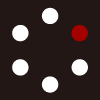
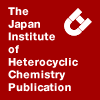
HETEROCYCLES
An International Journal for Reviews and Communications in Heterocyclic ChemistryWeb Edition ISSN: 1881-0942
Published online by The Japan Institute of Heterocyclic Chemistry
e-Journal
Full Text HTML
Received, 4th August, 2008, Accepted, 18th September, 2008, Published online, 22nd September, 2008.
DOI: 10.3987/COM-08-S(F)95
■ Dehalogenation and Barbier-Type Hydroxyalkylation of π-Deficient Haloheterocycles Using Indium
Eri Fukuda, Yukiko Takahashi, Natsumi Hirasawa, Osamu Sugimoto,* and Ken-ichi Tanji
Laboratory of Organic Chemistry, School of Food and Nutritional Sciences, University of Shizuoka, 52-1 Yada, Shizuoka 422-8526, Japan
Abstract
The reaction of π-deficient haloheterocycles with indium metal in water gave corresponding dehalogenated heterocycles. The use of diluted hydrochloric acid instead of water accelerated the reductive reactivity of indium metal. Furthermore, Barbier-type additions proceeded by reactions of α-iodoheterocycles with indium in the presence of pivalaldehyde.Indium (In, atomic number 49) is one of various rare metals discovered by Reich and Richter in 1863. Recently, indium has been widely used in organic syntheses. For example, organic halides react with acetylenes,1 or carbonyl compounds,2 in the presence of indium to create corresponding carbon-carbon bonded products. Iodoalkanes or iodobenzenes are converted into bialkyls or biphenyls by reactions with indium in dry DMF.3,4 It has been reported that nitrogen-containing heterocycles, e. g. quinolines, isoquinolines, quinoxalines, and indoles are reduced by indium in aqueous ethanolic ammonium chloride in order to create dihydro- or tetrahydroheterocycles.5 To our knowledge, however, the study of haloheterocycles as a substrate which uses indium has not been mentioned except in a sole report of the conversion of 2-chloroquinoline into 1,2,3,4-tetrahydroquinoline using indium.5 Here we report the deiodination of nitrogen-containing π-deficient haloheterocycles.6
Pitts et al. have reported that the reaction of 2-chloroquinoline with excess indium in aqueous ethanolic ammonium chloride under refluxing for several days creates 1,2,3,4-tetrahydroquinoline with a 79% yield.5 In this reaction, it has been speculated that the substrate, 2-chloroquinoline is dechlorinated to afford quinoline, which is further reduced to give 1,2,3,4-tetrahydroquinoline because quinoline is converted into 1,2,3,4-tetrahydroquinoline under the same condition. So we planned a selective dehalogenation of haloheterocycles using indium metal.
Initially, reactions of 2-haloquinolines with indium under several conditions were performed as shown in Table 1. A mixture of 2-iodoquinoline and indium powder (10 eq) in aqueous ammonium chloride was heated to reflux for 48 h and produced 1,2,3,4-tetrahydroquinoline (2) with a 73% yield and quinoline (1) with an 8% yield (entry 1). These results are identical to the results reported by Pitts et al. Conversely and interestingly, a selective deiodination proceeded to create 1 under mild conditions such as the use of a stoichiometric amount of indium (entries 2,3), or the use of water as a solvent instead of aqueous ammonium chloride (entries 4,5). Next, the deiodination of 2-iodoquinoline in water was performed with various amounts of indium and the reaction times. It was discovered that 2-iodoquinoline was successfully deiodinated to create 1 with the use of a stoichiometric amount of indium in water for 2 h (entry 7). Dehalogenation of 2-bromoquinoline and 2-chloroquinoline was performed (entries 14,15) in order to discover the different reactivities with 2-iodoquinoline. Although both 2-bromoquinoline and 2-chloroquinoline were dehalogenated to create 1, it was made clear that 2-bromoquinoline and 2-chloroquinoline are less reactive compared to 2-iodoquinoline, and more drastic conditions would be required to dehalogenate successfully. Subsequently, the dechlorination of 2-chloroquinoline using indium in diluted hydrochloric acid was performed (entries 16-21) because it is known that the reactivity of indium is accelerated in acidic solvent. As shown in entries 18 and 19, 2-chloroquinoline was smoothly dechlorinated with 2 eq of indium in 0.5 N hydriodic acid and refluxing for 2-5 h to produce a 75% yield of quinoline.
