204.1633.
Typical procedure of preparation of 6c and 6d
To a stirred solution of crude 8a described above in MeOH (70 mL) was added 37% formalin 2.5 mL (30.3 mmol) at rt. After stirring the mixture at the same temperature for 9 h, NaBH4 444 mg (11.7 mmol) was added as a small portion over 10 min at 0 oC and the mixture was further stirred at the same temperature for 12 h. After adding saturated aqueous NH4Cl, MeOH was removed under reduced pressure. The residue was dissolved to AcOEt and the mixture was washed with saturated aqueous NaHCO3 and brine. The organic layer was dried (Na2SO4) and the solvent was removed under reduced pressure. The residue was dissolved to THF (20 mL) and the solution was added to a stirred suspension of LAH 1.3 g (35 mmol) in THF (50 mL) at 0 oC. The mixture was further heated under reflux for 16 h. After cooling, 10% aqueous NaOH was carefully added at 0 oC and the mixture was filtered through a Celite pad. The filtrate was evaporated under reduced pressure. The residue was chromatographed (CHCl3/MeOH/NH4OH = 15/1/0.1) to afford 6c 2.3 g (48% from 7) as pale yellow oil.
(S)-N-Benzyl-N-methyl(2-pyrrolidinylmethyl)amine (6c)
[α]D29 -57.6 (c 1.01, MeOH); IR (film) ν cm-1 3026, 2942, 2781, 1453; 1H-NMR (400 MHz, CDCl3) δ 1.63-1.78 (1H, m), 1.88-1.94 (1H, m), 2.14-2.21 (1H, m), 2.30 (3H, s), 2.58 (1H, dd, J = 6.4 Hz, 11.6 Hz), 2.74 (1H, dd, J = 4.0 Hz, 11.6 Hz), 3.01-3.05 (1H, m), 3.79 (1H, d, J = 13.6 Hz), 3.84 (1H, d, J = 13.6 Hz), 7.23-7.33 (5H, m); 13C-NMR (100 MHz, CDCl3) δ 22.6, 29.3, 41.1, 52.1, 54.3, 57.5, 65.4, 126.7, 128.0, 128.2, 140.6; EIMS (m/z) 204 (M+), 84 (100%); HRMS calcd. for C13H20N2 204.1626, found 204.1587.
(S)-N-Benzyl-N, N’-dimethyl(2-pyrrolidinylmethyl)amine (6d)
Yield: 51% (yellow oil); [α]D28 -87.7 (c 1.00, MeOH), lit.,16 [α]D -83 (c 1.0, EtOH); IR (film) ν cm-1 2944, 2776, 1452; 1H-NMR (400 MHz, CDCl3) δ 1.52-1.60 (1H, m), 1.66-1.77 (2H, m), 1.96-2.03 (1H, m), 2.12-2.17 (1H, m), 2.20 (3H, s), 2.29-2.35 (2H, m), 2.38 (3H, s), 2.51-2.57 (1H, m), 3.01-3.05 (1H, m), 3.43 (1H, d, J = 13.2 Hz), 3.56 (1H, d, J = 13.2 Hz), 7.23-7.32 (5H, m); 13C-NMR (100 MHz, CDCl3) δ 22.4, 30.6, 41.3, 42.9, 57.6, 62.3, 63.1, 63.8, 126.8, 128.1, 128.9, 139.1; EIMS (m/z) 218 (M+), 84 (100%); HRMS calcd. for C14H22N2 218.1783, found 218.1786.
Typical procedure of preparation of 12
To a stirred solution of 1018 5.5 g, (27.5 mmol) in CH2Cl2 (60 mL) was added salicylaldehyde 3.35 g (27.5 mmol) at rt. After stirring at the same temperature, the solvent was removed under reduced pressure. The residue was dissolved to MeOH (60 mL) and NaBH4 469 mg (12.4 mmol) was added to the mixture as a small portion over 10 min at 0 oC. After stirring at 0 oC for 1.5 h, the solvent was removed under reduced pressure. The residue was dissolved to AcOEt and the mixture was washed with saturated aqueous NaHCO3 and brine. The organic layer was dried (Na2SO4) and the solvent was removed under reduced pressure. The residue was dissolved to CH2Cl2 (100 mL) and TFA 30 mL (275 mmol) was added to the mixture at rt. After stirring at the same temperature for 2 h, the mixture was extracted with H2O. The combined aqueous layer was basified by 10% aqueous NaOH and the mixture was extracted with CH2Cl2. The combined organic layer was dried (Na2SO4) and the solvent was removed under reduced pressure. The residue was chromatographed (CHCl3/MeOH/NH4OH =15/1/0.1) to afford 12a 3.6 g (63% from 10) as colorless oil.
