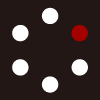
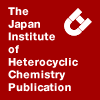
HETEROCYCLES
An International Journal for Reviews and Communications in Heterocyclic ChemistryWeb Edition ISSN: 1881-0942
Published online by The Japan Institute of Heterocyclic Chemistry
e-Journal
Full Text HTML
Received, 16th June, 2008, Accepted, 15th August, 2008, Published online, 18th August, 2008.
DOI: 10.3987/COM-08-S(F)28
■ Semi-quantitative Approaches to the Coordination Ability of o-Bisguanidiniobenzenes with Metal Salts
Koji Suda, Noriko Saito, Takuya Kumamoto, Waka Nakanishi, Masatoshi Kawahata, Kentaro Yamaguchi, Yasumitsu Ogura, Kazuo T. Suzuki, and Tsutomu Ishikawa*
Graduate School of Pharmaceutical Science, Chiba University, 1-33 Yayoi-cho, Inage-ku, Chiba 263-8522, Japan
Abstract
In order to uncover the potential functionality of o-bisguanidinobenzenes the ability of 1,2-bis(1,3-diethylethyleneguanidino)benzene to form complexes with eighteen kinds of metal salts in aqueous media was examined. Complex formation ability was judged by spectral analysis, including elemental analysis, of products obtained as water-insoluble precipitates, as water-soluble and chloroform-extractable materials, or as water-soluble and chloroform-unextractable materials. Positive coordination was observed with many metal salts used. Tight water-insoluble and crystalline complexes were formed in the cases of cobalt(II), zinc(II), and cadmium(II) salts, in the first two cases among which products were analyzable by X-ray crystallography. In addition, treatment of the complex with aqueous alkali re-produced the original bisguanidinobenzene quantitatively, indicating the promising use as a recyclable ligand.INTRODUCTION
Contamination of metals, especially toxic metals, to water has been very serious environmental problems to be solved in global level, together with earth-warming. Use of molecules able to coordinate with metals is one of promising techniques for the removal of metals contaminated in water.1 Thus, although several types of molecules have been applied, it is still important to create new molecules with more efficient coordination ability. It is known that 1,2-diaminobenzene (o-phenylenediamine) can coordinate with a variety of metal salts2; however, the ability is fairly weak and monodentate and / or bidentate types of complexation is available dependent upon the metal used, in spite of expectation of preferable bidentate form due to the adjacent location of two amine units at ortho position on the benzene ring. We have recently developed guanidine chemistry focusing on potential functionality based on the strong basicity of guanidine moiety and reported that introduction of chirality to the guanidine unit(s) led to the creation of new guanidine-type organocatalysts acting as chiral auxiliaries in asymmetric synthesis.3 As an alternative extension of this study, we4 had examined the complexability of 1,2-bis(1,3-dimethylethyleneguanidino)benzene (1), easily derived from 1,2-diaminobenzene and 2-chloro-1,3-dimethylimidazolium chloride (DMC),5 to hydroxyl-containing compounds such as benzoic acid (BA), phenol, and benzyl alcohol and observed the formation of not only 1 : 1 complex crystals between 1 and these three hydroxyl compounds but also additional 1 : 2 and 1 : 4 complex crystals with BA. Furthermore, 1 had been found to form other different ratio of complexes with BA in solution.6 These facts suggest that bisguanidinobenzene-type compounds could also make complexes with metal salts (MXn) through Lewis acid and base interaction by reason of the presence of two strongly basic guanidine moieties (Scheme 1). Therefore we aimed to systematically survey the host ability of the bisguanidinobenzene to form complexes with several kinds of a guest MXn under aqueous conditions and we expected that the experimental results obtained in the use of the corresponding N-ethyl derivative 2, in place of N-methyl one 1, should cover the coordination ability of the original 1 because of more severe steric requirement in the former 2. In this paper we present the possible complexation of the N-ethyl derivative 2 with lots of metal salts examined, among which tight, but reversible, crystalline coordination with cobalt(II), zinc(II), and cadmium(II) salts were observed.
