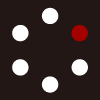
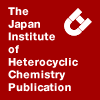
HETEROCYCLES
An International Journal for Reviews and Communications in Heterocyclic ChemistryWeb Edition ISSN: 1881-0942
Published online by The Japan Institute of Heterocyclic Chemistry
e-Journal
Full Text HTML
Received, 31st July, 2008, Accepted, 24th September, 2008, Published online, 24th September, 2008.
DOI: 10.3987/REV-08-SR(F)5
■ Ring Transformations of 2H-Pyran-2-ones and Fused Pyran-2-ones with Nucleophilic Reagents
Franc Pozgan and Marijan Kocevar*
Department of Organic Chemistry, Faculty of Chemistry and Chemical Technology, University of Ljubljana, Askerceva 5, P.O. Box 537, SI-1000 Ljubljana, Slovenia
Abstract
In this review we report on several recently described nitrogen- and carbon-nucleophile-induced ring transformations of selected pyran-2-one derivatives. These reactions provide convenient routes for the synthesis of different heterocycles and carbocycles as well as β-heteroaryl-α,β-didehydroamino acid derivatives.The pyran-2-one ring (1), a six-membered, cyclic, unsaturated ester (lactone), can be found in the form of isolated or fused ring systems in bacterial, microbial, plant and animal systems, demonstrating a whole spectrum of bioactivities like antifungal, antibiotic, cytotoxic, neurotoxic, etc.1 Pyran-2-ones are closely related in structure to pyrylium salts, having five sp2-hybridized carbon atoms. One of the carbon atoms in the pyran ring is derivatised in the form of a carbonyl group, which can be represented in an alternative canonical form, thus forming the pyrylium betaine structure (1b). 2H-Pyran-2-one derivatives find a wide variety of synthetic applications:2 in reactions with electrophiles and nucleophiles, as diene components in Diels-Alder reactions, in photochemical reactions, etc. The question related to the chemistry of pyran-2-ones is “whether the pyran-2-one ring is aliphatic or aromatic in nature”, as it exhibits the characteristic reactions of both alkenes and arenes. The aromatic nature of 2H-pyran-2-ones is demonstrated by their selective substitution patterns in electrophilic reactions, for example, nitration, sulfonation and halogenation, which all occur at the C-3 and C-5 positions. On the other hand, 2H-pyran-2-ones have been found to be vulnerable to nucleophilic attack because of the presence of three electrophilic centers, positions C-2, C-4 and C-6. These reactions usually lead to ring opening and rearrangements, and the pyran-2-one ring is difficult to regenerate; a new heterocylic or carbocyclic system tends to be formed instead.
The present review deals with the recently described ring-transformation reactions of selected pyran-2-one derivatives induced with different nitrogen and carbon nucleophiles. These reactions represent a set of fascinating reactions in heterocyclic chemistry, because the 2H-pyran-2-one ring can be easily converted into a variety of new heterocycles and carbocycles by using simple procedures.
NITROGEN-NUCLEOPHILE-INDUCED RING TRANSFORMATIONS OF 2H-PYRAN-2-ONES AND FUSED PYRAN-2-ONES
5,6,7,8-Tetrahydro-2H-1-benzopyran-2,5-diones (2)3 were converted with ammonia, hydroxylamine, aniline, amino acids, hydrazine and N,N-dimethylhydrazine in boiling ethanol into 2,5-dioxo-1,2,5,6,7,8-hexahydroquinolines (3) (Scheme 1).4 By heating the benzopyrans (2) with an excess of hydrazine hydrate the reaction took place at the pyranone ring and at the 5-oxo group, and even debenzoylation of the benzoylamino group occurred, yielding 3-amino-5-hydrazonoquinolinones (4).
On the other hand, the transformations of benzopyran-2,5-diones (2) with hydrazides, phenylhydrazines and heterocyclic hydrazines in the presence of acidic catalysts (BF3·Et2O, TsOH) gave selectively 5-hydrazonobenzopyrans (5) (Scheme 2).5 The reaction of benzopyran-2,5-diones (2) with aromatic and heteroaromatic hydrazines was remarkably accelerated under microwave conditions in the absence of any catalyst or solvent, thus providing an environmentally friendly route to hydrazones of type 5.6 The latter were further converted into quinoline-2,5-diones (8) in high yields by heating in a mixture of ethanol, water and triethylamine. The same products were also obtained under basic conditions from 2 via the proposed intermediates 6 and 7.7 In a particular case the compound 2 (R1 = R2 = Me) was heated in a mixture of ethanol, water and triethylamine and the compound 6 was isolated (thus confirming the proposed reaction pathway) and further reacted with the corresponding hydrazine derivative yielding the quinolinone product in a direct conversion.
