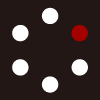
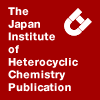
HETEROCYCLES
An International Journal for Reviews and Communications in Heterocyclic ChemistryWeb Edition ISSN: 1881-0942
Published online by The Japan Institute of Heterocyclic Chemistry
e-Journal
Full Text HTML
Received, 14th August, 2008, Accepted, 29th September, 2008, Published online, 6th October, 2008.
DOI: 10.3987/COM-08-11523
■ NMR Studies of 2-Aryl Derivatives of Benzimidazole, Benzimidazolium Ion, and Benzimidazoline
Chang Kiu Lee and In-Sook Han Lee*
Department of Chemistry, Kangwon National University, Chuncheon 200-701, S. Korea
Abstract
A series of 2-arylbenzimidazoles, 2-aryl-1,3-dimethylbenzimidazolium iodides, and 2-aryl-1,3-dimethylbenzimidazolines were prepared and their NMR spectra were examined. The substituent parameters were calculated for 2-benzimidazolyl, 2-benzimidazoliumyl, and 2-benzimidazolinyl groups on the chemical shifts of the protons and the carbons of benzene ring. The 2-benzimidazoliumyl group was found to cause a significant up-field shift of the ipso-carbon signal and down-field shift for the ortho- and para-carbon signals. On the other hand, the 2-benzimidazolyl and the 2-benzimidazolinyl groups cause down-field shift of the ipso-carbon signals but cause almost negligible change on the other carbon signals.INTRODUCTION
Benzimidazole has been one of the subjects that are widely investigated. Countless reports on physical properties, biological activities, reactions, and synthesis of benzimidazole and its derivatives can be found in literature.1
We have been interested in 2-arylbenzimidazolium ions and 2-arylbenzimidazolines because they are the mimics of NAD+ and NADH, respectively.2 In the course of our extensive investigation on the hydride transfer reaction between the benzimidazolium ions and the benzimidazolines, we came to observe striking phenomena in their NMR chemical shifts. This paper is to report the effects of benzimidazole, benzimidazolium ion, and benzimidazoline rings as substituents on the 1H and 13C NMR chemical shifts of benzene.
RESULTS AND DISCUSSION
The synthesis of 2-arylbenzimidazoles (1) have been investigated for more than a century. New and modified methods have been reported frequently in recent years.3 Our preparation of 1 was based on the method of Craig et al. with a slight modification.4 The synthesis of 1,3-dimethyl-2- arylbenzimidazolium ions (2), and 1,3-dimethyl-2-arylbenzimidazolines (3) are summarized in Scheme 1.
Several years ago we reported the prohibited tautomerism in unsubstituted 2-phenylbenzimidazole (1i).5 Now we have found that the tautomerism of the proton at N-1 to N-3 does not take place in all the m- and p-substituted 2-phenylbenzimidazoles (1a-h) in DMSO-d6 solution. This is evidenced by the appearance of seven 13C NMR signals for the benzimidzole skeleton. It is known that benzimidazole itself tautomerizes in solution showing only four 13C NMR signals.6
The NMR spectra of 1, 2, and 3 have been obtained in 0.1 M concentration at 25 ºC. The assignments of the 1H and 13C NMR signals were made by 1H-1H COSY and 1H-13C HETCOR spectroscopy. Meta- and para-fluorophenyl derivatives (1-3a and 1-3e) were investigated using the 19F-13C coupling constants for definite assignments. The observed coupling constants (i.e., for 1a: 1J = 244.8, 2J = 20.5 and 24.3, 3J = 8.6 and 9.2, 4J = 2.7 Hz; for 1e: 1J = 250.2, 2J = 22.2, 3J = 9.3, 4J = 4.0 Hz) are very close to the values for fluorobenzene.7
Once the accurate chemical shift assignments are made for 1a-i, the effect of 2-benzimidazolyl group on the chemical shift of the ring-protons and the ring-carbons in benzene can be calculated by following the additivity rule and using the values listed in literature.8 Similarly, the effects of 2-benzimidazoliumyl and 2-benzimidazolinyl groups were also calculated, and the results are listed in Table 1.