(S)-N-(2-Hydroxybenzyl)-N-(2-pyrrolidinylmethyl)amine (12a)
[α]D26 +14.3 (c 1.01, MeOH); IR (KBr) ν cm-1 3394, 2958, 1454; 1H-NMR (400 MHz, CDCl3) δ 1.34-1.40 (1H, m), 1.66-1.92 (3H, m), 2.50 (1H, dd, J = 8.4 Hz, 11.8 Hz), 2.65 (1H, dd, J = 4.4 Hz, 11.6 Hz), 2.84-2.97 (2H, m), 3.29-3.35 (1H, m), 3.94 (1H, d, J = 14 Hz), 4.06 (1H, d, J = 14 Hz), 4.88 (3H, brm), 6.76 (1H, dt, J = 1.2 Hz, 7.2 Hz), 6.82 (1H, dd, J = 1.2 Hz, 8.0 Hz), 6.98 (1H, d, J = 7.2 Hz), 7.14 (1H, dt, J = 1.4 Hz, 8.0 Hz); 13C-NMR (100 MHz, CDCl3) δ 26.0, 29.7, 46.6, 52.7, 53.8, 57.5, 116.2, 118.8, 122.6, 128.2, 128.5, 158.3; EIMS (m/z) 206 (M+), 70 (100%); HRMS calcd. for C12H18N2O 206.1419, found 206.1423.
(S)-N-(3-Hydroxybenzyl)-N-(2-pyrrolidinylmethyl)amine (12b)
Yield 50% (yellow solid); [α]D24 +14.0 (c 1.00, MeOH); IR (KBr) ν cm-1 3324, 3238, 2967, 1426; 1H-NMR (400 MHz, CDCl3) δ 1.31-1.40 (1H, m), 1.69-1.96 (3H, m), 2.57 (1H, dd, J = 8.8 Hz, 12.0 Hz), 2.72 (1H, dd, J = 4.4 Hz, 11.6 Hz), 2.87-2.98 (2H, m), 3.26-3.33 (1H, m), 3.64 (1H, d, J = 13.2 Hz), 3.70 (1H, d, J = 13.2 Hz), 4.78 (3H, brs), 6.65-6.70 (2H, m), 6.77 (1H, d, J = 2.0 Hz), 7.10 (1H t, J = 8.0 Hz); 13C-NMR (100 MHz, CDCl3) δ 2.53, 29.5, 45.8, 53.5, 53.9, 57.9, 114.9, 115.4, 119.0, 129.5, 141.1, 158.1; EIMS (m/z) 206 (M+), 70 (100%); HRMS calcd. for C12H18N2O 206.1419, found 206.1399.
(S)-N-(4-Hydroxybenzyl)-N-(2-pyrrolidinylmethyl)amine (12c)
Yield: 35% (yellow oil); [α]D25 +12.7 (c 1.01, MeOH); IR (film) ν cm-1 3289, 2966, 1456; 1H-NMR (400 MHz, CDCl3) δ 1.37-1.43 (1H, m), 1.89-1.96 (3H, m), 2.59 (1H, dd, J = 8.8 Hz, 11.6 Hz), 2.72 (1H, dd, J = 4.4 Hz, 12.0 Hz), 2.92-2.99 (2H, m), 3.26-3.33 (1H, m) 3.67 (2H ,s), 4.75 (3H, brs),6.62 (2H, d, J = 8.2 Hz), 7.01 (2H, d, J = 8.2 Hz); 13C-NMR (100 MHz, CDCl3) δ 25.4, 29.6, 45.9, 53.3, 53.4, 57.8, 115.7, 129.5, 129.8, 156.8; EIMS (m/z) 206 (M+), 70 (100%); HRMS calcd. for C12H18N2O 206.1419, found 206.1426.