In contrast to its six-membered analogues (2), the cyclopenta[b]pyran-2-one (9) gave, with hydrazine, acetohydrazide and heterocyclic hydrazines in ethanol under acidic conditions, directly cyclopenta[b]pyridine-2(1H)-ones (10) in 62−92% yields without the formation of the corresponding 5-hydrazono derivatives (Scheme 3).5c
To explain the difference between the preferential formation of hydrazones of type 5 in the benzopyran-2-one series and compounds of type 10 from cyclopenta[b]pyran-2-one (9), semi-empirical calculations on these systems were performed, which revealed that for the cyclopenta[b]pyran-2-one system cyclopenta[b]pyridine derivatives (10) are thermodynamically favored over the 5-hydrazone derivatives, whereas for benzopyrans the 5-hydrazono isomers are preferred.5a,c,d It is also important to mention that there is no evidence that thermodynamic parameters are controlling these reactions as the sole factors.
Similarly, the 8-oxobenzopyran derivative (11) in the reaction with nitrogen-containing nucleophiles (hydrazine hydrate, heterocyclic hydrazines, acetyl hydrazine and hydroxylamine) in the presence of an acidic catalyst gave the corresponding 8-hydrazono derivatives (12).8 The ring enlargement via the Schmidt reaction employed on some compounds 12 resulted in the formation of pyrano[2,3-c]azepine (13), which can be further transformed with hydrazine hydrate and phenylhydrazine into pyrido[2,3-c]azepines (14) (Scheme 4).
A totally different chemistry towards hydrazine hydrate is exhibited by pyrano[3,2-c]azepines (15), bearing a free amino group at position 3. Namely, they were shown to give the 1,4-dihydropyridazino[4,3-c]azepines (16) in 53-65% yields as the main products (Scheme 5).9 In addition to the compounds 16, the formation of small quantities of 1,3-diaminopyridin-2-ones (17) and pyridazines (18) was also observed. It was shown that for a successful reaction toward pyridazine derivatives (16), two equivalents of hydrazine hydrate under acidic conditions (TsOH) have to be employed, while with triethylamine as a solvent the pyridine derivative (17) (R1 = R2 = Me) was the major product. The aromatized, fused pyridazines (18) are obviously the oxidation products of 16, caused by the air.
The above reaction enabled the first synthesis of pyridazino[4,3-c]azepine derivatives, and their formation can be explained as outlined in Scheme 6.9 The nucleophilic attack of a hydrazine molecule onto 15 yields an open-ring intermediate, which is then cyclised by the action of the second molecule of hydrazine and the elimination of water and ammonia to give the products 16. The products 17 could be formed by cyclisation with the elimination of water from the first tautomeric intermediate.
In contrast to the behavior of pyrano[3,2-c]azepines (15) in the reaction with hydrazine hydrate, the transformation of the isomeric pyrano[2,3-c]azepine derivative (19) with 5 equivalents of hydrazine hydrate in boiling ethanol led to the 9a-hydroxypyridazino[3,4-c]azepine derivative (20) with an 83% yield (Scheme 7).10 This compound has been found to readily eliminate a water molecule when treated with aqueous HCl in absolute ethanol, thus giving 4,5,6,7,8,9-hexahydro-1H-pyridazino[3,4-c]azepine derivative (21).
Interestingly, pyran-2-ones (22) containing fused polymethylene chains were shown to be more resistant to the nucleophilic attack of hydrazine. Thus, for the successful preparation of 1,4-dihydropyridazines (23) the reaction had to be carried out in boiling hydrazine hydrate, which served as the reagent and as the solvent. Under these conditions, the reaction was finished within 20 minutes and provided the products 23 in good yields (Scheme 8).10 These products are sensible to oxidation in the air, thus giving quantitatively aromatized analogues (24).
The reaction of non-fused 2H-pyran-2-ones (25) with hydrazine hydrate provided a deeper insight into the transformations of pyran-2-ones with hydrazine. Due to the absence of a fused ring in the compounds 25, after the opening of the lactone ring with hydrazine hydrate the formation of a more flexible intermediate 26 (Scheme 9) is expected, which would not be prone to cyclization, thus allowing the attack of additional hydrazine molecules. When the compounds 25 were allowed to react with boiling hydrazine hydrate, 3-hydrazino-2,3,4,5-tetrahydropyridazine derivatives (27) were isolated as the main products.10 In the case of R1 = H and R2 = tert-Bu a small amount of 1,4-dihydropyridazine derivative (28) was also isolated. After refluxing the 2H-pyran-2-one (25) (R1 = Me, R2 = pyridin-2-yl) with 5 equivalents of hydrazine hydrate in ethanol or butanol as a solvent, compound 28 was isolated, which in ethanol was accompanied by the aromatic pyridazine (29).