Table 2 shows the dehalogenation of some haloheterocycles using indium. Water or 0.5 N hydrochloric acid was used as solvents to compare the effects on dehalogenation reactivity. In many cases, the use of 0.5 N hydrochloric acid as a solvent accelerated the dehalogenation of halopyridines and haloquinolines. Especially, chloroquinolines such as N,N-diethyl 2-chloroquinoline-4-carboxamide (entries 7,8) and 4-chloroquinoline (entries 12,13), were dechlorinated smoothly, using 0.5 N hydrochloric acid to produce corresponding dechlorinated products. It should be noted that a reaction of 7-chloro-4-iodoquinoline with indium preceded deiodination at the 4-position of the quinoline ring selectively and dechlorination at the 7-position did not proceed (entries 15,16). This result indicates that ring nitrogen of the quinoline ring affects the reactivity of dehalogenation using indium. Deiodination of iododiazines, 4-iodo-1-phenyl-1H-pyrazolo[3,4-d]pyrimidine (entries 17,18) and 2-iodoquinoxaline (entries 19,20), in 0.5 N hydrochloric acid did not produce any corresponding deiodinated compounds whereas deiodination in water created products in 30% and 44%, respectively. It was found that aqueous hydrochloric acid is not suitable for indium mediated deiodination of iododiazines because diazines tend to dimerize under acidic conditions.
Plausible mechanisms for the dehalogenation of haloheterocycles using indium were shown in Scheme 1. Pitts et al. have reported that quinoline derivatives are reduced to give 1,2,3,4-tetrahydroquinolines under drastic conditions, using excess indium in aqueous ammonium chloride for several days.
There was also speculation that a single electron transfer would proceed from indium to the carbon-nitrogen bond of the quinoline ring. Thus we have speculated that the dehalogenation would proceed through a similar mechanism, that is, a single electron transfer from indium to the carbon-nitrogen bond.
Since π-deficient heterocycles are less reactive with electrophiles, lithiation7-9 are frequently used to introduce electrophiles into π-deficient heterocycles. However, metalation of π-deficient heterocycles usually requires low temperature to avoid some side reactions. Through the examination of this study, we planned the novel introduction of electrophiles into π-deficient heterocycles via a reaction of iodoheterocycles with carbonyl compounds in the presence of indium without protonic solvents such as water. Table 3 shows the reaction of iodopyridines and iodoquinolines with indium in the presence of pivalaldehyde. The reaction of 2-iodoquinoline with indium in the presence of pivalaldehyde under refluxing created the corresponding adduct, 2,2-dimethyl-1-(2-quinolinyl)-1-propanol in moderate yields as well as the deiodinated product, quinoline (entries 1-5). 2-Iodopyridine was also converted to 2,2-dimethyl-1-(2-pyridinyl)-1-propanol using a similar method (entries 6-12). Interestingly, a Barbier-type hydroxyalkylation was facilitated using α-iodoheterocycles (entries 13,14). It is evident that more studies using various kinds of iodoheterocycles are necessary in order to clarify the potentiality and limitation of this reaction.
In conclusion, we have found a novel method of the deiodination of π-deficient haloheterocycles using indium in water to create corresponding dehalogenated heterocycles. The use of diluted hydrochloric acid instead of water accelerated the reductive reactivity of indium. Barbier-type coupling reactions were preceded by the reaction of α-iodoheterocycles with indium in the presence of pivalaldehyde.
EXPERIMENTAL
All melting points were not corrected. 1H-NMR spectra was measured with Hitachi R-90H spectrometer (90 MHz) using tetramethylsilane as an internal standard.
The dehalogenation of haloheterocycles using In in aqueous media (General procedure 1) : A mixture of In, haloheterocycles, and solvent was heated to reflux. The reaction mixture was alkalinized with 1 N NaOH and extracted with EtOAc. The organic layer was dried over Na2SO4 and treated with silica gel column chromatography to give the corresponding product.
1,2,3,4-Tetrahydroquinoline : Eluted with hexane - EtOAc (2:1). Yellow liquids. 1H-NMR (CDCl3), δ : 1.72-2.13 (2H, m, C3-H), 2.60-2.91 (2H, m, C4-H), 3.15-3.47 (2H, m, C2-H), 3.70 (1H, brs, NH), 6.32-6.72 (2H, m, C6 and C8-H), 6.78-7.11 (2H, m, C5 and C7-H).
Quinoline : Eluted with hexane - EtOAc (2:1). Yellow oil. 1H-NMR (CDCl3), δ : 7.38 (1H, dd, J = 8.3 Hz, 4.2 Hz, C3-H), 7.45-7.90 (3H, m, C5, C6, and C7-H), 8.13 (2H, d, J = 8.3 Hz, C4 and C8-H), 8.90 (1H, dd, J = 4.2 Hz, 1.4 Hz, C2-H).