(S)-N-(2-Methylbenzyl)-N-(2-pyrrolidinylmethyl)amine (12d)
Yield: 52% (yellow oil); [α]D21 +14.9 (c 1.01, MeOH); IR (film) ν cm-1 3300, 2956, 2870, 1404; 1H-NMR (400 MHz, CDCl3) δ 1.32-1.40 (1H, m), 1.68-1.76 (4H, m), 1.83-1.91 (1H, m), 2.35 (3H, s), 2.58 (1H, dd, J = 8.4 Hz, 11.2 Hz), 2.68 (1H, dd, J = 4.4 Hz, 11.2 Hz), 2.87-2.94 (2H, m), 3.26 (1H, ddd, J = 4.8 Hz, 7.4 Hz, 15.1Hz), 3.78 (2H, s), 7.14-7.30 (4H, m); 13C-NMR (100 MHz, CDCl3) δ 18.9, 25.6, 29.5, 46.4, 51.7, 54.8, 58.3, 125.7, 126.7, 128.2, 130.1, 136.2, 138.4; EIMS (m/z) 204 (M+), 70 (100%); HRMS calcd. for C13H20N2 204.1626, found 204.1630.
(S)-N-(2-Phenylbenzyl)-N-(2-pyrrolidinylmethyl)amine (12e)
Yield: 53% (yellow oil); [α]D22 +6.4 (c 1.00, MeOH); IR (film) ν cm-1 3299, 2958, 2870, 1403; 1H-NMR (400 MHz, CDCl3) δ 1.20-1.29 (1H, m), 1.62-1.83 (3H, m), 2.16 (2H, brs), 2.42 (1H, dd, J = 8.4 Hz, 11.6 Hz), 2.51 (1H, dd, J = 4.8 Hz, 11.8 Hz), 2.81-2.91 (2H, m), 3.13 (1H, ddd, J = 4.8 Hz, 7.2 Hz, 15.1 Hz), 3.72 (1H, d, J = 13.2 Hz), 3.77 (1H, d, J = 13.2 Hz), 7.25-7.34 (3H, m), 7.35-7.47 (6H, m); 13C-NMR (100 MHz, CDCl3) δ 25.3, 29.3, 46.2, 51.5, 53.9, 58.2, 126.8, 127.0, 127.4, 128.1, 129.0, 130.0, 137.6, 141.2, 141.7; EIMS (m/z) 266 (M+), 70 (100%); HRMS calcd. for C18H22N2 266.1783, found 266.1787.
(S)-N-(1-Naphthylmethyl)-N-(2-pyrrolidinylmethyl)amine (12f)
Yield: 63% (orange color oil); [α]D22 +8.8 (c 1.00, MeOH); IR (film) ν cm-1 3297, 2956, 1400; 1H-NMR (400 MHz, CDCl3) δ 1.31-1.40 (1H, m), 1.63-1.76 (4H, m), 1.83-1.92 (1H, m), 2.65 (1H, dd, J = 8.4 Hz, 11.2 Hz), 2.76 (1H, dd, J = 4.8 Hz, 11.6 Hz), 2.84-2.94 (2H, m), 3.23-3.30 (1H, m), 4.25 (2H, s), 7.40-7.55 (4H, m), 7.76 (1H, d, J = 8.4 Hz), 7.85 (1H, d, J = 8.0 Hz), 8.14 (1H, d, J = 8.0 Hz); 13C-NMR (100 MHz, CDCl3) δ 25.5, 29.6, 46.3, 51.7, 54.9, 58.3, 123.7, 125.3, 125.5, 126.0, 127.6, 128.6, 131.7, 133.8, 136.0; EIMS (m/z) 240 (M+), 70 (100%); HRMS calcd. for C16H20N2 240.1626, found 240.1629.
(S)-N-(2,6-Dimethylbenzyl)-N-(2-pyrrolidinylmethyl)amine (12g)
Yield: 71% (pale yellow oil); [α]D27 +16.8 (c 1.01, MeOH); IR (film) ν cm-1 3069, 2959, 2923, 2853, 1411; 1H-NMR (400 MHz, CDCl3) δ 1.30-1.39 (1H, m), 1.65-1.79 (4H, m), 1.83-1.91 (1H, m), 2.40 (6H, s), 2.62 (1H, dd, J = 8.2 Hz, 11.4 Hz), 2.70 (1H, dd, J = 4.8 Hz, 11.6 Hz), 2.82-2.95 (2H, m), 3.19-3.25 (1H, m), 3.76 (1H, d, J = 12.1 Hz), 3.80 (1H, d, J = 11.6 Hz), 6.99-7.07 (3H, m); 13C-NMR (100 MHz, CDCl3) δ 19.5, 25.4, 29.5, 46.3, 47.9, 55.2, 58.2, 126.8, 128.1, 136.7, 136.9; EIMS (m/z) 218 (M+), 70 (100%); HRMS calcd. for C14H22N2 218.1783, found 218.1781.
