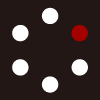
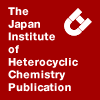
HETEROCYCLES
An International Journal for Reviews and Communications in Heterocyclic ChemistryWeb Edition ISSN: 1881-0942
Published online by The Japan Institute of Heterocyclic Chemistry
e-Journal
Full Text HTML
Received, 25th July, 2008, Accepted, 30th September, 2008, Published online, 2nd October, 2008.
DOI: 10.3987/COM-08-S(F)102
■ Chirality Control of Tropos Diphenylmethane-derived Phosphoramidites by Chiral Dienes: Its Application to Asymmetric Michael Addition
Kazuki Wakabayashi, Kohsuke Aikawa, and Koichi Mikami*
Department of Applied Chemistry, Graduate School of Science and Technology, Tokyo Institute of Technology, 2-12-1 Ookayama, Meguro-ku, Tokyo 152-8552, Japan
Abstract
The Rh complex of tropos diphenylmethane-derived phosphoramidite could be chirally controlled to adopt single chiral conformation upon addition of a chiral diene. In the asymmetric Michael addition of α-cyanocarboxylates catalyzed by the Rh complexes, the chiral diene and bisphenylmethane-derived phosphoramidite functioned to attain higher enantioselectivity and catalytic activity via asymmetric activation.INTRODUCTION
Various asymmetric catalysts with atropisomeric (atropos in Greek)1 ligands have been developed to attain high enantioselectivity.2 For example, Rh complexes with atropos BINOL-derived phosphoramidites were used as catalytically active and enantioselective catalysts for a variety of asymmetric reactions.3 On the other hand, we have reported that chirally flexible (tropos)1 benzophenone-derived ligands can be chirally controlled to a single chiral conformation by a chiral activator to attain higher catalytic activity and enantioselectivity via “asymmetric activation”.2f,g,4 Herein, we report that the Rh complexes with tropos diphenylmethane-derived phosphoramidites 1a-d can be controlled to a single chiral conformation by a chiral diene (Scheme 1) to attain higher catalytic activity and enantioselectivity in the asymmetric Michael addition. Chiral dienes5 could not only control the conformation of Rh complexes with tropos diphenylmethane-derived phosphoramidites but also increase catalytic activity and enantioselectivity of the resultant Rh-phosphoramidite complexes than that with achiral ethylene. In the asymmetric Michael addition, both chiral dienes and diphenylmethane-derived phosphoramidites in the Rh complexes could function to attain higher enantioselectivity and catalytic activity via asymmetric activation.2f,g,6
RESULTS AND DISCUSSION
Complexation of achiral diphenylmethane-derived phosphoramidites 1a-d and [RhCl(C1-D)]2 was found to give single enantiomeric RhCl(C1-D)(phosphoramidite) complex. Even though excess 1a-d was added, only RhCl(C1-D) complexes with one phosphoramidite were obtained along with an excess amount of phosphoramidite remained. In 31P NMR analysis of RhCl(C1-D)(1c), one doublet peak was observed to show that RhCl(C1-D)(1c) possessed a single conformation.
The most stable conformation of RhCl(C1-D)(1c) was deduced by DFT calculation using RhCl(C1-D’)(1c’) as the model (Figure 1).7 The front view shows that the dimethylamino group of 1c’ stays away from the xylyl group of chiral diene C1-D’ and the phosphoramidite (1c’) exists in the opposite side of chiral diene C1-D’. Different from RhCl(C1-D)(1c’), the conformation of phosphoramidite in RhCl(C2’-D’)(1c’) (the model of RhCl(C2’-D)(1c) complex) is determined by the steric repulsion between the diphenylmethane group of 1c’ and the benzyl group of C2’-D’. Therefore, the conformations of phosphoramidite were different between RhCl(C1-D’)(1c’) (upper) and RhCl(C2’-D’)(1c’) (lower). On the other hand, the top right view shows that the phosphoramidite (1c’) adopts a chiral C1-symmetric conformation because of the steric interaction between the diphenylmethane and chiral diene. 1H NMR spectrum of RhCl(C1-D)(1c) indeed showed the C1-symmetric conformation of 1c; 2-tert-Butyl-4-methylphenyl group in the phosphoramidite (1c) exhibited two singlets of the tert-butyl group and two singlets of the methyl group.