The compounds 27 can easily eliminate a hydrazine molecule when applying acidic conditions (TsOH) to give the products 28. Here again, 1,4-dihydropyridazines (28) are transformed by oxygen to aromatized analogues (29).
Interestingly, 2-pyridyl-2H-pyran-2-one (30) in the reaction with 5 equivalents of hydrazine hydrate in boiling ethanol led to the isolation of an open-ring intermediate α,δ-dihydrazonohydrazide derivative (31) accompanied by a small quantity of 2,3,4,5-tetrahydropyridazine derivative (32) (Scheme 10).11 On the other hand, when using butanol as a solvent, dihydropyridazine (33) and a small amount of its aromatic analogue (34) were obtained.
A. Sharon et al. reported on a concise synthesis of annulated [a]azaanthracenones (38), thieno[3,2-g]azanaphtalenones (39) and triazacyclopenta[a]anthracenones (40) through a several-step ring transformation of suitably functionalized 2H-pyran-2-ones (35) with α-oxoketene cyclic aminals (36) in the presence of NaH, followed by the photocyclization of the key intermediates (37) and a subsequent oxidation with the air (Scheme 11).12
Similarly, the reaction of 6-aryl-3-cyano-4-methylsulfanyl-2H-pyran-2-one (41) with imidazoliden-2-ylidene nitromethane (42) as the source of an in situ generated anion afforded imidazo[1,2-a]pyridines (43), resulting from the nucleophilic attack of an NH group at position C-6 of the 2H-pyran-2-one ring.13 The competitive reaction of the nucleophile at position C-4 resulted in the 2H-pyran-2-one derivatives (44) (Scheme 12).
2H-Pyran-2-ones (45) were used as synthons for the ring-ring transformation reactions induced with amidines, as reported by Pratap et al.14 Thus, the reaction with formamidine and acetamidine in DMF/KOH yielded 6-aryl substituted pyridines (46) (Scheme 13). The initial step in these reactions is an attack of the nitrogen nucleophile at position C-6 of the 2H-pyran-2-one ring, with ring closure followed by the elimination of carbon dioxide and ammonia to form the products 46.
On the other hand, the same group reported an interesting reaction of 4-secondary-amino-substituted 2H-pyran-2-ones (47) with different salts of arylamidines (48) providing (2,6-diarylpyrimidin-4-yl)acetonitriles (49) (Scheme 14).15 In contrast to the above-mentioned mechanism, the ring closure is accompanied here by the loss of carbon dioxide and a secondary amine (pyrrolidine or piperidine).
4-Methylpyridines (52) and 4-dialkylaminopyridines (53) were synthesized starting from 3-nitro-2H-pyran-2-one acetamidines (50) and secondary amines (51) as the nucleophilic sources (Scheme 15).16 The reaction again starts with a nucleophilic attack at position 6 of the 2H-pyran-2-one ring. Although two competing routes giving two different pyridines, (52) and (53), were observed, the reaction pathways could be controlled toward the prevailing 4-dialkylaminopyridine derivatives (53). Namely, the yield of the pyridine derivative (53) was enhanced when the reaction was performed in ethanol in the presence of TsOH.
SYNTHESIS OF α,β-DIDEHYDRO-α-AMINO ACID (α,β-DDAA) DERIVATIVES THROUGH RING TRANSFORMATIONS OF 2H-PYRAN-2-ONES
Dehydroamino acids and their derivatives play an important role as the constituents of various natural products and biologically active compounds, as well as representing versatile intermediates in organic syntheses.17 Among them, β-heteroaryl-α,β-didehydro-α-amino acid derivatives and compounds containing an amino acid moiety, partly or completely incorporated in the heterocyclic ring, are of current interest.3,18 An important methodology for the preparation of β-heteroaryl-a,β-DDAA derivatives starts from 2H-pyran-2-one derivatives. In contrast to their fused analogues (benzopyran-2,5-diones (2)), 5-acyl-2H-pyran-2-ones (54) do not give the corresponding pyridine derivatives in the reaction with hydrazines and hydroxylamine (Scheme 16). Instead, they react with various hydrazines (55) and hydroxylamine as binucleophiles at the C=O moiety of the 5-acyl group and at the carbon 6 yielding very efficiently (E)- and/or (Z)-α,β-didehydro-α-amino acid derivatives (56), (57) and (59) containing the pyrazolyl or isoxazolyl moiety at the β-position.19 In some cases decarboxylated products 58 were also observed. Under the applied conditions the hydroxylamine yields predominantly (Z)-products 59. The formation of the regioisomeric products 56 and 57 becomes important when R1 = Ph. In this particular case the reaction with hydrazine, methylhydrazine and benzylhydrazine led either to pure products 56 or to mixtures of products 56 and 57. The reaction with phenylhydrazines containing electron-donating groups or weak to moderate electron-withdrawing groups (Me, Cl, CF3, CO2H, etc.) on the phenyl moiety gives almost exclusively (E)-products 57, while phenylhydrazines having strong electron-withdrawing groups as well as heterocyclic hydrazines also yield (Z)-57 and/or decarboxylated products 58.