RESULTS AND DISCUSSION
N-Ethyl-substituted bisguanidinobenzene 2 was prepared from imidazolin-2-one (3), after N-ethylation, according to the reported procedure4 (Scheme 2) and then characterized as a hydrogenhexafluorophosphate (HPF6) salt by X-ray crystallographic analysis7 (Figure 1).
Experimental procedure for the coordination tests of 2 with MXn is shown in Figure 2. Water was used as the test medium because of our final aim to remove any toxic metals from polluted water. The possibility of complex formation was stepwise evaluated based on the following facts; (1) firstly, whether new water-insoluble crystalline material (ppt) was precipitated on mixing a water-insoluble bisguanidinobenzene 2 and a water-soluble (or -insoluble) MXn in water or not, (2) secondly, whether complex in aqueous solution is extractable with chloroform (CHCl3) (residue-1) if no (or less) formation of ppt or not, and (3) finally, whether water-soluble material remains in the aqueous mixture (residue-2) or not. Appearance of ppt strongly indicates the formation of a new complex product, except the recovery of a water-insoluble and uncoordinated metal salt. Careful examination is also needed when residue-1 is obtained because the starting water-insoluble 2 should be moved to this fraction in the case of no coordination with MXn. Product in residue-2 strongly suggests to be formed a new water-soluble complex between 2 and MXn.
A stoichiometric suspension of an oily 2 and MXn in water was vigorously stirred at room temperature (rt) for 30 min. If ppt was separated, it was collected by filtration and the filtrate was extracted with CHCl3. Each evaporations of the solvent from the organic solution and from the aqueous solution afforded residue-1 and residue-2, respectively. When no ppt appeared in the aqueous mixture, the mixture was directly extracted with CHCl3. Thus, eighteen MXn shown in Table 1 were tested for coordination ability with 2 and a major product obtained in each operation was basically analyzed by spectroscopic methods, including X-ray analysis in some cases. It should be noted that weight balance between the starting materials and product(s) is not necessarily identical because of possible alternation of the counter anion of the starting MXn during operations.
Sodium and magnesium salts afforded main products in residue-1 as yellow oils, respectively, which were identified with the starting 2 (entries 1-3) except with magnesium iodide (MgI2) (entry 4). In the last case, although a major product was given in ppt as colorless solids (mp 125-128 °C), it was determined to be the monohydroiodide salt of 2 by spectral data and combustion elemental analysis. Thus, it was found that 2 could not be formed complexes with classes-1 and -2 metals under aqueous conditions.
In trials for complexation with cerium chloride (CeCl3) (entry 5), belongs to class-3 metal (lanthanoid series), with zirconium chloride (ZrCl4) (entry 6), belongs to class-4 metal, and with chromium chloride (CrCl2) (entry 7), belongs to class-6 metal, each product was mainly dropped in residue-2 fractions. Formation of a water-soluble product as residue-2 suggested that bisguanidinobenzene 2 may form a complex with these metal salts because of insolubility of the starting 2 in water and possible coordination was strongly supported by elemental analysis of each residue-2 using inductively coupled plasma mass spectroscopy (ICP-MS).
With ferric chloride (FeCl3) (entry 8), belongs to class-8 metal, a major product was also obtained in residue-2, which was solidified to afford reddish brown solids. The presence of iron(III) in the residue-2 was established by atomic absorption spectrophotometry (AAS).
Cobalt chloride (CoCl2), belongs to class-9 metal, was subjected to the coordination test (entry 9). A major product was given in ppt as blue prisms (mp 215-216 °C), which was characterized to be a coordination product by combustion elemental analysis. In addition, X-ray crystallographic analysis was unequivocally established complexation of 2 with CoCl2 through bidentate type coordination8 (Figure 3).