There are few reports on the complexes with only single phosphoramidite as highly enantioselective catalysts. For example, the Ir-cod complex with single chiral phosphoramidite has recently been reported to attain higher catalytic activity and enantioselectivity in the asymmetric hydrogenation.8 On the other hand, we found that the Rh-chiral diene complexes with diphenylmethane-derived phosphoramidites attain high catalytic activity in the asymmetric Michael addition of α-cyanocarboxylate.9 The Rh-diene complexes with single phosphoramidite catalyzed the asymmetric Michael addition without dissociation of the chiral diene part. Therefore, the diene part increases the catalytic activity and enantioselectivity. To clarify the effect of the diene part, the asymmetric Michael additions catalyzed by the Rh catalysts of the chiral phosphoramidite (1e) and several achiral dienes were examined (Table 1). RhCl(nbd)(1e) catalyzed the Michael addition even at -78 °C to afford product 3a in 96% yield with 48% ee (entry 1).10 1,5-Cycloocatadiene (cod) and 1,5-dimethylcycloocatadiene (DM-COD) gave lower enantioselectivities (entries 4 and 5) but 1,2-dibromonorbornadiene (Br-nbd) provided higher enantioselectivity (entry 3). These results indicate that six-membered bicyclic dienes attained higher enantioselectivity than eight-membered monocyclic dienes.
The Rh complexes with chiral six-membered bicyclic diene C1-D and C2’-D were thus used as asymmetric catalysts (Table 2). Chiral dienes C1-D and C2’-D controlled the conformation of achiral phosphoramidite to attain higher enantioselectivity in the asymmetric Michael addition. Low catalytic activity of RhCl(ethylene)2 (1a) (entry 3) clearly shows that both phosphoramidites and chiral dienes exert to give higher catalytic activity (entries 4 and 5).
The Rh-chiral diene (C1-D) complexes with the achiral phosphoramidite (1a) attained higher enantioselectivity than Rh-chiral diene (C2’-D) complexes (entries 4 vs. 5). The 4-methyl group of the phosphoramidite (1a) did not affect the enantioselectivity, but the bulky 2-tert-butyl group of the phosphoramidite (1c) attained higher enantioselectivity (entries 4 vs. 7). Furthermore, the asymmetric Michael addition of 2-cyanopropionic acid tert-butyl ester catalyzed by RhCl(C1-D)(1c) gave product 3b in 95% yield with 86% ee (entry 9). However, the phosphoramidite (1d) bearing benzyl amine and tert-butyl group was too bulky to decrease the catalytic activity and enantioselectivity (entry 10).
The absolute configuration of products could be dictated by the chiral dienes (entries 4 vs. 5 and 7 vs. 8). Figure 1 shows that the conformation of phosphoramidite (1c) is different between RhCl(C1-D)(1c) and RhCl(C2’-D)(1c). In the case of the Rh-catalyzed Michael addition of α-cyano carboxylate, the active intermediate has been reported to be the N-bonded enolate complex of α-cyanocarboxylate.9a,c The enantioface of the N-bonded enolate complex is reversed (entries 4 vs. 5 and 7 vs. 8) by changing the chiral dienes from RhCl(C1-D)(1c) to RhCl(C2’-D)(1c) (Figure 2). Both the N-bonded enolate complexes are attacked by an electrophile from the opposite side of the phosphoramidite (1c); Therefore, the Rh complex with C1-symmetric chiral diene C1-D gives (S)-enriched products and the Rh complex with pseudo C2-symmetric chiral diene C2’-D affords (R)-enriched products.
CONCLUSION
In the Rh complexes, the monodentate diphenylmethane-derived phosphoramidite can be controlled by a chiral diene to possess a single chiral conformation. The complex with the chirally controlled phosphoramidite could be used in the asymmetric Michael additions of α-cyanocarboxylates. Both the chiral diene and achiral diphenylmethane-derived phosphoramidite cooperated to give higher yields and enantiomeric excesses.
EXPERIMENTAL
1H NMR, 13C NMR, and 31P NMR spectra were measured on Bruker AV300 (300 MHz) spectrometer. Capillary gas chromatographic analysis (GC) was conducted on Shimadzu GC-14B instrument equipped with FID detector and capillary column coated with PEG-20 M by using N2 as a carrier gas. Peak area was calculated by Shimadzu C-R6A as an automatic integrator. CP-Chirasil-Dex CB (i.d. 0.25 mm x 25 m, CHROMPACK; GL Science) was used as chiral column. Optical rotations were measured on a JASCO DIP-370. TOF Mass spectra were measured on a JEOL JMS-T100LC. Computational calculations were executed on Sun Fire X4600 (Tokyo Institute of Technology).
All experiments were carried out under an argon atmosphere otherwise noted. Analytical thin layer chromatography (TLC) was performed on a glass plates pre-coated with silica-gel (Merck Kieselgal 60 F254, layer thickness 0.25 mm). Visualization was accomplished by UV light (254 nm), anisaldehyde, KMnO4. Column chromatography was performed on KANTO Silica Gel 60N (spherical, neutral) or ICN Alumina N (neutral, Activity Super I). Dichloromethane (dehydrate) and toluene (dehydrate) were purchased from Kanto Chemical Co., Inc.