On the basis of the isolated products two possible attacks of hydrazines to pyran-2-one were proposed, as depicted in Scheme 17.19 Attack (a) at the C=O part of the 5-acyl group would result in the formation of a hydrazone derivative, which would give exclusively (E)-56 in a direct conversion. If the 2H-pyran-2-one ring of the intermediate 60 was opened toward the tautomeric intermediate 61, the expected (E)-56 would also be accompanied by (Z)-56. On the other hand, the attack (b) at position 6-C of the pyran-2-one ring gives the intermediate 62, which can lead to the formation of the products (E)-57 and, via the tautomeric form of the same intermediate, to the formation of (Z)-57. The formation of enamines (58) can be explained by the decarboxylation of the intermediate 62, giving a new tautomeric intermediate 63, which then cyclised into the final products 58.
CARBANION-INDUCED RING TRANSFORMATIONS OF 2H-PYRAN-2-ONES
Carbanions can be easily obtained from Grignard reagents or by the reaction of compounds containing an activated methylene or methyl group with a base; they are strong nucleophiles. As such, they mostly react with the C-6 position of the 2H-pyran-2-one ring, causing a ring opening with possible decarboxylation followed by recyclization, giving different arenes or, depending on the substituents at the carbanion, heteroarenes.
The carbanion-induced ring transformation of 4-amino-substituted 2H-pyran-2-ones (47) with 4-methylpent-3-en-2-one (64) yielded functionally hindered styrene biaryls (65) (Scheme 18).20 This transformation is possibly initiated by the Michael addition of the anion generated from 4-methylpent-3-en-2-one at the C-6 position of the 2H-pyran-2-one ring, followed by intramolecular cyclization involving the carbonyl functionality of 64 and the C-3 position of the pyranone ring to form the intermediate A, which on the elimination of carbon dioxide, followed by protonation and dehydration, furnished the final products 65. On the other side, 2,3,5-trisubstituted cyclopentadienones (66) were prepared through the base-induced ring contraction of 6-aryl-4-amino-2H-pyran-3-carbonitriles (47) using cyanoacetamide, as reported by Sil et al. (Scheme 19).21 A plausible reaction pathway involves the nucleophilic attack of carbanion generated from cyanoacetamide at position C-6 of the 2H-pyran-2-one moiety, followed by the ring opening and by recyclization involving the carboxy group and the active methylene group of the cyanoacetamide with the elimination of water. Though the authors did not succeed to isolate any of the potential intermediates, they proposed a formation and a ring contraction of the cyclic intermediate A, followed by the elimination of the cyanoacetamide affording the cyclopentadienones (66) as the final products.
A different ring contraction was observed in the transformation of 2H-pyran-2-ones (47) by a carbanion generated in situ from nitromethane yielding 2-oxo-2,5-dihydrofuran-3-carbonitriles (67) and (68) (Scheme 20).22 The initial step in the formation of 67 is the attack of the carbanion at position C-6 of the pyran ring, followed by ring opening and relactonization, involving the carboxy group and the C-5 position of the pyran ring with the elimination of the nitro group, as depicted in Scheme 20. In some cases the formation of products 68 was observed, which may have been the result of the partial isomerisation of 67.
The reaction of 2H-pyran-2-one (41) and 5-aryl-3-cyanomethyl-1H-pyrazoles (69) as 1,3-C,N-ambident nucleophiles led to the formation of highly functionalized pyrazolo[1,5-a]pyridines (70) as the major products, while pyrano[4,3-d]pyrazolo[1,5-a]pyridines (71) were obtained as the minor products (Scheme 21).23
The difference in the degree of electrophilicity of the positions C-6 and C-4 and their vulnerability to nucleophiles are the two main reasons for the formation of two different products. The attack of carbanion, formed in situ from cyanomethylpyrazole (69) and KOH as a base, at position C-6 yielded an open-ring intermediate (path (a)) after decarboxylation. The latter underwent a Michael addition with pyrazole nitrogen and the concomitant elimination of methanethiol to give the products 70. On the other hand, the competitive nucleophilic attack at position C-4 (path (b)) followed by ring closure via the cyano group afforded the products 71.
An innovative one-pot synthesis of tetrahydroisoquinolines (74), macrocyclic biaryls (76) and dihydro-1H-isothiochromenes (77) was described by Ram et al.24 These compounds were obtained through base-catalyzed ring-transformation reactions of suitably functionalized 2H-pyran-2-one (72) by a carbanion generated from 4-piperidone (73), cycloalkanone (75) or tetrahydrothiopyran-4-one (Scheme 22). This procedure is very simple and opens up a new avenue for the convenient synthesis of unsymmetrical biaryls.