One of the striking observations is the shielding effect of 1,3-dimethylbenzimidazolium-2-yl group on the ipso-C, causing an up-field shift of 6.1 ppm. Although the positive charge may be delocalized mostly on
the two N atoms through resonance, the imidazole ring is positively charged. The positively charged
group is expected to deshield a neighboring nucleus and a down-field shift should be the result. For example, the (CH3)3N+− group causes a down-field shift of the ipso-C signal by 19.5 ppm.9 Substituents such as vinyl, phenyl, and carbonyl that have an sp2-hybridized carbon atom usually cause a down-field shift of the ipso-C signal of the benzene ring.9
There are many examples of substituents that cause a down-field shift of the ipso-C and a simultaneous up-field shift of ortho-C signals (i. e., F−). On the other hand, less than ten examples of substituents that cause the opposite effect have been reported, and they are HC≡C−, N≡N+−, Br−, I−, CN−, NCS−, CF3CO−, NC−, and H3Si− groups.9 Among these only HC≡C−, Br−, and CF3CO− show comparable magnitudes of shift of the ipso-C signal to the benzimidazolium-2-yl group, as shown in Table 1. But, meta- and para-Cs do not show similar trends in either magnitude or direction.
The up-field shift of the ipso-C signal by Br is explained by heavy atom shielding while the similar phenomenon by HC≡C− or N≡C− is believed to be the result of the anisotropy effect of triple bond.10 The only example of the up-field shift of the ipso-C signal by a substituent having an sp2-hybridized carbon is the trifluoroacetyl group. It is conceivable that the strong electron-withdrawing property of the trifluoromethyl group may induce the lone-pair electrons on the O atom to move toward the C atom so that the C=O double bond becomes close to a triple bond (C≡O). This should enhance the s character of the carbonyl carbon (i.e., from sp2 to sp), which, in turn, generates the anisotropy effect as if it is an alkynyl carbon.
The magnitudes of the up-field shift of ipso-C signal caused by N≡C− (− 15.7 ppm) and N≡N+− (− 12.7 ppm) are much greater than for the other substituents except the I− group.9 This can also be explained by the increasing anisotropy effect due to the movement of the lone-pair electron on N atom toward the C or N atom, which should make the s character of the these atoms far greater than a normal sp-hybridized C of alkynyl group.
In order to examine the effect of the methyl groups in 2 on the chemical shift, we prepared 2-phenylbenzimidazolium chloride (4), obtained its NMR spectra under the same conditions, and compared the spectra with those of 2i. To our surprise, the chemical shift value of C-2 of 4 (150.31 ppm) was very close to that of 2i (150.76 ppm). But the ipso-C signal is shifted to down-field by 3.50 ppm. The signal which corresponds to C-3a/7a is shifted most down-field by 3.57 ppm and the other 13C NMR signals are all shifted to same direction by 1.25-2.30 ppm. The 1H NMR spectrum of 4 also shows an interesting deviation. The signal corresponding to H-4/7 is shifted to down-field by 0.13 ppm, but other signals are all shifted to up-field by 0.37-0.44 ppm. These findings are consistent with the predominant paramagnetic shielding term that may be the major factor affecting the 13C NMR chemical shift.
Then, a question may arise: why the ipso-Cs of the phenyl groups in 2 show an up-field shift with significant magnitude (− 6.1 ppm) whereas those of 1 show a down-field shift with a relatively small magnitude (+ 3.3 ppm)? The benzimidazole and phenyl rings in 1 may lie nearly in the same plane because there is no significant steric hindrance between the two rings. The electronic effect of the benzimidazole ring is both electron-withdrawing by the inductive effect and electron-releasing by the mesomeric effect. The two effects may offset one another, if not completely, and the result is small deviation in the 13C NMR chemical shift values of the phenyl rings (− 0.8 for o-C, 0.05 for m- and p-C).
In case of the benzimidazolines (3) the two rings are not coplanar. The effect of the benzimidazolin-2-yl group may mostly originate from the sp3-hybridized carbon atom bearing two electronegative N atoms. Therefore, the phenyl C signals are shifted to down-field and only the ipso-C signal is shifted the most (+ 12.8 ppm).