1,5-Dibromocycloocta-1,5-diene11 and 2-cyanopropionic acid tert-butyl ester (2b)12 were prepared by the reported method. 2,3-Dibromobicyclo[2.2.1]hepta-2,5-diene (Br-nbd),13 (2S,8R)-2-(3,5-dimethylphenyl)- 8-methoxy-1,8-dimethylbicyclo[2.2.2]octa-2,5-diene (C1-D),5 (1S,4S,8S)-5-benzyl-2-phenyl-8-methoxy- 1,8-dimethyl-bicyclo[2.2.2]octa-2,5-diene (C2’-D)5 and [RhCl(C1-D)]26 were also prepared by the reported method.
(2,10-Dimethyl-12H-5,7-dioxa-6-phosphadibenzo[a,d]cycloocten-6-yl)dibenzylamine (1a)
To a solution of 2,2’-methylenebis(4-methylphenol) (45.7 mg, 0.2 mmol) in toluene (2 mL) was added HMPT (hexamethylphosphoric triamide) (45 μL, 0.24 mmol) at rt under an argon atmosphere. After stirred for 2 h at 100 °C, toluene and excess HMPT were evaporated under reduced pressure. The residue and 1H-tetrazole (21.1 mg, 0.3 mmol) were dissolved in toluene. To the mixture was added dibenzylamine (38 µL, 0.2 mmol) at rt. After stirred for 24 h at 100 °C, the reaction mixture was quenched with H2O three times. The organic layer was dried over K2CO3. After concentration under reduced pressure, the residue was purified by alumina column chromatography (hexane/CH2Cl2 = 10/1) to give phosphoramidite 1a (68.9 mg, 76% yield).
1H NMR (CDCl3, 300 MHz) δ 2.32 (s, 6H), 3.54 (d, 1H, J = 12.9 Hz), 4.33 (d, 4H, J = 10.2 Hz), 4.35 (dd, 1H, J = 12.6, 3.0 Hz), 6.99 (br, 4H), 7.16 (s, 2H), 7.29-7.46 (m, 10H).
13C NMR (CDCl3, 75 MHz) δ 22.78, 34.13, 47.86, 48.14, 122.51, 127.11, 128.40, 128.56, 128.63, 130.40, 133.93, 134.71, 134.74, 138.38, 148.82, 148.88.
31P NMR (CDCl3, 121 MHz) δ 138.18.
TOF-HRMS (ESI), Calcd for C29H29NO2P [M+H]+: 454.1936, Found: 454.1946.
(4,8-Dimethyl-12H-5,7-dioxa-6-phosphadibenzo[a,d]cycloocten-6-yl)dimethylamine (1b)
To a solution of 2,2’-methylenebis(6-methylphenol) (45.7 mg, 0.2 mmol) in toluene (2 mL) was added HMPT (45 μL, 0.24 mmol) at rt under an argon atmosphere. After stirred for 2 h at 100 °C, toluene and excess HMPT were evaporated under reduced pressure. The residue was purified by alumina column chromatography (hexane/CH2Cl2 = 10/1) to give phosphoramidite 1b (36.8 mg, 61% yield).
1H NMR (CDCl3, 300 MHz) δ 2.27 (s, 6H), 2.97 (d, 6H, J = 10.8 Hz), 3.48 (d, 1H, J = 12.3 Hz), 4.46 (dd, 1H, J = 12.3, 3.0 Hz), 6.93 (t, 2H, J = 7.5 Hz), 7.04 (d, 2H, J = 7.5 Hz), 7.19 (d, 2H, J = 7.5 Hz).
13C NMR (CDCl3, 75 MHz) δ 16.95, 16.99, 33.99, 34.53, 35.16, 35.40, 124.10, 124.12, 127.42, 127.44, 129.01, 131.10, 131.14, 135.71, 135.75, 149.34, 149.40.
31P NMR (CDCl3, 121 MHz) δ 138.72.
TOF-HRMS (ESI), Calcd for C17H21NO2P [M+H]+: 302.1310, Found: 302.1298.
(4,8-Di-tert-butyl-2,10-dimethyl-12H-5,7-dioxa-6-phosphadibenzo[a,d]cycloocten-6-yl)dimethylamine (1c)
Phosphoramidite 1c was prepared from 2,2’-methylenebis(6-tert-butyl-4-methylphenol) in a similar manner to phosphoramidite 1b (90% yield).
1H NMR (CDCl3, 300 MHz) δ 1.40 (s, 18H), 2.30 (s, 6H), 2.96 (d, 6H, J = 9.3 Hz), 3.33 (d, 1H, J = 12.3 Hz), 4.35 (dd, 1H, J = 12.3, 3.0 Hz), 7.02 (d, 2H, J = 2.1 Hz), 7.11 (d, 2H, J = 2.1 Hz).