Similarly, reactions of 2H-pyran-2-ones (72) with benzene-fused cycloalkanone and benzene-fused tetrahydrothiopyran-4-one led to the formation of benzo[c]chromenes and benzo[c]thiochromenes, respectively.25
2H-Pyran-2-ones (72) were used as the precursors for the ring-ring transformation reaction, using 1,1-dimethoxypropan-2-one as the carbanion source; the carbanion was generated in situ by the action of KOH in DMF.26 The reaction is possibly initiated by the attack of the carbanion at position C-6 followed by the concomitant ring closure. In the next step, after decarboxylation and dehydration-substituted benzaldehyde dimethyl acetal (78) is obtained, which on acetal hydrolysis by Amberlyst 15 or ethanolic HCl led to the substituted benzaldehydes (79) (Scheme 23).
On the other hand, the reaction of 2H-pyran-2-one (72) with 6,7-dihydro-5H-benzofuran-4-one and 6,7-dihydro-5H-benzothiophene-4-one provided a one-pot synthesis of diversely functionalized naphtho[2,1-b]furans (80) 27 and naphtho[b]thiophenes (81),28 respectively, as reported by Goel and Dixit (Scheme 24).
Moreover, the 2H-pyran-2-one (72) was converted with (E/Z)-4-phenylbut-3-en-2-one through a ring-ring transformation into highly functionalized (E)-stilbenes (82) (Scheme 25).29 In some cases (X = SMe, Y = CN), the formation of the compounds 82 was accompanied by 4-aryl-6-styrylpyran-2-ylidineacetonitriles (83). The products 82 are formed by the attack of the carbanion at position C-6 of the 2H-pyran-2-one ring of 72 followed by the ring closure and by the liberation of carbon dioxide and water. On the other hand, the products 83 are generated through the attack of a nucleophile at position C-6 followed by decarboxylation and recyclization involving position C-4 of the previous pyran ring and the enolic OH of the intermediate A.
A convenient synthesis of highly functionalized isophthalonitriles (85) and (87) was achieved through a base-catalyzed ring-ring transformation of 6-alkyl/arylpyran-2-ones (84) and 5-arylpyran-2-ones (86) with malononitrile (Scheme 26).30 The strength of the reaction lies in the creation of an aromatic ring from six-membered lactones under mild reaction conditions. This approach is an alternative to the Diels-Alder reactions of 2H-pyran-2-ones with dienophiles, which require forcing thermal conditions to obtain benzene derivatives and offers the flexibility of introducing the electron donating or accepting groups in the benzene scaffold.
Another approach to the synthesis of unsymmetrical biaryls was described in the transformation of 6-aryl-2H-pyran-2-ones or 6-isopropyl-2H-pyran-2-ones with the carbanion generated from 2-methylpentan-3-one or substituted phenylacetones.31 By using this simple method o,m-cymene-cored derivatives were prepared in excellent yields. Biaryls functionalized with electron-withdrawing or electron-donating substituents were prepared from the corresponding pyran-2-ones and acetyltrimethylsilane.32
An efficient route for the synthesis of functionalized oligoarenes and heteroarenes starting from suitably substituted 2H-pyran-2-one (88) and aromatic ketones (89) (1,4-diacetylbenzene, 2,6-diacetylpyridine) was described by Sil et al.33 The first step of this conversion is the formation of quaterarene (90), which was further exploited as a reagent for the ring transformation of the same starting 2H-pyran-2-one (88) to obtain septiarene (91) (Scheme 27). In this way oligoarenes containing only benzene rings (Z = CH) and oligoarenes with a central pyridine ring (Z = N) were prepared.
Similarly, a synthetic methodology for preparing unsymmetrical meta- and para-terphenyls starting from 6-aryl- and 5-aryl-substituted 2H-pyran-2-ones and 2-methoxyacetophenone was developed.34
3-Cyano-6-ferrocenyl-4-methylsulfanyl-2H-pyran-2-one (92) was allowed to react with aliphatic, alicyclic and heterocyclic ketones (93) and (95) under basic conditions to obtain various highly substituted ferrocenylarenes (94) and (96) (Scheme 28).35 This methodology might replace an alternative procedure for the preparation of ferrocenylarenes from ferrocenylalkynes and zirconium cyclopentadiene.36
The carbanion generated in situ at position 4 of 1-aryl-3-methyl-1H-pyrazol-5(4H)-one (97) reacts at position 6 of the pyran-2-one (35) with ring opening and decarboxylation, as depicted in Scheme 29.37 Here, no ring closure occurred, but the initially formed intermediate A tautomerizes to the pure E-isomer of the final product 98. This method enables the synthesis of substituted pyrazol-5(4H)-ones, which may be useful precursors for the construction of various heterocycles.