The signals corresponding to the ortho- and para-C of 2 are shifted to down-field significantly with similar magnitude (4.4 and 4.2 ppm, respectively) compared to those of 1 and 3. This may be the results of the so called π polarization which may be initiated by the positively charged benzimidazolium substituent.11
The effect of the benzimidazol-2-yl, benzimidazolium-2-yl, and benzimidazolin-2-yl groups on the proton chemical shift of the benzene ring is also interesting. It is worthy to point out that the ortho-H in 1 is shifted to down-field by 1.00 ppm. Such a large shift cannot be found in literature to the best of our knowledge. The nitro (-NO2) group has been known to cause the most down-field shift of the ortho-H by 0.95 ppm. Phenyloxycarbonyl (-COOC6H5) group is reported to be the second most effective substituent that causes down-field shift of the ortho-H signal by 0.90 ppm.12 Chlorocarbonyl (ClCO−) and chlorosulfonyl (ClO2S−) groups have comparable effects on ortho-H as the benzimidazolium-2-yl group has, but their effects on meta- and para-H are quite different as shown in Table 1. The large down-field shift of the ortho-H signal of 1 may be due to the diamagnetic anisotropy effect originated by the π electrons in the benzimidazole ring. However, the hydrogen bonding between N and ortho-H atoms should also contribute to the unusual magnitude of the shift.
In general, the electronic effect of a substituent decreases as the distance from it increases, as appeared in meta- and para-H signals of 1. But the proton signals of 3 show quite a large irregularity (o-H 0.11, m-H − 0.10, p-H 0.26 ppm). All the proton signals of 2 show quite a down-field shift (o-H 0.78, m-H 0.64, p-H 0.73 ppm). Apparently, the electronic effect of the positively charged ring is propagated to the phenyl ring not only by through-bond transmission but also by through-space transmission. The two rings may stack on each other, as shown for I. Similar stacking should not be possible with the benzimidazoline (3) because C-2 is sp3-hybridized. The meta-H of the phenyl ring may lie on the shielding region of the ring current effect produced by the benzene ring, as shown like II.
In conclusion, we report the benzimidazolium-2-yl group as a positively charged substituent that cause the up-field shift of the ipso-C signal of benzene ring. The benzimimidazol-2-yl group causes a down-field shift of the ortho-H signal of the benzene ring.
EXPERIMENTAL
Nuclear magnetic resonance (NMR) spectra were recorded on a Bruker DPX-400 FT NMR spectrometer in the Central Lab of Kangwon National University at 400 MHz for 1H and 100 MHz for 13C and were referenced to tetramethylsilane (TMS). The concentration of the solution was 0.10 M in DMSO-d6. Each solution was prepared in a 1 mL cylindrical volumetrical flask by weighing the compound into the flask and filling with solvent containing 1%-TMS. A portion (0.6 mL) of the solution was transferred into an NMR tube and the spectrum was obtained at 20 oC, and the results are listed in Tables 2-7.
The benzimidazole derivatives 1, 2, and 3 are all known compounds.13
An Illustrative Procedure for the Preparation of 2-Arylbenzimidazoles: A mixture of o-phenylenediamine (2.06 g, 19.1 mmol), benzoic acid (2.30 g, 20.5 mmol), and polyphosphoric acid (5.52 g) was stirred in an oil bath at 180 ºC for 1.5 h. The solution was cooled to rt. The pH was adjusted to 10 with 6%-NH4OH. The tarry solid was collected by filtration, washed with NH4OH solution, and recrystallized from EtOH to give 1i.
An Illustrative Procedure for the Preparation of 2-Aryl-1,3-dimethylbenzimidazolium Iodides: A solution of sodium hydroxide (0.28 g, 7 mmol) in MeOH (7 mL) was prepared first in a pressure tube. 1i (1.27 g, 6.54 mmol) and methyl iodide (6.75 g, 47 mmol) were added. The tube was sealed and was heated in an oil bath at 110 ºC for 12 h. After cooling to rt, the mixture was suspended in acetone and filtered. The residue was dissolved in EtOH (200 mL) by heating, decolorized with charcoal, and recrystallized from EtOH to give 2i.