2(1H)-Quinolinone : Eluted with EtOAc-EtOH (20:1). White solids. Mp 199.5-200.5 °C (lit.,10 196-197 °C). 1H-NMR (CDCl3), δ : 6.72 (1H, d, J = 9.5 Hz, C3-H), 7.02-7.32 (1H, m, C6-H), 7.32-7.68 (3H, m, C5, C7, and C8-H), 7.81 (1H, d, J = 9.5 Hz, C4-H), 12.50 (1H, brs, NH).
Nicotinic acid : Eluted with EtOAc-EtOH (4:1). Yellow solids. Mp 237.7-239.1 °C (lit.,11 236-239 °C). 1H-NMR (DMSO-d6), δ : 7.50 (1H, dd, J = 7.6 Hz, 4.7 Hz, C5-H), 8.25 (1H, d, J = 7.6 Hz, C4-H), 8.74 (1H, d, J = 4.7 Hz, C6-H), 9.08 (1H, s, C2-H).
Ethyl nicotinate : Eluted with hexane - EtOAc (1:1). Pale yellow liquids. 1H-NMR (CDCl3), δ : 1.41 (3H, t, J = 7.1 Hz, CH3), 4.42 (2H, q, J = 7.1 Hz, CH2), 7.38 (1H, dd, J = 7.9 Hz, 4.8 Hz, C5-H), 8.29 (1H, dt, J = 7.9 Hz, 1.7 Hz, C4-H), 8.76 (1H, dd, J = 4.8 Hz, 1.7 Hz, C6-H), 9.22 (1H, d, J = 1.7 Hz, C2-H).
2-Diethylaminopyridine : Eluted with hexane - EtOAc (15:1). Slight violet liquids. 1H-NMR (CDCl3), δ : 1.18 (6H, t, J=7.0 Hz, CH3 x 2), 3.51 (4H, q, J=7.0 Hz, CH2 x 2), 6.32-6.57 (2H, m, C3 and C5-H), 7.38 (1H, td, J=7.8 Hz, 1.9 Hz, C4-H), 8.13 (1H, dd, J=5.5 Hz, 1.9 Hz, C6-H).
N,N-Diethyl-4-quinolinecarboxamide : Eluted with EtOAc. White oil. 1H-NMR (CDCl3), δ : 1.02 (3H, t, J = 7.1 Hz, CH3), 1.38 (3H, t, J = 7.1 Hz, CH3), 3.09 (2H, q, J = 7.1 Hz, CH2), 3.43-3.95 (2H, m, CH2), 7.29 (1H, d, J = 4.4 Hz, C3-H), 7.41-7.90 (3H, m, C5, C6, and C7-H), 8.14 (1H, dd, J = 8.4 Hz, 1.5 Hz, C8-H), 8.94 (1H, d, J = 4.4 Hz, C2-H).
7-Chloroquinoline : Eluted with hexane - EtOAc (2:1). Yellow liquids. 1H-NMR (CDCl3), δ : 7.25-7.60 (2H, m, C3 and C6-H), 7.74 (1H, d, J = 8.8 Hz, C5-H), 7.95-8.24 (2H, m, C4 and C8-H), 8.90 (1H, dd, J = 4.2 Hz, 1.6 Hz, C2-H).
1-Phenyl-1H-pyrazolo[3,4-d]pyrimidine : Eluted with hexane - EtOAc (2:1). Pale yellow solids. Mp 75-79 °C (lit.,12 80 °C). 1H-NMR (CDCl3), δ : 7.25-7.70 (3H, m, phenyl-H), 8.08-8.38 (2H, m, phenyl-H), 8.32 (1H, s, C3-H), 9.12 (1H, s, C6-H), 9.26 (1H, s, C4-H).
Quinoxaline : Eluted with hexane - EtOAc (2:1). Brown needles. Mp 28.5 °C (lit.,13 29-30 °C). 1H-NMR (CDCl3), δ : 7.66-7.91 (2H, m, C5 and C8-H), 7.91-8.22 (2H, m, C6 and C7-H), 8.85 (2H, s, C2 and C3-H).
The reaction of iodoheterocycles with In in the presence of pivalaldehyde (General procedure 2) : A mixture of iodoheterocycles, In, and excess pivalaldehyde was heated to reflux. The reaction mixture was diluted with EtOAc, and insoluble solids were filtered off. After the filtrate was diluted with water, the mixture was neutralized with 1 N NaOH and extracted with EtOAc. The organic layer was dried over Na2SO4 and treated with silica gel column chromatography to give the corresponding product.