A concise protocol for the construction of highly congested aryl-substituted 2-hydroxybenzyl alcohols (101) in two steps was developed by Ram’s group.38 The first step is the synthesis of 3-benzyloxy-2-benzyloxymethylbenzonitriles (100) through the base-catalyzed ring transformation of suitably functionalized pyran-2-ones (99) by 1,3-dibenzyloxypropan-2-one (Scheme 30). In the second step, debenzylation by stirring with BCl3 afforded the desired products 101. Similarly, 2-aminobenzylamines (103) as TFA salts were prepared from the same pyran-2-ones and Boc-protected 1,3-diaminopropan-2-one, followed by the TFA-catalyzed hydrolysis of the intermediate 102.39
CONCLUSION
In summary, we have presented some selected ring transformations of suitably functionalized 2H-pyran-2-ones and fused analogues with nitrogen- and carbon-nucleophiles. These reactions have provided convenient routes for the synthesis of different heterocycles and carbocycles as well as α,β-DDAA derivatives applying simple and economical procedures.
ACKNOWLEDGEMENTS
We thank the Ministry of Higher Education, Science and Technology of the Republic of Slovenia and the Slovenian Research Agency for their financial support (P1-0230-0103).
References
1. (a) J. D. Hepworth ‘Comprehensive Heterocyclic Chemistry: Pyrans and Fused Pyrans: (iii) Synthesis and Applications,’ Vol. 3, ed. by A. R. Katritzky and C. W. Rees, Pergamon Press, Oxford, 1984, pp. 737-883; (b) J. D. Hepworth, C. D. Gabbutt, and B. M. Heron, ‘Comprehensive Heterocyclic Chemistry II: Pyrans and their Benzo Derivatives: Synthesis,’ Vol. 5, ed. by A. R. Katritzky, C. W. Rees, and E. F. V. Scriven, Pergamon Press, Oxford, 1996, pp. 351-468; (c) G. R. Geen, J. M. Evans, and A. K. Vong, ‘Comprehensive Heterocyclic Chemistry II: Pyrans and their Benzo Derivatives: Applications,’ Vol. 5, ed. By A. R. Katritzky, C. W. Rees, and E. F. V. Scriven, Pergamon Press, Oxford, 1996, pp. 469-500; (d) K. Afarinkia and M. V. Vinader, ‘Science of Synthesis,’ Vol. 14, ed. by E. J. Thomas, Thieme, Stuttgart, 2003, pp. 275-346; (e) J. D. Hepworth and B. M. Heron, ‘Progress in Heterocyclic Chemistry,’ Vol. 17, ed. by G. W. Gribble and J. A. Joule, Elsevier, Amsterdam, 2005, pp. 362-388; (f) J. D. Hepworth and B. M. Heron, ‘Progress in Heterocyclic Chemistry,’ Vol. 18, ed. by G. W. Gribble and J. A. Joule, Elsevier, Amsterdam, 2007, pp. 376-401.
2. (a) G. P. Ellis, ‘Comprehensive Heterocyclic Chemistry: Pyrans and Fused Pyrans: (ii) Reactivity,’ Vol. 3, ed. by A. R. Katritzky and C. W. Rees, Pergamon Press, Oxford, 1984, pp. 647-736; (b) K. Afarinkia, V. Vinader, T. D. Nelson, and G. H. Posner, Tetrahedron, 1992, 48, 9111; CrossRef (c) B. T. Woodard and G. H. Posner, ‘Advances in Cycloaddition,’ Vol. 5, ed. By M. Harmata, JAI Press Inc., Greenwich, 1999, pp. 47-83; (d) V. J. Ram and P. Srivastava, Curr. Org. Chem., 2001, 5, 571; CrossRef (e) J. D. Hepworth and B. M. Heron, ‘Progress in Heterocyclic Chemistry,’ Vol. 17, ed. by G. W. Gribble and J. A. Joule, Elsevier, Amsterdam, 2005, pp. 33-62.
3. For the synthesis of 3-acylamino substituted 2H-pyran-2-ones and fused pyran-2-ones, see: (a) M. Kocevar, S. Polanc, M. Tisler, and B. Vercek, Synth. Commun., 1989, 19, 1713; CrossRef (b) V. Kepe, M. Kocevar, S. Polanc, B. Vercek, and M. Tisler, Tetrahedron, 1990, 46, 2081; CrossRef (c) M. Kocevar, S. Polanc, B. Vercek, and M. Tisler, Liebigs Ann. Chem., 1990, 501; CrossRef (d) V. Kepe, M. Kocevar, A. Petric, S. Polanc, and B. Vercek, Heterocycles, 1992, 33, 843; CrossRef (e) V. Kepe, M. Kocevar, and S. Polanc, Heterocycles, 1995, 41, 1299; CrossRef (f) V. Kepe, M. Kocevar, and S. Polanc, J. Heterocycl. Chem., 1996, 33, 1707; CrossRef (g) V. Kepe, S. Polanc, and M. Kocevar, Heterocycles, 1998, 48, 671; CrossRef (h) F. Pozgan, K. Kranjc, V. Kepe, S. Polanc, and M. Kocevar, ARKIVOC, 2007, (viii), 97.