(S)-N-(3,5-Dimethylbenzyl)-N-(2-pyrrolidinylmethyl)amine (12h)
Yield: 60% (pale yellow oil); [α]D28 +10.0 (c 1.00, MeOH); IR (film) ν cm-1 3418, 2920, 1408; 1H-NMR (400 MHz, CDCl3) δ 1.28-1.37 (1H, m), 1.64-1.76 (4H, m), 1.83-1.91 (1H, m), 2.30 (6H, s), 2.53 (1H, dd, J = 8.8 Hz, 11.6 Hz), 2.63 (1H, dd, J = 4.4 Hz, 11.4 Hz), 2.86-2.93 (2H, m), 3.20-3.27 (1H, m), 3.72 (2H, s), 6.88 (1H, s), 6.93 (2H, s); 13C-NMR (100 MHz, CDCl3) δ 21.2, 25.6, 29.6, 46.4, 54.1, 54.7, 58.2, 125.9, 128.4, 137.8, 140.3; EIMS (m/z) 218 (M+), 70 (100%); HRMS calcd. for C14H22N2 218.1783, found 218.1786.
(S)-N-(2,6-Dimethoxylbenzyl)-N-(2-pyrrolidinylmethyl)amine (12i)
Yield: 53% (yellow oil); [α]D19 +6.6 (c 1.00, MeOH); IR (film) ν cm-1 3402, 2938, 2837, 1475; 1H-NMR (400 MHz, CDCl3) δ 1.31-1.38 (1H, m), 1.71-1.78 (2H, m), 1.84-1.91 (1H, m), 2.52 (1H, dd, J = 8.4 Hz, 12.4 Hz), 2.64 (1H, dd, J = 5.6 Hz, 12.0 Hz), 2.92 (2H, t, J = 6.8 Hz), 3.03 (2H, brs), 3.28-3.33 (1H, m), 3.82 (6H, s), 3.89 (2H, s), 6.54 (2H, d, J = 8.8 Hz), 7.18 (1H, t, J = 8.4 Hz); 13C-NMR (100 MHz, CDCl3) δ 25.1, 29.3, 41.3, 45.9, 52.9, 55.6, 58.2, 103.5, 115.8, 128.3, 158.6; EIMS (m/z) 250 (M+), 151 (100%); HRMS calcd. for C14H22N2O2 250.1681, found 250.1683.
(S)-N-(9-Anthracenylmethyl)-N-(2-pyrrolidinylmethyl)amine (12j)
Yield: 72% (yellow oil); [α]D22 +19.0 (c 1.00, MeOH); IR (film) ν cm-1 3302, 2954, 2869, 1445, 1405; 1H-NMR (400 MHz, CDCl3) δ 1.33-1.42 (1H, m), 1.61-1.74 (2H, m), 1.81-1.89 (1H, m), 2.79-2.97 (4H, m), 3.28-3.35 (3H, m), 4.70 (2H, s), 7.42-7.53 (4H, m), 7.99 (2H, d, J = 8.4 Hz), 8.33 (2H, d, J = 8.8 Hz), 8.39 (1H, s); 13C-NMR (100 MHz, CDCl3) δ 25.4, 29.5, 46.0, 46.3, 55.4, 58.3, 124.2, 124.9, 126.0, 127.1, 129.1, 130.3, 131.5, 131.8; EIMS (m/z) 290 (M+), 70 (100%); HRMS calcd. for C20H22N2 290.1783, found 290.1773.
Typical procedure of intramolecular aldol reaction of 1
To a stirred solution of 12j 203 mg (0.70 mmol) and TFA 80 μL (1.05 mmol) in DMSO (1.4 mL) was added 1 137 mg (0.70 mmol) at rt. After stirring at the same temperature for 9 h, the mixture was diluted with AcOEt and the mixture was washed with saturated aqueous NaHCO3 and brine. The organic layer was dried (Na2SO4) and the solvent was removed under reduced pressure. The residue was chromatographed (AcOEt/CH2Cl2/hexane = 1/1/4) to afford (R)-2 63 mg (51%) as pale yellow oil. The optical purity was determined to be 86% ee by HPLC with a chiral stationary phase column. HPLC conditions: Chiralcel OD (Daicel Chemical Industries, LTD), 2-propanol/hexane = 1/10 (v/v), flow rate 1.0 mL/min. detected by UV at 254 nM, tR = 14.6 min for (S)-2, 15.7 min for (R)-2. According to the procedure reported previously,7a the optically pure material (>99% ee) was obtained by a single recrystallization from Et2O/hexane as colorless needles.