13C NMR (CDCl3, 75 MHz) δ 21.05, 30.75, 30.81, 34.73, 35.60, 35.86, 126.39, 128.56, 132.73, 136.16, 136.20, 141.61, 141.66, 148.30, 148.40.
31P NMR (CDCl3, 121 MHz) δ 144.28.
TOF-HRMS (ESI), Calcd for C25H37NO2P [M+H]+: 414.2562, Found: 414.2561.
(4,8-Di-tert-butyl-2,10-dimethyl-12H-5,7-dioxa-6-phosphadibenzo[a,d]cycloocten-6-yl)dibenzylamine (1d)
Phosphoramidite 1d was prepared from 2,2’-methylenebis(6-tert-butyl-4-methylphenol) and dibenzylamine in a manner similar to phosphoramidite 1a (88% yield).
1H NMR (CDCl3, 300 MHz) δ 1.58 (s, 18H), 2.44 (s, 6H), 3.52 (d, 1H, J = 12.6 Hz), 4.53 (d, 4H, J = 7.2 Hz), 4.64 (dd, 1H, J = 12.6, 2.7 Hz), 7.19 (d, 2H, J = 2.1 Hz), 7.28 (d, 2H, J = 2.1 Hz), 7.38-7.56 (m, 10H).
13C NMR (CDCl3, 75 MHz) δ 21.18, 31.40, 31.47, 34.90, 35.11, 48.53, 48.79, 126.72, 127.19, 128.23, 128.59, 129.79, 133.18, 136.75, 136.78, 137.75, 137.82, 141.72, 141.77, 147.56, 147.64.
31P NMR (CDCl3, 121 MHz) δ 137.53.
TOF-HRMS (ESI), Calcd for C37H45NO2P [M+H]+: 566.3194, Found: 566.3188.
(S,S)-N-(2,10-Dimethyl-12H-5,7-dioxa-6-phosphadibenzo[a,d]cycloocten-6-yl)bis(1-phenylethyl)amine (1e)
To a solution of 2,2’-methylenebis(4-methylphenol) (45.7 mg, 0.2 mmol) in toluene (2 mL) was added HMPT (hexamethylphosphoric triamide) (45 μL, 0.24 mmol) at rt under an argon atmosphere. After stirred for 2 h at 100 °C, toluene and excess HMPT were evaporated under reduced pressure. The residue and 1H-tetrazole (21.1 mg, 0.3 mmol) were dissolved in toluene. To the mixture was added bis[(S)-1-phenylethyl]amine (45 µL, 0.2 mmol) at rt. After stirred for 24 h at 100 °C, the reaction mixture was quenched with H2O three times. The organic layer was dried over K2CO3. After concentration under reduced pressure, the residue was purified by alumina column chromatography (hexane/CH2Cl2 = 10/1) to give phosphoramidite 1e (38.4 mg, 40% yield).
1H NMR (CDCl3, 300 MHz) δ 1.87 (d, 6H, J = 7.2 Hz), 2.32 (s, 3H), 2.33 (s, 3H), 3.48 (d, 1H, J = 12.9 Hz), 4.42 (dd, 1H, J = 12.9, 3.0 Hz), 4.94 (dq, 2H, J = 11.7, 7.2 Hz), 6.64 (d, 1H, J = 8.1 Hz), 6.93 (d, 1H, J = 8.1 Hz), 6.99 (s, 2H), 7.16-7.30 (m, 12H).
13C NMR (CDCl3, 75 MHz) δ 20.80, 22.08, 22.21, 34.08, 53.00, 53.16, 122.57, 122.61, 122.73, 122.78, 126.58, 127.80, 128.03, 128.07, 128.49, 130.22, 130.38, 133.75, 135.13, 143.55, 143.57, 149.45, 149.54, 149.71, 149.80.
31P NMR (CDCl3, 121 MHz) δ 142.20.
TOF-HRMS (ESI), Calcd for C31H32NO2PNa [M+Na]+: 504.2068, Found: 504.2060.
[α]D27 -66 (c 0.50 in CHCl3).
[RhCl(C2’-D)]2
A mixture of (1S,4S,8S)-5-benzyl-2-phenyl-8-methoxy-1,8-dimethylbicyclo[2.2.2]octa-2,5-diene (chiral diene C2’-D) (33.0 mg, 0.1 mmol) and [RhCl(ethylene)2] (21.4 mg, 0.055 mmol) in benzene (4.0 mL) was stirred under an argon atmosphere at rt for 24 h, and then the reaction mixture was filtered through Celite. After the filtrate was evaporated under reduced pressure, the yellow residue was washed with Et2O. Prolonged evacuation of the product at 50 °C gave [RhCl(C2’-D)]2 (90% yield). The product was diastereomeric mixture.