An Illustrative Procedure for the Preparation of 2-Aryl-1,3-dimethylbenzimidazolium Iodides: NaBH4 (0.35 g, 9.25 mmol) was added to a solution of 2i (1.60 g, 4.57 mmol) in MeOH (30 mL). The mixture was stirred. Reaction took place immediately. After stirring for 30 min, the solvent was evaporated off and the residue was extracted with CH2Cl2. The extract was evaporated and the residue was recrystallized from EtOH -water (5:1 v/v) to give 3i.
ACKNOWLEDGEMENTS
We thank Dr. Gary Kwong of the 3M Co. for help in preparing the manuscript. This research was supported by the Korea Research Foundation -C000207(I01794).
References
1. M. R. Grimmett, in ‘Comprehensive Heterocyclic Chemistry,’ 2nd Ed., Pergamon, New York, 1996, pp. 77-220.
2. I.-S. H. Lee, H. J. Kil, and Y. R. Ji, J. Phys. Org. Chem., 2007, 20, 484. CrossRef
3. G. Navarrete-Vazquez, H. Moreno-Diaz, S. Estrada-Soto, M. Torres-Piedra, I. Leon-Rivera, H. Tlahuext, O. Munoz-Muniz, and H. Torres-Gomez, Synth. Commun., 2007, 37, 2815; CrossRef S. Kaul, A. Kumar, B. Sain, and A. K. Bhatnagar, Synth. Commun., 2007, 37, 2457; CrossRef H. Ma, X. Han, Y. Wang, and J. Wang, Heterocycles, 2007, 71, 1821; CrossRef B. Y. Giri, B. L. A. P. Devi, K. N. Gangadhar, K. V. Lakshmi, R. B. N. Prasad, N. Lingaiah, and P. S. S. Prasad, Synth. Commun., 2007, 37, 2331; CrossRef H. Prokopcova, and C. O. Kappe, J. Org. Chem., 2007, 72, 4440; CrossRef J. W. Hubbard, A. M. Piegols, and B. C. G. Soederberg, Tetrahedron, 2007, 63, 7077; CrossRef M. P. Surpur, P. R. Singh, S. B. Patil, and S. D. Samant, Synth. Commun., 2007, 37, 1375. CrossRef
4. J. C. Craig, N. N. Ekwuribe, C. C. Fu, and K. A. M. Walker, Synthesis, 1981, 303. CrossRef
5. I.-S. H. Lee, E. H. Jeoung, and C. K. Lee, J. Hetereocycl. Chem., 1996, 33, 1711. CrossRef
6. E. P. Papadopoulos and U. Hollstein, Org. Magn. Reson., 1982, 19, 188. CrossRef
7. E. Breitmaier and W. Voelter, ‘Carbon-13 NMR Spectroscopy,’ 3rd Ed., VCH, Weinheim, Germany, 1987, p. 269.
8. D. L. Pavia, G. M. Lampman, and G. S. Kriz, ‘Introduction to Spectroscopy,’ 3rd Ed., Harcourt, Inc., Orlando, FL, 2001, pp. A29-A30.
9. E. Pretsch, T. Clerc, J. Seibl, and W. Simon, ‘Tables of Spectral Data for Structure Determination of Organic Compounds,’ 2nd Ed., 1989, pp. C120-C125.
10. Reference 7, p. 255.
11. R. T. C. Brownlee and D. J. Craik, J. Chem. Soc., Perkin Trans. 2, 1981, 760. CrossRef
12. Reference 9, p. H260.
13. I.-S. H. Lee, E. H. Jeoung, and M. M. Kreevoy, J. Am. Chem. Soc., 1997, 119, 2722; CrossRef X.-Q. Zhu, M.-T. Zhang, A. Yu, C.-H. Wang, and J.-P. Cheng, J. Am. Chem. Soc., 2008, 130, 2501. CrossRef