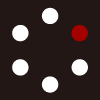
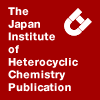
HETEROCYCLES
An International Journal for Reviews and Communications in Heterocyclic ChemistryWeb Edition ISSN: 1881-0942
Published online by The Japan Institute of Heterocyclic Chemistry
e-Journal
Full Text HTML
Received, 11th August, 2008, Accepted, 10th October, 2008, Published online, 14th October, 2008.
DOI: 10.3987/COM-08-S(F)106
■ Synthesis and Electrochromic Properties of Bis(2-tetrathiafulvalenylethynylphenyl)ethynes
Masashi Hasegawa, Yusuke Kobayashi, Kenji Hara, Hideo Enozawa, and Masahiko Iyoda*
Graduate School of Science, Tokyo Metropolitan University, 1-1, Minami-ohsawa, Hachioji, Tokyo 192-0364, Japan
Abstract
Bis(2-tetrathiafulvalenylethynylphenyl)ethynes 1a and 1b have been synthesized by the Sonogashira coupling reaction of 4-iodotetrathiafulvalenes with bis(2-ethynylphenyl)ethyne. The dimeric TTFs 1a and 1b form an open-chain anti conformation in the neutral and tetracation states, whereas 1a and 1b form a helical syn conformation in the mono- and dication states owing to their face–to–face interaction between the two TTF units. Such conformational changes lead to unique electrochromic and on–off switching properties of 1a and 1b in UV–vis–NIR spectra.INTRODUCTION
Large π–conjugated systems with multi–redox sites show various electronic structures that lead to important electrochromic, magnetic, and electroconducting properties.1,2 Dimeric and oligomeric tetrathiafulvalenes (TTFs) have been utilized widely as an electroactive unit for designing functional materials in supramolecular chemistry, materials science, and nanoscience.2-4 Among these oligomers, face–to–face stacked TTFs show various interactions in the neutral and oxidation states, forming weakly interacting neutral (TTF)2, strongly dimerized mixed–valence (TTF)2•+, and π–dimer (TTF•+)2.5,6 Previously, we reported dimeric TTFs and higher oligomers that show a conformational change between nonhelical and helical structures owing to the strong interaction of TTF units upon oxidation.7 With this in mind, we designed a new class of dimeric TTFs, namely, 1a and 1b, connected to a phenylene ethynylene scaffold. The dimeric TTFs 1a and 1b may show clear electrochromic properties owing to a dynamic conformational change between their open–chain “anti” and helical “syn” structures depending on the strength of the interaction of their terminal TTF moieties (Figure 1). We report here the unique electrochromic and on–off switching properties of 1a and 1b observed in UV–vis–NIR spectra.
RESULTS AND DISCUSSION
The synthetic route to 1a and 1b is depicted in Scheme 1. The treatment of the alkylthio–substituted TTF derivatives 2a and 2b with C4H9nLi at -90 °C, followed by the addition of C6F13nI gave 4-iodo-4’,5’-bis(alkylthio)tetrathiafulvalenes 3a and 3b in good yields. The Sonogashira coupling reaction of 3a and 3b with bis(2-ethynylphenyl)ethyne afforded 1a and 1b in 73 % and 45 % yields, respectively.8,9 The dimeric TTFs 1a and 1b are air–stable red crystalline compounds. The chemical shift of the TTF protons of both 1a and 1b was similar to those of ethynyl TTF derivatives reported previously.4d,10 Since the VT–NMR experiments on 1a and 1b in CD2Cl2 did not show any spectral change until -90 °C, the predominant conformation of 1a and 1b would be the anti form in the neutral state (Figure 1).
As shown in Figure 2 and Table 1, the deconvoluted cyclic voltammogram of 1a in CH2Cl2 revealed two one–electron oxidations at 0.03 and 0.05 V (vs. Fc/Fc+) and one two–electron oxidation at 0.38 V, corresponding to the formation of 1a•+, 1a2+, and 1a4+, respectively. Similarly, the deconvoluted cyclic voltammogram of 1b showed sequential oxidation to produce 1b•+, 1b2+, and 1b4+. Since bis(tetrathiafulvalenyl)ethyne and 1,4-bis(tetrathiafulvalenyl)benzene showed only two redox potentials corresponding to the formation of bis(cation–radical) and bis(dication), the observation of the cation radical 1•+ suggests that a face-to-face stacking interaction at the terminal two TTF units produces a mixed-valence (MV) state.11
The dimeric TTFs 1a and 1b showed unique electrochromic properties depending on their oxidation state. The chemical oxidation of 1a or 1b with n equiv. of Fe(ClO4)3 in CH2Cl2–MeCN (v/v = 4:1) afforded the corresponding cationic species 1an+ and 1bn+ (n = 1, 2, and 4), respectively. The solution color of 1a upon oxidation changed markedly. Thus, the neutral 1a was yellow, the cation radical 1a•+ was orange, the dication 1a2+ was green, and the tetracation 1a4+ was blue. The oxidation of 1b exhibited similar electrochromism.