4. (a) M. Kocevar, S. Polanc, M. Tisler, and B. Vercek, Heterocycles, 1990, 30, 227; CrossRef (b) P. Trebse, S. Polanc, M. Kocevar, and T. Solmajer, Heterocycles, 1996, 43, 809. CrossRef
5. (a) P. Trebse, S. Polanc, and M. Kocevar, Tetrahedron, 1997, 53, 1383; CrossRef (b) P. Trebse, B. Recelj, M. Kocevar, and S. Polanc, J. Heterocycl. Chem., 1997, 34, 1247; (c) P. Trebse, B. Recelj, T. Lukanc, S. Golic Grdadolnik, A. Petric, B. Vercek, T. Solmajer, S. Polanc, and M. Kocevar, Synth. Commun., 1997, 27, 2637; CrossRef (d) S. Golic Grdadolnik, P. Trebse, M. Kocevar, and T. Solmajer, J. Chem. Inf. Comp. Sci., 1997, 37, 489. CrossRef
6. (a) M. Jeselnik, R. S. Varma, S. Polanc, and M. Kocevar, Chem. Commun., 2001, 1716; (b) M. Jeselnik, R. S. Varma, S. Polanc, and M. Kocevar, Green Chem., 2002, 4, 35. CrossRef
7. P. Trebse, S. Polanc, and M. Kocevar, Heterocycles, 2003, 59, 129. CrossRef
8. P. Trebse, L. Vranicar, I. Music, S. Polanc, W. C. Stevens, and M. Kocevar, Heterocycles, 2000, 53, 1111. CrossRef
9. S. Kafka, P. Trebse, S. Polanc, and M. Kocevar, Synlett, 2000, 254. CrossRef
10. F. Pozgan, S. Polanc, and M. Kocevar, Tetrahedron, 2006, 62, 9718. CrossRef
11. F. Pozgan, S. Kafka, S. Polanc, and M. Kocevar, Heterocycles, 2006, 70, 235. CrossRef
12. A. Sharon, R. Pratap, P. R. Maulik, and V. J. Ram, Tetrahedron, 2005, 61, 3781. CrossRef
13. V. J. Ram, N. Agarwal, A. Sharon, and P. R. Maulik, Tetrahedron Lett., 2002, 43, 307. CrossRef
14. R. Pratap, B. Kumar, and V. J. Ram, Tetrahedron, 2007, 63, 10309. CrossRef
15. R. Pratap, S. P. Kushwaha, A. Goel, and V. J. Ram, Tetrahedron Lett., 2007, 48, 549. CrossRef
16. V. Bertacche, A. Contini, E. Erba, D. Nava, and P. Trimarco, Tetrahedron, 2007, 63, 9652. CrossRef
17. (a) U. Schmidt, A. Lieberknecht, and J. Wild, Synthesis, 1988, 1592; (b) C. Bonauer, T. Walenzyk, B. and König, Synthesis, 2006, 1.
18. For selected recent examples of different types of heterocyclic dehydroamino acid derivatives and their transformations, as well as further applicability of some products thus obtained, see also: (a) I. Music, A. Golobic, and B. Vercek, Heterocycles, 1998, 48, 353; CrossRef (b) M. Dobnikar Tehovnik, A. Petric, and B. Vercek, Acta Chim. Slov., 1996, 43, 133; (c) M. Polak and B. Vercek, Synth. Commun., 2000, 30, 2863; CrossRef (d) T. Trcek, A. Meden, and B. Vercek, Synlett, 2000, 1458; (e) P. M. T. Ferreira, H. L. S. Maia, and L. S. Monteiro, Eur. J. Org. Chem., 2003, 2635; CrossRef (f) T. Trcek and B. Vercek, ARKIVOC, 2003, (xiv), 246; (g) T. Trcek and B. Vercek, ARKIVOC, 2005, (xiv), 96; (h) T. Trcek and B. Vercek, Acta Chim. Slov., 2005, 52, 171; (i) T. Trcek and B. Vercek, Synthesis, 2006, 3437; (j) F. Pozgan, M. Krejan, S. Polanc, and M. Kocevar, Heterocycles, 2006, 69, 123; CrossRef (k) K . Kranjc, M. Kocevar, F. Iosif, S. M. Coman, V. I. Parvulescu, E. Genin, J.-P. Gênet, and V. Michelet, Synlett, 2006, 1075; (l) K. Cucek, A. Golobic, and B. Vercek, Heterocycles, 2007, 73, 555; CrossRef (m) J. Hren, K. Kranjc, S. Polanc, and M. Kocevar, Heterocycles, 2007, 72, 399; CrossRef (n) K. Kranjc and M. Kocevar, Heterocycles, 2007, 73, 481; CrossRef (o) F. Iosif, V. I. Parvulescu, M. E. Pérez-Bernal, R. J. Ruano-Casero, V. Rives, K. Kranjc, S. Polanc, M. Kocevar, E. Genin, J.-P. Gênet, and V. Michelet, J. Mol. Catal. A-Chem., 2007, 276, 34; CrossRef (p) K. Kranjc and M. Kocevar, Bull. Chem. Soc. Jpn., 2007, 80, 2001; CrossRef (r) J. Hren, K. Kranjc, S. Polanc, and M. Kocevar, Synthesis, 2008, 452; (s) K. Kranjc and M. Kocevar, Tetrahedron, 2008, 64, 45; CrossRef (t) K. Cucek and B. Vercek, Synthesis, 2008, 1741.