1H NMR (300 MHz, CDCl3) major diastereomer δ 0.89 (d, J = 13.8 Hz, 1H), 1.09 (d, J = 13.8 Hz, 1H), 1.14 (s, 3H), 1.78 (s, 3H), 2.91 (d, J = 15.9 Hz, 1H), 3.00 (d, J = 15.9 Hz, 1H), 3.07 (s, 3H), 3.30 (s, 1H), 3.40-3.42 (m, 1H), 4.06 (d, J = 5.4 Hz, 1H), 7.22-7.39 (m, 6H), 7.95-8.00 (m, 4H).
1H NMR (300 MHz, CDCl3) minor diastereomer δ 0.85 (d, J = 14.1 Hz, 1H), 1.03 (d, J = 14.1 Hz, 1H), 1.14 (s, 3H), 1.63 (s, 3H), 3.05 (s, 3H), 3.21-3.30 (m, 3H), 3.36 (s, 1H), 4.12 (d, J = 6.3 Hz, 1H), 7.22-7.39 (m, 4H), 7.52-7.47 (m, 2H), 7.79-7.81 (m, 2H), 7.95-8.00 (m, 2H).
13C NMR (75 MHz, CDCl3) major diastereomer δ 21.80, 41.21, 46.22 (d, JRh-C = 10.9 Hz), 47.68, 49.56, 49.76 (d, JRh-C = 3.6 Hz), 53.13 (d, JRh-C = 2.9 Hz), 55.85 (d, JRh-C = 10.8 Hz), 70.84 (d, JRh-C = 12.1 Hz), 71.46 (d, JRh-C = 11.2 Hz), 77.24, 81.10, 126.22, 127.12, 128.15, 130.41, 130.85, 131.12, 137.66, 138.39.
13C NMR (75 MHz, CDCl3) minor diastereomer δ 21.90, 41.31, 44.90 (d, JRh-C = 11.6 Hz), 47.58, 49.46, 54.12, 55.01 (d, JRh-C = 10.1 Hz), 71.74 (d, JRh-C = 11.2 Hz), 81.03, 120.33, 127.20, 130.92, 137.72, 138.49.
Anal. Calcd for C48H52Cl2O2Rh2·2H2O: C, 57.10; H, 5.99. Found: C, 57.10; H, 5.99. H2O was derived from ether that washed the yellow residue (1H NMR: 1.52 (s, 4H)).
[α]D27 -76 (c 1.2 in CHCl3).
[RhCl(Br-nbd)]2
[RhCl(Br-nbd)]2 was prepared from 2,3-dibromobicyclo[2.2.1]hepta-2,5-diene (Br-nbd) and [RhCl(ethylene)2] in a similar to manner as [RhCl(C2’-D)]2 (40% yield).
1H NMR (CDCl3, 300 MHz) δ 1.20-1.27 (m, 4H), 1.58 (br, 2H), 1.76 (d, 2H, J = 9.6 Hz), 4.32 (br, 2H), 4.57 (br, 2H).
1,5-Dimethylcycloocta-1,5-diene (DM-cod)
To a suspension of 1,5-dibromocycloocta-1,5-diene (280 mg, 1.05 mmol) and NiCl2(dppp) (23 mg, 0.04 mmol) in dry Et2O (20 mL) was added methyl Grignard reagent (1.59 mmol, 3.0 M in Et2O) under an argon atmosphere at 0 °C. The reaction mixture was stirred for 30 min at 0 °C, for 1 h at rt and for 2 h at reflux. H2O (20 mL) was added and the reaction mixture was extracted with Et2O. Combined organic layer was dried over MgSO4 and solvent was removed under reduced pressure. Crude product was purified by flash chromatography over silica-gel eluted with pentane to give the product (yield 90%).
1H NMR (300 MHz, CDCl3) δ 0.95 (d, J = 6.9 Hz, 12 H), 2.29-2.38 (m, 10 H), 5.32 (t, J = 6.0 Hz, 2H).
13C NMR (75 MHz, CDCl3) δ 26.3, 33.3, 122.5, 135.8.
[RhCl(dm-cod)]2
[RhCl(dm-cod)]2 was prepared from 1,5-dimethylcycloocta-1,5-diene (DM-cod) and [RhCl(ethylene)2] in a similar to manner as [RhCl(C2’-D)]2 (30% yield). The product was diastereomeric mixture.