The UV–vis–NIR data of the cation radicals, dications, and tetracations derived from 1a and 1b are summarized in Table 2. Both the electronic spectra of the cation radicals derived from 1a and 1b are very similar. The electronic spectra of the cation radicals prepared from 1a or 1b with 1 equiv. of Fe(ClO4)3 revealed a very broad absorption in the NIR region (λmax = ca. 2000 nm) in addition to the absorption at 750 nm (Figure 3). On the basis of results of previous studies, the absorption is assigned to the CT absorption due to the MV state of stacked (TTF)2•+ units.6a Since the spectra were measured under very dilute conditions (10-5 M), intermolecular contacts of the TTF•+ can be ruled out.12 Thus, both 1a and 1b adopt a helical syn structure in the cation radical state. For the dications 1a2+ and 1b2+, the absorption in the NIR region disappeared and the electronic spectra showed a large split in the TTF•+ transition into blue-shifted absorption and shoulder CT absorption (Figure 3). The pronounced blue shift of the absorptions at 420 and 727 nm is Davydov’s blue shift due to the formation of the π-dimer of TTF•+.11,13,14 Therefore, the dications 1a2+ and 1b2+ also adopt a helical syn structure.15 However, because of the strong electrostatic repulsion, the electronic spectra of 1a4+ and 1b4+ showed no stacked absorption.
In summary, we report here the synthesis of bis(2-tetrathiafulvalenylethynylphenyl)ethynes 1a and 1b by the Sonogashira coupling reaction and their electronic properties. The dimeric TTFs 1a and 1b showed marked elecrochromism depending on the oxidation state, i.e., yellow for 1, orange for 1•+, green for 12+, and blue for 14+. The remarkable color change is due to the formation of helical structures in the cation–radical and dication states. Furthermore, the cation radicals 1a•+ and 1b•+ showed very wide and intense absorptions in the NIR–IR region, and hence may be a new class of NIR–IR photofilters controlled by redox potentials.
ACKNOWLEDGEMENTS
This work was partially supported by a grant-in aid for Scientific Research from the Ministry of Education, Cultre, Sports, Science and Technology of Japan. The authors are greatly indebted to Dr. Yoshiyuki Kuwatani (VSN, Inc.), Dr. Yoshihiro Miyake (The University of Tokyo), and Dr. Tohru Nishinaga (Tokyo Metropolitan University) for helpful discussions.
This paper is dedicated to Professor Emeritus Keiichiro Fukumoto on the occasion of his 75th birthday.
References
1. (a) Y. Shirota, J. Mater. Chem., 2000, 10, 1; CrossRef (b) N. Robertson and C. A. McGowan, Chem. Soc. Rev., 2003, 32, 96; CrossRef (c) J. Roncali, Macromol. Rapid Commun., 2007, 28, 1761; CrossRef (d) G. C. Bazan, J. Org. Chem., 2007, 72, 8615; CrossRef (e) K. Müllen and J. P. Rabe, Acc. Chem. Res., 2008, 41, 511. CrossRef
2. (a) M. B. Nielsen, C. Lomhold, and J. Becher, Chem. Soc. Rev., 2000, 29, 153; CrossRef (b) M. R. Bryce, J. Mater. Chem., 2000, 10, 589; CrossRef (c) J. L. Segura and N. Martín, Angew. Chem. Int. Ed., 2001, 40, 1372; CrossRef (d) M. Iyoda in ‘TTF Chemistry,’ ed. by J. Yamada and T. Sugimoto, Kodansha-Springer, Tokyo (2004), Chap. 8, p. 177; (e) M. Iyoda, M. Hasegawa and M. Miyake, Chem. Rev., 2004, 104, 5085. CrossRef
3. M. Iyoda, M. Hasegawa and H. Enozawa, Chem. Lett., 2007, 36, 1402. CrossRef
4. (a) M. Iyoda, M. Hasegawa, K. Hara, J. Takano, E. Ogura, and Y. Kuwatani, J. Phys. IV France, 2004, 114, 455; CrossRef (b) M. Hasegawa, Y. Kuwatani, and M. Iyoda, J. Phys. IV France, 2004, 114, 505; CrossRef (c) H. Enozawa, M. Hasegawa, D. Takamatsu, K. Fukui, and M. Iyoda, Org. Lett., 2006, 8, 1917; CrossRef (d) M. Hasegawa, H. Enozawa, Y. Kawabata, and M. Iyoda, J. Am. Chem. Soc., 2007, 129, 3072. CrossRef
5. (a) S. V. Roskha and J. K. Kochi, J. Am. Chem. Soc., 2007, 129, 828; CrossRef (b) L. Khdorkovsky, L. Shapiro, Krief, A. Shames, G. Mabon, A. Gorgues, and M. Griffard, Chem. Commun., 2001, 273.