In the cases of nickel chloride (NiCl2) (entry 10) and palladium chloride (PdCl2) (entry 11), belong to class-10 metal, and copper salts (entries 12 and 13), belong to class-11 metal, products were nearly equally distributed to the three fractions. The melting point of ppts (mp >300 °C) suggested that the starting metal salts were recovered. In fact, the elemental analyses of ppts derived from NiCl2 (entry 10) and CuCl2 (entry 12) using ICP-MS showed the nearly quantitative recovery of the starting metal atoms. Furthermore, the same analyses of residues-1 and -2 obtained from PdCl2 (entry 11) and of residue-1 from Cu(OAc)2 (entry 13) also showed no content of these metal atoms. These facts suggest no complex formation of 2 with NiCl2, PdCl2, and copper salts in aqueous media; however, we have independently observed the positive coordination ability of 2 with these metal salts in non-aqueous media.9 Therefore, coordination ability could be affected by the conditions used. In fact, it had been reported that tetramethylguanidine could be formed complexes with not only these three nickel(II), palladium(II), and copper(II) metals but also other cobalt(II), zinc(II), and chromium(III) metals in non-aqueous solvents such as 2,2-dimethoxypropane.10
Easy complexation of 2 with zinc (entries 14 and 15) and cadmium salts (entries 16 and 17), belong to class-12 metals, was deduced by successful precipitation of water-insoluble materials as ppt. With zinc chloride (ZnCl2) (entry 14) and zinc iodide (ZnI2) (entry 15), colorless prisms (mp 190-191 °C) and colorless prisms (mp 248-249 °C), which were completely characterized to be coordination products by spectral data, combustion elemental analysis, and X-ray crystallography, were produced, respectively. Interestingly, comparable crystal structures with different bidentate-type coordination dependent upon counter anion were given; a mirror image structure with ZnCl211 (Figure 4) whereas a C2-symmetrical one with ZnI212 (Figure 5). On the other hand, with cadmium chloride (CdCl2) (entry 16) and cadmium iodide (CdI2) (entry 17), colorless needles (mp 182-183 °C) and colorless prisms (mp 259-260 °C), which were characterized to be coordination products by spectral data, combustion elemental analysis and AAS, were obtained, respectively.
In trial for complexation with indium chloride (InCl3) (entry 18), belongs to class-13 metal, a product was dropped in residue-2 fraction as colorless solid (mp 102-108 °C). Possible coordination was suggested by ICP-MS analysis.
It is important to easily recover the bisguanidinobenzene component from a metal coordination product on respect with its recyclable use as a metal capturer. Thus, when we tried to dissociate each component from a crystalline ZnI2-complex product, as an example, by simple extraction with organic solvent such as toluene under aqueous alkaline conditions, quantitative recovery of 2 was achieved.
CONCLUSION
In conclusion, modification of the amine moiety of 1,2-diaminobenzene to guanidine one, as expected, caused to be able to coordinate with wide ranges of MXn under aqueous conditions due to their strong basicity. Successful X-ray crystallographic analysis of some complexes indicates that bidentate type coordination could be, in general, preferred in the complexation of bisguanidinobenzene with MXn. In addition, the original bisguanidinobenzene was quantitatively recovered from the metal complex by simple extraction. Thus, these findings strongly suggest that bisguanidinobenzene could serve as an effective host ligand for the removal of toxic metals contaminated in water.
EXPERIMENTAL
General experimental procedures. Melting points were determined on a Yanagimoto micro melting point hot-stage instrument and are uncorrected. IR spectra were recorded on a JASCO IR-300 E spectrophotometer. 1H- and 13C-NMR spectra were recorded with JEOL JNM ECP 400 (400 MHz) and 600 (600 MHz) spectrometers with tetramethylsilane as internal reference. EIMS was recorded on a JEOL GC-Mate. FABMS were recorded on a JEOL JMX-HX 110A spectrometer with 3-nitrobenzyl alcohol as a matrix. The concentrations of Cr, Ni, Cu, Zr, Pd, In, and Ce were determined by an ICP-MS (Agilent 7500ce, Agilent Technologies, Hachiouji, Japan) at m/z 52, 60, 65, 90, 105, 115, and 140, respectively, and those of Na, Mg, Fe and Cd were determined by an AAS (Hitachi Z-8000, Hitachi High-Technologies Corporation, Tokyo, Japan). Data collections for X-ray crystallography were performed using a Bruker SMART 1000 CCD area diffractometer with MoKα radiation with an ω-scan mode (λ = 0.71073 Å). The structures were solved by direct methods and refined by full-matrix least-squares refinements based on F2. Empirical absorption corrections were carried out utilizing SADABS routine about bisguanidinobenzene + ZnCl2 complex and bisguanidinobenzene + ZnI2 complex. All non-hydrogen atoms were anisotropically refined. Hydrogen atoms were added geometrically and refined with a riding model. Structure solutions and refinement were performed with the SHELXS-97 and SHELXL-97.13 Anh. CH2Cl2 was purchased from Kanto Chemicals and was used as purchased. Anhydrous benzene was prepared with drying under Na wire. DMF was used after distillation under CaH2.