M.p. 49.5-50 oC, lit.,4b 48.6-49.4 oC; [α]D25 -100 (c 1.00, benzene), lit.[(S)-2],3l [α]D25 +100 (c 1.40, benzene); IR (film) ν cm-1 1714, 1668; 1H-NMR (400 MHz, CDCl3) δ 1.46 (3H, s), 1.67-1.78 (1H, m), 2.10-2.19 (3H, m), 2.44-2.53 (4H, m), 2.68-2.77 (2H, m), 5.87 (1H, s); 13C-NMR (100 MHz, CDCl3) δ 22.8, 23.2, 29.6, 31.7, 33.5, 37.6, 50.5, 125.8, 165.8, 198.2, 211.0; EIMS (m/z) 178 (M+, 100%); HRMS calcd. for C11H14O2 178.0994, found 178.0988; Anal. Calcd for C11H14O2 C, 74.13; H, 7.92. Found C, 74.03; H, 8.16.
Typical procedure of catalytic intramolecular aldol reaction of 1
To a stirred solution of 12j 65 mg (0.23 mmol) and DCA 28 μL (0.34 mmol) in DMSO (1.4 mL) was added 1 147 mg (0.75 mmol) at rt. After stirring at the same temperature for 20 h, the mixture was diluted with AcOEt and the mixture was washed with saturated aqueous NaHCO3 and brine. The organic layer was dried (Na2SO4) and the solvent was removed under reduced pressure. The residue was chromatographed (AcOEt/CH2Cl2/hexane = 1/1/4) to afford (R)-2 91 mg (68%) as pale yellow oil. The optical purity was determined to be 84% ee by the same procedure described above.
This paper is dedicated to Professor Emeritus Keiichiro Fukumoto on the occasion of his 75th birthday.
References
1. (a) P. Wieland and K. Miescher, Helv. Chim. Acta, 1950, 33, 2215; CrossRef (b) S. Ramachandran and M. S. Newman, Organic Syntheses, 1961, 41, 38; (c) J. Gutzwiller, P. Buchschacher, and A. Fürst, Synthesis, 1977, 167; CrossRef (d) R. Uma, S. Swaminathan, and K. Rajagopalan, Tetrahedron Lett., 1984, 25, 5825; CrossRef (e) G. Ottolina, G. Gonzalo, G. Carrea, and B. Danieli, Adv. Synth. Catal., 2005, 347, 1035; CrossRef (f) Y. Kasai, K. Shimanuki, S. Kuwahara, M. Watanabe, and N. Harada, Chirality, 2006, 18, 177. CrossRef
2. Recent applications of 2: (a) N. Shah and T. S. Scanlan, Bioorg. Med. Chem. Lett., 2004, 14, 5199; CrossRef (b) S. Díaz, A González, B. Bradshaw, J. Cuesta, and J. Bonjoch, J. Org. Chem., 2005, 70, 3749; CrossRef (c) H. Liu, D. R. Siegel, and S. J. Danishefsky, Org. Lett., 2006, 8, 423; CrossRef (d) A. B. Smith, L. Kürti, A. H. Davulcu, and Y. S. Cho, Org. Proc. Res. Dev., 2007, 11, 19; CrossRef (e) K. P. Kaliappan and V. Ravikumar, Org. Lett., 2007, 9, 2417; CrossRef (f) B. Bradshaw, G. Etxebarria-Jardí, and J. Bonjoch, Org. Biomol. Chem., 2008, 6, 772. CrossRef