6. (a) M. Iyoda, M. Hasegawa, Y. Kuwatani, H. Nishikawa, K. Fukami, S. Nagase, and G. Yamamoto, Chem. Lett., 2001, 30, 1146; CrossRef (b) M. Hasegawa, J. Takano, H. Enozawa, Y. Kuwatani, and M. Iyoda, Tetrahedron Lett., 2004, 45, 4109; CrossRef (c) J. Lyskawa, M. Sallé, J.-Y. Balandier, F. Le Derf, E. Levillain, M. Allain, P. Viel, and S. Palacin, Chem. Commun., 2006, 2233. CrossRef
7. (a) M. Iyoda, K. Hara, Y. Kuwatani, and S. Nagase, Org. Lett., 2000, 2, 2217; CrossRef (b) U. Kux and M. Iyoda, Chem. Lett., 1994, 2327. CrossRef
8. Data for 1a: red needles, mp 102.5-104 °C; LDI-MS m/z 982 (M+); 1H NMR (600 MHz, CDCl3) δ 7.65 (dd, J = 7.6 and 1.1 Hz, 2H), 7.49 (d, J = 7.6 Hz, 2H), 7.36 (td, J = 7.7 Hz and 1.2 Hz, 2H), 7.32 (td, J = 7.7 and 1.2 Hz, 2H), 6.55 (s, 2H), 2.83-2.80 (m, 8H), 1.65-1.61 (m, 8H), 1.48-1.41 (m, 8H), 0.94-0.92 (m, 12H); 13C NMR (100 MHz, CDCl3) δ 132.3 (2C, CH), 131.6 (2C, CH), 128.7 (2C), 128.3 (2C, CH), 127.9 (2C), 127.6 (2C), 125.6 (2C), 124.8 (2C), 123.5 (2C), 115.9 (2C), 112.0 (2C), 109.1 (2C), 92.5 (2C, C≡C), 92.1 (2C, C≡C), 84.6 (2C, C≡C), 35.99 (2C, CH2), 35.96 (2C, CH2), 31.73 (2C, CH2), 31.71 (2C, CH2), 21.63 (2C, CH2), 21.61 (2C, CH2), 13.6 (4C, CH3).
9. Data for 1b: red fine crystals, mp 85-86.5 °C; LDI-MS m/z 1430 (M+); 1H NMR (600 MHz, CDCl3) δ 7.65 (dd, J = 7.7 and 1.2 Hz, 2H), 7.49 (dd, J = 7.7 and 1.2 Hz, 2H), 7.37 (td, J = 7.7 and 1.2 Hz, 2H), 7.32 (td, J =7.7 and 1.2 Hz, 2H), 6.57 (s, 2H), 2.83-2.80 (m, 8H), 1.65-1.61 (m, 8H), 1.43-1.38 (m, 8H), 1.32-1.22 (m, 64 H), 0.89-0.86 (m, 12 H); 13C NMR (150 MHz, CDCl3) δ 132.38 (2C, CH), 131.71 (2C, CH), 128.73 (2C), 128.34 (2C, CH), 128.01 (2C), 127.76 (2C), 125.70 (2C), 124.81 (2C, CH), 124.55 (2C), 116.03 (2C), 112.57 (2C), 109.21 (2C), 92.54 (2C, C≡C), 92.19 (2C, C≡C), 84.63 (2C, C≡C), 36.40 (2C, CH2), 36.37 (2C, CH2), 31.95 (4C, CH2), 29.79 (2C, CH2), 29.78 (2C, CH2), 29.70 (4C, CH2), 29.68 (4C, CH2), 39.64 (4C, CH2), 29.57(2C, CH2), 29.56 (2C, CH2), 29.38 (4C, CH2), 29.18 (2C, CH2), 29.16 (2C, CH2), 28.59 (2C, CH2), 28.57 (2C, CH2), 22.71 (4C, CH2), 14.13 (4C, CH3).
10. T. Otsubo, Y. Kochi, A. Bitoh, and F. Ogura, Chem. Lett., 1994, 2047. CrossRef
11. (a) M. Iyoda, M. Hasegawa, J. Takano, K. Hara, and Y. Kuwatani, Chem. Lett., 2002, 590; CrossRef (b) M. Hasegawa, Dissertation (2004).
12. On the basis of our previous studies of the self-association of TTF oligomers, it was found that a very strong association (K2 > 100,000) is necessary in a dilute solution. See refs. 4c and 4d.
13. J. B. Torrance, B. A. Scott, B. Welber, F. B. Kaufman, and P. E. Seiden, Phys. Rev. B, 1979, 19, 730. CrossRef
14. Other examples of the π-dimer: (a) K. Nakao, T. Nishiuchi, and M. Iyoda, Heterocycles, 2008, 76, 727; CrossRef (b) T. Sakai, T. Satou, T. Kaikawa, K. Takimiya, T. Otsubo, and Y. Aso, J. Am. Chem. Soc. 2005, 127, 8082.. CrossRef
15. The intensity of the ESR signals of 1a2+ in CH2Cl2-MeCN solution decreased at low temperatures. The solution at -70 °C contains only 10 % unpaired spin compared with the solution at 20 °C