Synthesis of bisguanidinobenzene 2 and its HPF6 salt.
1,3-Diethylimidazolin-2-one (4). A mixture of imidazolidin-2-one (DMI) (126 mg, 1.5 mmol) and sodium hydride (60% in oil, 122 mg, 3.0 mmol) in DMF (1.9 mL) was stirred at rt for 1.5 h and, then, iodoethane (0.25 mL, 3.1 mmol) was added under ice-cooling. The whole was stirred at rt for 5 h, quenched with cold 10% HCl aq (1 mL), and extracted with CH2Cl2 (3 × 10 mL). The combined organic solutions were washed with water (5 × 2 mL) and brine (2 mL), dried (MgSO4), and evaporated. Distillation of the residual brownish oil at 130 °C / 13 mmHg afforded colorless oil (165 mg, 79%). IR (neat): 1687 (C=O). 1H-NMR (400 MHz, CDCl3): 1.10 (t, J = 7.2 Hz, 2 × Me), 3.24 (q, J = 7.2 Hz, 2 × CH2), 3.28 (s, 2 × CH2). 13C-NMR (100 MHz, CDCl3): 12.7, 38.7, 42.1, 161.0. HREIMS m/z: 142.1104 ([M]+, C7H14N2O; calc. 142.1106).
1,2-Bis(1,3-diethylimidazolideneimino)benzene (2). A solution of 4 (308 mg, 2.2 mmol) and oxalyl chloride (0.95 mL, 11 mmol) in benzene (1.4 mL) was refluxed for 15 h and evaporated to give the crude chloroamidine as a brownish oil, which was used for the next step without further purification. A mixture of 1,2-diaminobenzene (89 mg, 0.79 mmol), the chloroamidine (413 mg, 1.73 mmol), and triethylamine (0.50 mL, 3.59 mmol) in CH2Cl2 (4.4 mL) was stirred under ice-cooling for 40 min. The mixture was extracted with 10% HCl aq (3 × 30 mL). The combined aqueous solutions were washed with toluene (3 × 30 mL), made alkaline to be ca pH >11 with 20% NaOH aq (90 mL), and extracted with toluene (3 × 200 mL). The combined organic solutions were washed with water (5 × 10 mL) and brine (10 mL), dried (MgSO4), and evaporated to give 2 (265 mg, 94%) as a light brown oil. IR (neat): 1654 (C=N). 1H-NMR (400 MHz, CDCl3): 0.99 (t, J = 7.1 Hz, 2 × Me), 3.06 (q, J = 7.1 Hz, 2 × CH2), 3.21 (s, 4 × CH2), 6.70-6.75 (m, 2 × arom. H), 6.75-6.89 (m, 2 × arom. H). 13C-NMR (100 MHz, CDCl3): 12.0, 41.2, 45.0, 120.4, 121.6, 141.9, 151.3. HRFABMS m/z: 357.2776 ([M+H]+, C20H33N6; calc. 357.2767).