3. (a) Z. G. Hajos and D. R. Parrish, German Patent DE 2102623, 1971; (b) U. Eder, G. Sauer and R. Wiechert, German Patent DE 2014757, 1971; (c) U. Eder, G. Sauer, and R. Wiechert, Angew. Chem., 1971, 83, 492; CrossRef (d) Z. G. Hajos and D. R. Parrish J. Org. Chem., 1973, 38, 3239; CrossRef (e) Z. G. Hajos and D. R. Parrish, J. Org. Chem., 1974, 39, 1615; CrossRef (f) R. A. Micheli, Z. G. Hajos, N. Cohen, D. R. Parrish, L. A. Portland, W. Sciamanna, M. A. Scott, and P. A. Wehri, J. Org. Chem., 1975, 40, 675; CrossRef (g) P. Buchschacher and A. Fuerst, Organic Syntheses, 1985, 63, 37; (h) Z. G. Hajos and D. R. Parrish, Organic Syntheses, Willey: New York, 1990, Collect. Vol. VII, p. 363; (i) N. Harada, T. Sugioka, H. Uda, and T. Kuriki, Synthesis, 1990, 53; CrossRef (j) D. Rajagopal, K. Rajagopalan, and S. Swaminathan, Tetrahedron: Asymmetry, 1996, 7, 2189; CrossRef (k) T. Bui and C. F. Barbas III, Tetrahedron Lett., 2000, 41, 6951; CrossRef (l) D. Rajagopal, R. Narayanan, and S. Swaminathan, Tetrahedron Lett., 2001, 42, 4887. CrossRef
4. (a) G. Zhong, T. Hoffmann, R. A. Lerner, S. Danishefsky, and C. F. Barbas III, J. Am. Chem. Soc., 1997, 119, 8131; CrossRef (b) K. Fuhshuku, N. Funa, T. Akeboshi, H. Ohta, H. Hosomi, S. Ohba, and T. Sugai, J. Org. Chem., 2000, 65, 129; CrossRef (c) H. Hioki, T. Hashimoto, and M. Kodama, Tetrahedron: Asymmetry, 2000, 11, 829; CrossRef (d) A. Gassama, J. d‘Angelo, C. Cavé, J. Mahuteau, and C. Riche, Eur. J. Org. Chem., 2000, 3165; CrossRef (e) S. G. Davies, R. L. Sheppard, A. D. Smith, and J. E. Thomson, Chem. Commun., 2005, 3802; CrossRef (f) M. Hosseini, N. Stiasni, V. Barbieri, and C. O. Kappe, J. Org. Chem., 2007, 72, 1417; CrossRef (g) S. Sulzer-Mossé, M. Laars, K. Kriis, T. Kanger, and A. Alexakis, Synthesis, 2007, 1729.
5. (a) K. L. Brown, L. Damm, J. D. Dunitz, A. Eschenmoser, R. Hobi, and C. Kratky, Helv. Chim. Acta, 1978, 61, 3108 ; CrossRef (b) C. Agami and H. Sevestre, J. Chem. Soc., Chem. Commun., 1984, 1385; CrossRef (c) C. Agami, C. Puchot, and H. Sevestre, Tetrahedron Lett., 1986, 27, 1501; CrossRef (d) C. Agami and C. Puchot, J. Mol. Catal., 1986, 38, 341; CrossRef (e) C. Agami, N. Platzer, and H. Sevestre, Bull. Soc. Chim. Fr., 1987, 358; (f) C. Agami, Bull. Soc. Chim. Fr., 1988, 499; (g) N. Gathaergood, Austr. J. Chem., 2002, 55, 615. CrossRef
6. (a) S. Bahmanyar and K. N. Houk, J. Am. Chem. Soc., 2001, 123, 11273; CrossRef (b) S. Bahmanyar and K. N. Houk, J. Am. Chem. Soc., 2001, 123, 12911; CrossRef (c) L. Hoang, S. Bahmanyar, K. N. Houk, and B. List, J. Am. Chem. Soc., 2003, 125, 16; CrossRef (d) S. Bahmanyar, K. N. Houk, H. J. Martin, and B. List, J. Am. Chem. Soc., 2003, 125, 2475; CrossRef (e) B. List, L. Hoang, and H. J. Martin, Proc. Nat. Acad. Sci. U. S. A., 2004, 101, 5839; CrossRef (f) B. List, Acc. Chem. Res., 2004, 37, 548; CrossRef (g) C. Allemann, R. Gordillo, F. R. Clemente, P. H.-Y. Cheong, and K. N. Houk, Acc. Chem. Res., 2004, 37, 558; CrossRef (h) P. H-Y. Cheong and K. N. Houk, Synthesis, 2005, 1533.