1H NMR (300 MHz, CDCl3) δ 1.50 (s, 6H, dia. minor), 1.65-1.85 (m, 8H), 1.72 (s, 6H, dia. major), 2.05-2.23 (m, 4H), 2.47-2.64 (m, 4H), 3.79 (d, 2H, J = 7.2 Hz, dia. minor), 3.99 (d, 2H, J = 6.9 Hz, dia. major).
RhCl(C1-D)(1c)
To a mixture of (4,8-di-tert-butyl-2,10-dimethyl-12H-5,7-dioxa-6-phosphadibenzo[a,d]cycloocten-6- yl)dimethylamine (1c) (8.3 mg, 0.02 mmol) and [RhCl(C1-D)]2 (8.0 mg, 0.01 mmol) was added CH2Cl2 (1 mL) at rt under an argon atmosphere. After stirred for 1 h, the reaction mixture was concentrated under reduced pressure to give RhCl(C1-D)(1c) complex (16.1 mg, 98% yield).
1H NMR (CDCl3, 300 MHz) δ 0.90 (s, 1H), 0.94 (s, 1H), 1.15 (s, 3H), 1.31 (s, 9H), 1.34 (s, 9H), 1.39 (s, 3H), 2.26 (s, 6H), 2.29 (s, 3H), 2.31 (s, 3H), 2.72 (br, 1H), 3.22 (d, 6H, J = 9.0 Hz), 3.27 (s, 3H), 3.57 (br, 1H), 3.90 (d, 1H, J = 15.6 Hz), 4.18 (t, 1H, J = 6.0 Hz), 5.00 (t, 1H, J = 4.8 Hz), 6.83 (s, 1H), 6.98 (br, 2H), 7.01 (br, 2H), 7.07 (br, 2H).
31P NMR (CDCl3, 121 MHz) δ 119.5 (d, JP-Rh = 268.6 Hz).
[α]D27 -65 (c 0.20 in CHCl3).
m/z (ESI): Calcd for Rh(C1-D)(1c) [M-Cl]+: 784.3, 785.3, 786.3. Found: 784.3, 785.3, 786.3.
RhCl(nbd)(1e)
To a mixture of (S,S)-N-(2,10-Dimethyl-12H-5,7-dioxa-6-phosphadibenzo[a,d]cycloocten-6-yl)bis(1-phenylethyl)amine (1e) (9.6 mg, 0.02 mmol) and [RhCl(nbd)]2 (4.6 mg, 0.01 mmol) was added CH2Cl2 (1 mL) at rt under an argon atmosphere. After stirred for 1 h, the reaction mixture was concentrated under reduced pressure to give RhCl(nbd)(1e) complex (11.8 mg, 98% yield).
1H NMR (CDCl3, 300 MHz) δ 1.22 (s, 2H), 1.73 (d, 6H, J = 7.2 Hz), 2.31 (s, 3H), 2.32 (s, 3H), 2.86 (br, 1H), 3.23 (br, 1H), 3.46 (br, 2H), 3.79 (d, 1H, J = 13.8 Hz), 4.58 (d, 1H, J = 13.8 Hz), 5.13 (br, 1H), 5.22 (br, 1H), 5.34 (dq, 2H, J = 14.1, 7.2 Hz), 6.81 (d, 1H, J = 8.1 Hz), 6.90 (d, 1H, J = 8.1 Hz), 7.01 (dd, 1H, J = 8.1, 2.1 Hz), 7.08 (d, 1H, J = 2.1 Hz), 7.10 (d, 1H, J = 2.1 Hz), 7.21 (d, 1H, J = 8.1 Hz), 7.30-7.40 (m, 6H), 7.51 (d, 4H, J = 7.5 Hz).
31P NMR (CDCl3, 121 MHz) δ 125.0 (d, JP-Rh = 266.0 Hz).
[α]D27 -2.4 (c 0.25 in CHCl3).
m/z (ESI): Calcd for Rh(nbd)(1e) [M-Cl]+: 676.2, 677.2, 678.2, 679.2, 680.2. Found: 676.2, 677.2, 678.2, 679.2, 680.2.
Typical procedure for Rh-catalyzed Michael addition of α-cyanocarboxylate
To a mixture of [RhCl(C1-D)]2 (0.8 mg, 0.001 mmol) and (4,8-di-tert-butyl-2,10-dimethyl-12H-5,7- dioxa-6-phosphadibenzo[a,d]cycloocten-6-yl)dimethylamine 1c (0.8 mg, 0.002 mmol) was added CH2Cl2 (2.0 mL) under an argon atmosphere. After stirred for 30 min at rt, to the solution was added 2-cyanoproionic acid tert-butyl ester (2b) (15.5 mg, 0.1 mmol). After cooled down to -78 °C, to the reaction mixture were added acrolein (35 µL, 0.5 mmol) and iPr2NEt (1.7 µL, 0.01 mmol). After stirred for 3 h at -78 °C, the reaction mixture was evaporated under reduced pressure. The residue was purified by silica-gel chromatography (hexane/EtOAc = 3/2) to give 2-cyano-2-methyl-5-oxopentanoate acid tert-butyl ester (3b) (20.0 mg, 95% yield).