The Bis(hexafluorophosphate) Ammonium hexafluorophosphate (147 mg, 0.9 mmol) was added to a suspension of the above 2 (164 mg, 0.45 mmol) in water (1.7 mL) with vigorously stirring. The separated ppt was collected by filtration and recrystallized from methanol to give the salt (111 mg, 38% from phenylenediamine) as colorless prisms, mp 219-220 °C. IR (Nujol): 1637 (C=N). 1H-NMR (400 MHz, acetone-d6): 1.16 (t, J = 7.1 Hz, 4 × Me), 3.37 (q, J = 7.1 Hz, 4 × CH2), 4.02 (s, 4 × CH2), 7.48-7.50 (m, 2 × arom. H), 7.59-7.61 (m, 2 × arom. H). (400 MHz, D2O): 1.13 (t, J = 7.2 Hz, 4 × Me), 3.20 (q, J = 7.2 Hz, 4 × CH2), 3.82 (s, 4 × CH2), 7.43 (br, 2 × arom. H), 7.49 (br, 2 × arom. H). 13C-NMR (100 MHz, CDCl3): 11.8, 41.8, 45.4, 122.2, 123.5, 123.8, 155.4. LRFABMS m/z: 357 ([2+H]+). Anal. Calcd for C20H34F12N2P2: C, 37.04; H, 5.28; N, 12.96; Found: C, 37.10; H, 5.33; N, 12.91.
General procedure for coordination test of 2 with MXn.
A stoichiometric amount of MXn was added to a vigorously stirred suspension of 2 (0.15 mmol) in water (0.3 mL) at rt with one portion and the whole mixture was vigorously stirred at rt for 0.5 h. In the case of suspension, ppt was collected by filtration. The filtrate was extracted with CHCl3 (3 × 2 mL). The combined organic solutions were washed with water (0.5 mL), dried (MgSO4), and evaporated to give residue-1. Evaporation of the aqueous solution afforded residue-2. In the case of solution, the mixture was directly separated according to the above extraction procedure to give residue-1 and / or residue-2.
With MgI2 (Entry 4 in Table 1). Ppt was given as colorless solids, which were recrystallized from ethyl acetate to give the HI salt of 2 as colorless needles, mp 125-128 °C. IR (KBr): 1626 (C=N). 1H-NMR (400 MHz, CDCl3): 1.08 (t, J = 7.2 Hz, 4 × Me), 3.25 (q, J = 7.2 Hz, 4 × CH2), 3.56 (s, 4 × CH2), 6.95-6.99 (m, 2 × arom. H), 7.09-7.11 (m, 2 × arom. H). 13C-NMR (100 MHz, CDCl3): 12.0, 41.9, 45.4, 123.4, 123.7, 135.5, 154.9. LRFABMS m/z: 357 ([M+H]+). Anal. Calcd for C20H33N6I: C, 45.59; H, 6.87; N, 17.35. Found: C, 49.63; H, 7.14; N, 17.23. No Mg atom was detected in the crystal by AAS.
With CeCl3 (Entry 5 in Table 1). Residue-2 was given as a colorless viscous oil. 1H-NMR (400 MHz, D2O): 0.94 (t, J = 7.2 Hz, 4 × Me), 3.01 (q, J = 7.2 Hz, 4 × CH2), 3.66 (s, 4 × CH2), 7.27-7.34 (br, 4 × arom. H). ICP-MS14: Calcd for C20H32CeCl3N6: Ce, 23.24. Found: Ce, 21.85.
With ZrCl4 (Entry 6 in Table 1). Residue-2 was given as colorless solids, mp 175-180 °C. 1H-NMR (400 MHz, D2O): 0.93 (t, J = 7.2 Hz, 4 × Me), 3.00 (q, J = 7.2 Hz, 4 × CH2), 3.66 (s, 4 × CH2), 7.25-7.28 (br, 2 × arom. H), 7.30-7.35 (br, 2 × arom. H). ICP-MS14: Calcd for C20H32N6Cl4Zr: Zr, 15.47. Found: Zr, 13.40.
With CrCl2 (Entry 7 in Table 1). Residue-2 was obtained as colorless solids, mp 145-148 °C. 1H-NMR (400 MHz, CDCl3): 1.15 (t, J = 7.2 Hz, 4 × Me), 3.51 (q, J = 7.2 Hz, 4 × CH2), 3.83 (s, 4 × CH2), 7.33 (br, 4 × arom. H). ICP-MS14: Calcd for C20H32Cl2CrN6: Cr, 10.85. Found: Cr, 6.91.