2,2-Dimethyl-1-(2-quinolinyl)-1-propanol : Eluted with hexane - EtOAc (5:1). Pale yellow solids. Mp 70-72 °C (lit.,12 73-75 °C). 1H-NMR (CDCl3), δ : 0.98 (9H, s, tBu), 4.50 (1H, brs, CH), 4.52-4.85 (1H, br, OH), 7.32 (1H, d, J = 8.6 Hz, C3-H), 7.38-7.66 (1H, m, C6-H), 7.66-7.90 (2H, m, C5 and C7-H), 8.08 (2H, d, J = 8.6 Hz, C4 and C8-H).
2,2-Dimethyl-1-(2-pyridinyl)-1-propanol : Eluted with hexane - EtOAc (1:1). Pale yellow solids. Mp 53-57 °C. 1H-NMR (CDCl3), δ : 0.92 (9H, s, tBu), 4.32 (2H, brs, CHOH), 7.06-7.35 (2H, m, C3 and C5-H), 7.61 (1H, ddd, J = 7.5 Hz, 7.5 Hz, 1.8 Hz, C4-H), 8.57 (1H, dd, J = 5.0 Hz, 1.8 Hz, C6-H).
3-Pivaloylpyridine : Eluted with hexane - EtOAc (5:1). Pale yellow liquids. 1H-NMR (CDCl3), δ : 1.36 (9H, s, tBu), 7.35 (1H, ddd, J = 8.1Hz, 5.2 Hz, 0.5 Hz, C5-H), 8.00 (1H, ddd, J = 7.9 Hz, 2.0 Hz, 2.0 Hz, C4-H), 8.69 (1H, dd, J = 5.8 Hz, 1.5 Hz, C6-H), 8.97 (1H, d, J = 1.8 Hz, C2-H). MS 163 (M+).
2,2-Dimethyl-1-(3-pyridinyl)-1-propanol : Eluted with EtOAc. Pale yellow liquids. 1H-NMR (CDCl3), δ : 0.93 (9H, s, tBu), 4.42 (1H, s, CH), 7.24 (1H, dd, J = 7.8 Hz, 4.7 Hz, C5-H), 7.69 (1H, d, J = 10.0 Hz, C4-H), 8.38-8.63 (2H, m, C2 and C6-H). MS 165 (M+).
This paper is dedicated to Professor Emeritus Keiichiro Fukumoto on the occasion of his 75th birthday.
References
1. R. Yanada, S. Obika, Y. Kobayashi, T. Inokuma, M. Oyama, K. Yanada, and Y. Takemoto, Adv. Synth. Catal., 2005, 347, 1632. CrossRef
2. C. J. Li and T. H. Chan, Tetrahedron Lett., 1991, 32, 7017. CrossRef
3. B. C. Ranu, P. Dutta, and A. Sarkar, Tetrahedron Lett., 1998, 39, 9557. CrossRef
4. Y. M. Chang, S. H. Lee, M. Y. Cho, B. W. Yoo, H. J. Rhee, S. H. Lee, and C. M. Yoon, Synth. Commun., 2005, 35, 1851. CrossRef
5. M. R. Pitts, J. R. Harrison, and C. J. Moody, J. Chem. Soc., Perkin Trans. 1, 2001, 955. CrossRef
6. We have reported the dehalogenation of haloheterocycles using indium in water as a preliminary report, see : N. Hirasawa, Y. Takahashi, E. Fukuda, O. Sugimoto, and K. Tanji, Tetrahedron Lett., 2008, 49, 1492. CrossRef
7. I. Gomez, E. Alonso, D. J. Ramon, and M. Yus, Tetrahedron, 2000, 56, 4043. CrossRef
8. Y. Kondo, M. Shilai, M. Uchiyama, and T. Sakamoto, J. Chem. Soc., Perkin Trans. 1, 1996, 1781.
9. H. Gilman and S. M. Spats, J. Am. Chem. Soc., 1940, 62, 446. CrossRef
10. P. G. Gassman and H. R. Drewes, J. Am. Chem. Soc., 1978, 100, 7600. CrossRef
11. H. Nagano, Y. Nawata, and M. Hamana, Chem. Pharm. Bull., 1987, 35, 4068.
12. O. Sugimoto, M. Sudo, and K. Tanji, Tetrahedron, 2001, 57, 2133. CrossRef
13. H. S. Broadbent, E. L. Allred, L. Pendleton, and C. W. Whittle, J. Am. Chem. Soc., 1960, 82, 189 CrossRef