2-Cyano-2-methyl-5-oxopentanoate acid ethyl ester (3a)9c
1H NMR (CDCl3, 300 MHz) δ 1.28 (t, J = 7.2 Hz, 3H), 1.57 (s, 3H), 2.05 (ddd, J = 14.4, 10.2, 5.7 Hz, 1H), 2.22 (ddd, J = 14.4, 9.9, 6.0 Hz, 1H), 2.59 (ddd, J = 18.6, 10.2, 6.0 Hz, 1H), 2.69 (ddd, J = 18.6, 9.9, 5.7 Hz, 1H), 4.21 (q, J = 7.2 Hz, 2H), 9.73 (s, 1H).
13C NMR (CDCl3, 121 MHz) δ 13.98, 23.51, 30.02, 39.76, 43.03, 63.09, 119.37, 186.71, 199.12.
[α]D27 +1.3 (c 1.0 in CHCl3) for a sample that is 48% ee (R).
GC (column, CP-Chirasil-Dex CB, i.d. 0.25 mm x 25 m, Chrompack; carrier gas, N2 (75 kPa); column temp, 110 ºC; injection and detection temp, 140 ºC; split rate, 100:1), tR = 21.2 min (S)/23.9 min (R).
2-Cyano-2-methyl-5-oxopentanoate acid tert-butyl ester (3b)9c
1H NMR (CDCl3, 300 MHz) δ 1.52 (s, 9H), 1.60 (s, 3H), 2.10 (ddd, J = 14.4, 10.2, 5.4 Hz, 1H), 2.23 (ddd, 1H, J = 14.4, 10.5, 5.1 Hz), 2.64 (dddd, 1H, J = 18.3, 10.2, 5.4, 0.9 Hz), 2.77 (dddd, 1H, J = 18.3, 10.5, 5.1, 0.9 Hz), 9.82 (s, 1H).
13C NMR (CDCl3, 121 MHz) δ 23.51, 27.75, 29.96, 39.84, 43.77, 84.37, 119.68, 167.67, 199.26.
[α]D27 -2.4 (c 0.90 in CHCl3) for a sample that is 86% ee (S).
GC (column, CP-Chirasil-Dex CB, i.d. 0.25 mm x 25 m, Chrompack; carrier gas, N2 (75 kPa); column temp, 115 ºC; injection and detection temp, 150 ºC; split rate, 100:1), tR = 15.7 min (S)/17.1 min (R).
Computational Methods
All the calculations were performed with GAUSSIAN 03 program package. All the structures were optimized at B3LYP/631SDD (SDD for Rh, 6-31G(d) for others) level. The optimized geometries were verified as an equilibrium structures having no imaginary frequency.
RhCl(C1-D’)(1c’)
Charge = 0, Multiplicity = 1
SCF Done: E(B3LYP/631SDD) = -2358.51183292 a.u.
§In honor of Professor Emeritus Keiichiro Fukumoto on 75th birthday
References
1. The word atropos consists of “a” meaning “not” and “tropos” meaning “turn” in Greek. Therefore, the chirally rigid or flexible nature of a ligand can be called atropos or tropos, respectively. K. Mikami, K. Aikawa, Y. Yusa, J. J. Jodry, and M. Yamanaka, Synlett, 2002, 1561; CrossRef Also see: W. Kuhn, ‘Stereochemie’, ed. by K. Freudenberg, Franz Deuticke, Leipzig, 1933, pp. 803-824.