With FeCl3 (Entry 8 in Table 1). Residue-2 was obtained as reddish brown semi-solids. AAS14: Calcd for C20H32N6Cl3Fe: Fe, 10.77. Found: Fe, 19.71.
With CoCl2·6H2O (Entry 9 in Table 1). Ppt was given as blue prisms, mp 215-216 °C, which were recrystallized from EtOAc. IR (KBr): 1542 (C=N). FABMS m/z: 489 ([M37Cl × 2]+), 487 ([M(37Cl + 35Cl)]+), 485 ([M35Cl × 2]+), 357 ([2+H]+). Anal. Calcd for C20H32Cl2CoN6: 49.39; H, 6.63; N, 17.28. Found: C, 49.21; H, 6.57; N, 17.24.
With ZnCl2 (Entry 14 in Table 1). Ppt was given as colorless prisms, mp 190-191 °C, which were recrystallized from EtOAc. IR (KBr): 1559 (C=N). 1H-NMR (400 MHz, CDCl3): 1.14 (t, J = 7.2 Hz, 4 × Me), 3.36 (q, J = 7.2 Hz, 4 × CH2), 3.59 (s, 4 × CH2), 6.72-6.74 (m, 2 × arom. H), 6.79-6.81 (m, 2 × arom. H). 13C-NMR (100 MHz, CDCl3): 12.1, 42.4, 44.9, 119.7, 120.9, 138.8, 163.0. LRFABMS m/z: 496 ([M68Zn]+), 495 ([M66Zn+1]+), 494 ([M66Zn+1]+), 493 ([M64Zn+1]+), 492 ([M66Zn]+). Anal. Calcd for C20H32Cl2N6Zn: C, 48.74; H, 6.55; N, 17.05. Found: C, 48.82; H, 6.56; N, 16.89.
With ZnI2 (Entry 15 in Table 1). Ppt was given as colorless prisms, mp 248-249 °C, which were recrystallized from EtOAc. IR (KBr): 1542 (C=N). 1H-NMR (400 MHz, CDCl3): 1.13 (t, J = 7.2 Hz, 4 × Me), 3.36 and 3.43 (dq, J = 15.0, 7.2 Hz, 4 × CH2), 3.64 (s, 4 × CH2), 6.70-6.75 (m, 4 × arom. H). 13C-NMR (100 MHz, CDCl3): 12.6, 42.8, 45.0, 119.3, 120.6, 139.0, 164.0. LRFABMS m/z: 678 ([M68Zn]+), 677 ([M66Zn+1]+), 676 ([M66Zn]+), 675 ([M64Zn+1]+), 674 ([M66Zn]+). AAS14: Calcd for C20H32ZnI2N6: Zn, 9.68. Found: Zn, 7.91.
With CdCl2·5/2H2O (Entry 16 in Table 1). Ppt was given as colorless needles, mp 182-183 °C, which were recrystallized from EtOAc. IR (KBr): 1543 (C=N). 1H-NMR (400 MHz, CDCl3): 1.13 (t, J = 7.2 Hz, 4 × Me), 3.29 and 3.35 (dq, J = 14.2, 7.1 Hz, 2 × CH2), 3.56 (s, 4 × CH2), 6.73-6.80 (m, 4 × arom. H). 13C-NMR (150 MHz, CDCl3): 12.1, 42.3, 44.9, 120.7, 121.2, 138.3, 162.7. LRFABMS m/z: 1080 ([2M+]). Anal. Calcd for C20H32CdCl2N6: C, 44.50; H, 5.97; N, 15.57. Found: C, 42.31; H, 5.78; N, 14.50. AAS14: Calcd for C20H32CdCl2N6: Cd, 20.82. Found: Cd, 25.60.