19. (a) L. Vranicar, S. Polanc, and M. Kocevar, Tetrahedron, 1999, 55, 271; CrossRef (b) L. Vranicar, A. Meden, S. Polanc, and M. Kocevar, J. Chem. Soc., Perkin Trans. 1, 2002, 675; CrossRef (c) L. Vranicar, F. Pozgan, S. Polanc, and M. Kocevar, Amino Acids, 2003, 24, 273. CrossRef
20. A. Kumar, F. V. Singh, and A. Goel, Tetrahedron Lett., 2007, 48, 8223. CrossRef
21. D. Sil, A. Sharon, P. R. Maulik, and V. J. Ram, Tetrahedron Lett., 2004, 45, 6619. CrossRef
22. D. Sil, A. Sharon, P. R. Maulik, and V. J. Ram, Tetrahedron Lett., 2004, 45, 6273. CrossRef
23. M. Nath, P. Srivastava, A. Goel, and V. J. Ram, Eur. J. Org. Chem., 1998, 2083. CrossRef
24. V. J. Ram, N. Agarwal, A. S. Saxena, Farhanullah, A. Sharon, and P. R. Maulik, J. Chem. Soc., Perkin Trans. 1, 2002, 1426.
25. (a) A. Sharon, P. R. Maulik, C. Vithana, Y. Ohashi, and V. J. Ram, Eur. J. Org. Chem., 2004, 886
; CrossRef (b) V. J. Ram, P. Srivastava, and A. S. Saxena, J. Org. Chem., 2001, 66, 5333. CrossRef
26. R. Pratap, D. Sil, and V. J. Ram, Tetrahedron Lett., 2004, 45, 5743. CrossRef
27. A. Goel and M. Dixit, Tetrahedron Lett., 2004, 45, 8819. CrossRef
28. M. Dixit and A. Goel, Tetrahedron Lett., 2006, 47, 3557. CrossRef
29. R. Pratap, R. Kumar, P. R. Maulik, and V. J. Ram, Tetrahedron Lett., 2006, 47, 2949. CrossRef
30. (a) N. Agarwal, A. S. Saxena, Farhanullah, A. Goel, and V. J. Ram, Tetrahedron, 2002, 58, 8793; CrossRef (b) F. V. Singh, V. Kumar, B. Kumar, and A. Goel, Tetrahedron, 2007, 63, 10971. CrossRef
31. F. V. Singh, A. Kumar, and A. Goel, Tetrahedron, 2006, 47, 7767. CrossRef
32. A. Goel, D. Verma, M. Dixit, R. Raghunandan, and P. R. Maulik, J. Org. Chem., 2006, 71, 804. CrossRef
33. D. Sil, A. Goel, and V. J. Ram, Tetrahedron, 2003, 44, 3363. CrossRef
34. A. Goel, D. Verma, and F. V. Singh, Tetrahedron Lett., 2005, 46, 8487. CrossRef
35. D. Sil, Farhanullah, and V. J. Ram, Tetrahedron Lett., 2004, 45, 9025. CrossRef
36. L. Dufkova, H. Matsumura, D. Necas, P. Stepnicka, F. Uhlik, and M. Kotora, Collect. Czech. Chem. Commun., 2004, 69, 351. CrossRef
37. D. Sil, R. Kumar, A. Sharon, P. R. Maulik, and V. J. Ram, Tetrahedron Lett., 2005, 46, 3807. CrossRef
38. Farhanullah, F. Samrin, and V. J. Ram, Tetrahedron Lett., 2007, 48, 8213. CrossRef
39. Farhanullah, F. Samrin, and V. J. Ram, Tetrahedron Lett., 2007, 48, 3187 CrossRef