2. a) ‘Catalytic Asymmetric Synthesis’, Vol. I and II, ed. by I. Ojima, VCH, New York, 1993, 2000; b) H. Brunner and W. Zettlmeier, ‘Handbook of Enantioselective Catalysis’, VCH, Weinheim, 1993; c) R. Noyori, ‘Asymmetric Catalysis in Organic Synthesis’, Wiley, New York, 1994; d) ‘Transition Metals for Organic Synthesis’, ed. by M. Beller and C. Bolm, VCH: Weinheim, 1998; e) ‘Comprehensive Asymmetric Catalysis’, Vol. 1-3, ed. by E. N. Jacobsen, A. Pfaltz, and H. Yamamoto, Springer, Berlin, 1999; f) ‘New Frontiers in Asymmetric Catalysis’, ed. by K. Mikami and M. Lautens, Wiley, New York, 2007; g) Review on Asymmetric Activation, K. Mikami, M. Terada, T. Korenaga, Y. Matsumoto, M. Ueki, and R. Angelaud, Angew. Chem. Int. Ed., 2000, 39, 3532. CrossRef
3. a) L. Horner, H. Siegel, and H. Büthe, Angew. Chem., 1968, 80, 1034; CrossRef b) W. S. Knowles and M. J. Sabacky, Chem. Commun., 1968, 1445; CrossRef c) C. Claver, E. Fernandez, A. Gillon, K. Heslop, D. J. Hyett, A. Martorell, A. G. Orpen, and P. G. Pringle, Chem. Commun., 2000, 961; CrossRef d) M. T. Reetz and G. Mehler, Angew. Chem. Int. Ed., 2000, 39, 3889; CrossRef e) M. T. Reetz and T. Sell, Tetrahedron Lett., 2000, 41, 6333; CrossRef f) M. van den Berg, A. J. Minnaard, E. P. Schudde, J. van Esch, A. H. M. de Vries, J. G. de Vries, and B. L. Feringa, J. Am. Chem. Soc., 2000, 122, 11539; CrossRef g) D. Pena, A. J. Minnaard, A. H. M. de Varis, J. G. de Vries, and B. L. Feringa, Org. Lett., 2003, 5, 475; CrossRef h) C. Monti, C. Gennari, and U. Piarulli, Chem. Eur. J., 2007, 13, 1547. CrossRef
4. a) K. Mikami, K. Wakabayashi, and K. Aikawa, Org. Lett., 2006, 8, 1517; CrossRef b) K. Mikami, K. Wakabayashi, Y. Yusa, and K. Aikawa, Chem. Commun., 2006, 2365; CrossRef c) Q. Jing, C. A. Sandoval, Z. Wang, and K. Ding, Eur. J. Org. Chem., 2006, 3606; CrossRef d) K. Wakabayashi, K. Aikawa, and K. Mikami, J. Am. Chem. Soc., 2008, 130, 5012. CrossRef
5. a) T. Hayashi, K. Ueyama, U. Norihito, and K. Yoshida, J. Am. Chem. Soc., 2003, 125, 11508; CrossRef b) C. Fischer, C. Defieber, T. Suzuki, and E. M. Carreira, J. Am. Chem. Soc., 2004, 126, 1628; CrossRef c) C. Defieber, J.-F. Paquin, S. Serna, and E. M. Carreira, Org. Lett., 2004, 6, 3873; CrossRef d) N. Yokunaga, Y. Otomaru, K. Okamoto, K. Ueyama, R. Shintani, and T. Hayashi, J. Am. Chem. Soc., 2004, 126, 13584. CrossRef
6. Asymmetric catalysts are generally prepared from chiral ligands and central metals. The asymmetric catalysts thus prepared can be evolved into more activated catalysts with higher catalytic activity and enantioselectivity by chiral activators (“Asymmetric Activation”, See ref. 2f,g). However, the additional ligation does not necessarily lead to higher catalytic activity. We have thus proposed the term “asymmetric synergy (effect)” leading to the higher enantioselectivity without increase in the catalytic activity (even with decrease). For the asymmetric synergy (effect), see: K. Aikawa, S. Akutagawa, and K. Mikami, J. Am. Chem. Soc., 2006, 128, 12648. CrossRef
7. All the calculations were performed with GAUSSIAN 03 program package. All the structures were optimized at B3LYP/631SDD (SDD for Rh, 6-31G(d) for others) level. The optimized geometries were verified as equilibrium structures having no imaginary frequency.
8. F. Giacomia, A. Meersma, L. Panella, L. Lefort, A. H. M. de Varis, and J. G. de Varis, Angew. Chem. Int. Ed., 2007, 46, 1497. CrossRef
9. a) M. Sawamura, Y. Hamashima, and Y. Ito, J. Am. Chem. Soc., 1992, 114, 8295; CrossRef b) K. Inagaki, K. Nozaki, and H. Takaya, Synlett, 1997, 119; CrossRef c) Y. Motoyama, Y. Koga, K. Kobayashi, K. Aoki, and H. Nishiyama, Chem. Eur. J., 2002, 8, 2968. CrossRef
10. For deprotonation of cyanocarboxylate, a catalytic amount of iPr2NEt was needed in this Michael addition (see Table 1: entry 2).
11. H. Detert, B. Rose, W. Mayer, and H. Meier, Chem. Ber., 1994, 127, 1529. CrossRef
12. X. Wang, M. Kitamura, and K. Maruoka, J. Am. Chem. Soc., 2007, 129, 1038. CrossRef
13. P. Peluso, C. Greco, O. De Lucchi, and S. Cossu, Eur. J. Org. Chem., 2002, 4024 CrossRef