With CdI2 (Entry 17 in Table 1). Ppt was given as colorless prisms, mp 259-260 °C, which were recrystallized from EtOAc. IR (KBr): 1541 (C=N). 1H-NMR (400 MHz, CDCl3): 1.12 (t, J = 7.2 Hz, Me), 3.29 (dq, J = 14.2, 7.1 Hz, 2 × one of CH2), 3.35 (dq, J = 14.2, 7.1 Hz, 2 × one of CH2), 3.59 (s, 8 × CH2), 6.71-6.76 (m, 4 × arom. H). 13C-NMR (100 MHz, CDCl3): 12.6, 42.6, 44.8, 120.7, 120.9, 138.8, 163.5. LRFABMS: 726 ([M116Cd]+), 725 ([M116Cd+1]+), 724 ([M114Cd]+), 723 ([M113Cd]+), 722 ([M112Cd]+), 721 ([M111Cd]+), 720 ([M110Cd]+). Anal. Calcd for C20H32CdI2N6: C, 33.24; H, 4.46; N, 11.63. Found: C, 33.16; H, 4.54; N, 11.52.
With InCl3 (Entry 18 in Table 1). Residue-2 was given as colorless semi-solids, mp 102-108 °C. 1H-NMR (400 MHz, D2O): 0.96 (t, J = 7.2 Hz, 4 × Me), 3.03 (q, J = 7.2 Hz, 4 × CH2), 3.69 (s, 4 × CH2), 7.29-7.37 (br, 4 × arom. H). ICP-MS14: Calcd for C20H32Cl2InN6: In, 19.88. Found : In, 33.85.
Re-generation of Bisguanidinobenzene 2 from the Complex with ZnI2.
The salt (48 mg, 0.07 mmol) was dissolved in MeOH (3 mL) at 60 °C. After cooling to rt, the mixture was basified with 20% NaOH aq (1 mL), stirred at rt for 0.5 h, and extracted with toluene (3 × 12 mL). The combined organic solution was washed with H2O (3 × 1.5 mL) and brine (1.5 mL), dried over Mg2SO4 and evaporated to give pure 2 as a pale yellow oil (29 mg, quant.).
Dedicated to Professor Emeritus Keiichiro Fukumoto on the occasion of his 75th birthday.
References
1. D. Sano, K. Myojo, and T. Omura, Water Sci. Technol., 2006, 53, 221. CrossRef
2. D. A. House, “Comprehensive Coordination Chemistry,” ed by G. Wilkinson, R. D. Gillard, and J. A. McCleverty, Vol. 2, Pergamon Press, 1987, pp. 23-72, Oxford; C. Redshaw, G. Wilkinson, B. Hussain-Bates, and M. B. Hursthouse, J. Chem. Soc., Dalton Trans., 1992, 555; CrossRef C. Redshaw, G. Wilkinson, B. Hussain-Bates, and M. B. Hursthouse, J. Chem. Soc., Dalton Trans., 1992, 1803; CrossRef A. Mederos, S. Dominguez, R. Hernandez-Molina, J. Sanchiz, and F. Brito, Coord. Chem. Rev., 1999, 193-195, 913. CrossRef
3. T. Isobe, K. Fukuda, and T. Ishikawa, Tetrahedron: Asymmetry, 1998, 9, 1729 ; CrossRef T. Isobe, K. Fukuda, Y. Araki, and T. Ishikawa, Chem. Commun., 2001, 243; CrossRef T. Ishikawa, Y. Araki, T. Kumamoto, H. Seki, K. Fukuda, and T. Isobe, Chem. Commun., 2001, 245; CrossRef K. Hada, T. Watanabe, T. Isobe, and T. Ishikawa, J. Am. Chem. Soc., 2001, 123, 7705; CrossRef T. Ishikawa and T. Isobe, Chem. Eur. J., 2002, 8, 552; CrossRef Y. Kitani, T. Kumamoto, T. Isobe, K. Fukuda, and T. Ishikawa, Adv. Synth. Cat., 2005, 347, 1653; CrossRef D. Wannaporn and T. Ishikawa, Molecular Diversity, 2005, 9, 321; CrossRef T. Kumamoto, K. Ebine, M. Endo, Y. Araki, Y. Fushimi, I. Miyamoto, T. Ishikawa, T. Isobe, and K. Fukuda, Heterocycles, 2005, 66, 347; CrossRef T. Haga and T. Ishikawa, Tetrahedron, 2005, 61, 2857; CrossRef D. Wannaporn and T. Ishikawa, J. Org. Chem., 2005, 70, 9399; CrossRef D. Wannaporn, T. Ishikawa, M. Kawahata, and K. Yamaguchi, J. Org. Chem., 2006, 71, 6600. CrossRef