7. (a) Y. Akahane, N. Inage, T. Nagamine, K. Inomata, and Y. Endo, Heterocycles, 2007, 74, 637; CrossRef (b) T. Nagamine, K. Inomata, and Y. Endo, Heterocycles, in press. CrossRef
8. For reviews: (a) B. List, Tetrahedron, 2002, 58, 5573; CrossRef (b) W. Notz, F. Tanaka, and C. F. Barbas III, Acc. Chem. Res., 2004, 37, 580; CrossRef (c) P. I. Dalko and L. Moisan, Angew. Chem. Int. Ed., 2004, 43, 5138; CrossRef (d) A. Berkessel and H. Gröger, Asymmetric Organocatalysis, Willey-VCH: Weinheim, 2005; CrossRef (e) B. List, Chem. Commun., 2006, 819; CrossRef (f) H. Pellissier, Tetrahedron, 2007, 63, 9267; CrossRef (g) G. Guillena, C. Nájera, and D. J. Ramón, Tetrahedron: Asymmetry, 2007, 18, 2249; CrossRef (h) A. Dondoni and A. Massi, Angew. Chem. Int. Ed., 2008, 47, 4638. CrossRef
9. (a) S. Saito, M. Nakadai, and H. Yamamoto, Synlett, 2001, 1245; CrossRef (b) M. Nakadai, S. Saito, and H. Yamamoto, Tetrahedron, 2002, 58, 8167; CrossRef (c) S. Saito and H. Yamamoto, Acc. Chem. Res., 2004, 37, 570; CrossRef (d) S. Saito, N. Momiyama, and H. Yamamoto, J. Synth. Org. Chem., Jpn 2008, 66, 774.
10. (a) K. Ishihara and K. Nakano, J. Am. Chem. Soc., 2005, 127, 10504; CrossRef and 13079; CrossRef (b) A. Sakakura, K. Suzuki, K. Nakano, and K. Ishihara, Org. Lett., 2006, 8, 2229; CrossRef (c) A. Sakakura, K. Suzuki, and K. Ishihara, Adv. Synth. Catal., 2006, 348, 2457; CrossRef (d) H. Sundén, R. Rios, Y. Xu, L. Eriksson, and A. Córdova, Adv. Synth. Catal., 2007, 349, 2549. CrossRef
11. (a) S. Mossé, M. Laars, M. Kriis, T. Kanger, and A. Alexakis, Org. Lett., 2006, 8, 2559; CrossRef (b) S. Mossé and A. Alexakis, Chem. Commun., 2007, 3123; (c) H. Chen, Y. Wang, S. Wei, and J. Sun, Tetrahedron: Asymmetry, 2007, 18, 1308. CrossRef
12. (a) T. Kanger, K. Kriis, M. Laars, T. Kailas, A-M. Müürisepp, T. Pehk, and M. Lopp, J. Org. Chem., 2007, 72, 5168; CrossRef (b) E. Lacoste, E. Vaique, M. Berlande, I. Pianet, J-M. Vincent, and Y. Landais, Eur. J. Org. Chem., 2007, 167; CrossRef (c) M. Laars, K. Kriis, T. Kailas, A-M. Müürisepp, T. Pehk, T. Kanger, and M. Lopp, Tetrahedron: Asymmetry, 2008, 19. 641. CrossRef
13. M. Amedjkouh and P. Ahlberg, Tetrahedron: Asymmetry, 2002, 13. 2229. CrossRef
14. F. Sternfeld, A. R. Guiblin, R. A. Jelley, V. G. Matassa, A. J. Reeve, P. A. Hunt, M. S. Beer, A. Heald, J. A. Stanton, B. Sohal, A. P. Watt, and L. J. Street, J. Med. Chem., 1999, 42, 677. CrossRef
15. R. H. Reitsema, J. Am. Chem. Soc., 1949, 71, 2041. CrossRef
16. D. Terakado, H. Koutaka, and T. Oriyama, Tetrahedron: Asymmetry, 2005, 16. 1157. CrossRef
17. In our previous report about the aldol reaction using 4, addition of 1.5 equiv. of TFA as a Brønsted acid has afforded a highest enantioselectivity. See ref. 7a.
18. (a) A. Slaitas and E. Yeheskiely, Eur. J. Org. Chem., 2002, 2391; CrossRef (b) N. Dahlin, A. Bøgevig, and H. Adolfsson, Adv. Synth. Catal., 2004, 346, 1101; CrossRef (c) E. Bellis, K. Vasilatou, and G. Kokotos, Synthesis, 2005, 2407.
19. All of the substituted benzaldehyde derivatives have been commercially available