4. M. Kawahata, K. Yamaguchi, and T. Ishikawa, Cryst. Growth Des., 2005, 5, 373. CrossRef
5. T. Isobe and T. Ishikawa, J. Org. Chem., 1999, 64, 5832; CrossRef T. Isobe and T. Ishikawa, J. Org. Chem., 1999, 64, 6984; CrossRef T. Isobe and T. Ishikawa, J. Org. Chem., 1999, 64, 6989. CrossRef
6. M. Kawahata, K. Shikii, H. Seki, T. Ishikawa, and K. Yamaguchi, Chem. Pharm. Bull., 2006, 54, 147. CrossRef
7. Crystal dimensions 0.30 x 0.25 x 0.20 mm3; C20H34F12N6P2, Mr = 648.47; monoclinic C2/c, a = 13.6137(9) Å, b = 9.8866(7) Å, c = 20.8561(14) Å, β = 101.287(1)°, V = 2752.8(3) Å3, Z = 4, Dcalc = 1.565 g·cm-3, T = 173 K, 2715 unique and 3265 observed [I> 2σ (I)] reflections, 187 parameters, final [I> 2σ (I)] R1 = 0.0815, wR2 = 0.2396. S = 1.093. CCDC-673828.
8. Crystal dimensions 0.50 x 0.20 x 0.20 mm3; C20H32Cl2CoN6, Mr = 486.35; monoclinic P21/c, a = 9.0240(9) Å, b = 20.428(2) Å, c = 12.7729(12) Å, β = 97.949(2)°, V = 2331.9(4) Å3, Z = 4, Dcalc = 1.385 g·cm-3, T = 173 K, 4243 unique and 5512 observed [I> 2σ (I)] reflections, 266 parameters, final [I> 2σ (I)] R1 = 0.0346, wR2 = 0.0877. S = 1.075. CCDC-673829.
9. M. Kawahata, K. Yamaguchi, W. Nakanishi, T. Isobe, and T. Ishikawa, unpublisheed results.
10. R. Longhi and R. S. Drago, Inorg. Chem., 1965, 4, 11; CrossRef R. C. Mehrotra, “Comprehensive Coordination Chemistry,” ed by G. Wilkinson, R. D. Gillard, and J. A. McCleverty, Vol. 2, Pergamon Press, 1987, pp. 269-291, Oxford.
11. Crystal dimensions 0.50 x 0.30 x 0.20 mm3; C20H32Cl2N6Zn, Mr = 492.79; orthorhombic Pna21, a = 12.3128(10) Å, b = 16.0623(13) Å, c = 11.8451(9) Å, V = 2342.6(3) Å3, Z = 4, Dcalc = 1.295 g·cm-3, T = 173 K, 4937 unique and 5410 observed [I> 2σ (I)] reflections, 266 parameters, final [I> 2σ (I)] R1 = 0.0269, wR2 = 0.0631. S = 0.996. CCDC-673830.
12. Crystal dimensions 0.50 x 0.40 x 0.40 mm3; C20H32I2N6Zn, Mr = 675.69; monoclinic C2/c, a = 13.4577(13) Å, b = 9.9946(10) Å, c = 19.0356(18) Å, β = 105.484(1)° , V = 2467.4(4) Å3, Z = 4, Dcalc = 1.819 g·cm-3, T = 173 K, 2654 unique and 2898 observed [I> 2σ (I)] reflections, 135 parameters, final [I> 2σ (I)] R1 = 0.0210, wR2 = 0.0559. S = 1.068. CCDC-673831.
13. G. M. Sheldrick, SHELXL97, Program for the Refinement of Crystal Structures, University of Göttingen, Germany, 2007.
14. Molecular formula was tentatively calculated as a complex with the original metal salt.