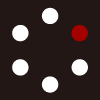
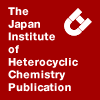
HETEROCYCLES
An International Journal for Reviews and Communications in Heterocyclic ChemistryWeb Edition ISSN: 1881-0942
Published online by The Japan Institute of Heterocyclic Chemistry
e-Journal
Full Text HTML
Received, 19th August, 2008, Accepted, 18th September, 2008, Published online, 22nd September, 2008.
DOI: 10.3987/COM-08-11529
■ Synthesis of Related Substances of Cilostazol
Jin Zheng, Zheng Liu, Yiru Dai, Qingjie Zhao, and Jingshan Shen*
Chinese Academy of Sciences, Shanghai Institute of Materia Medica, 555 Zuchongzhi Rd., Zhangjiang Hi-Tech Park, Shanghai, 201203, China
Abstract
The impurities in API of cilostazol were detected by LC/MS during the process development. The structures of two impurities 6 and 7 and the related formation mechanisms were proposed. Synthesis of 6 and 7 was conducted for confirmation of the speculated structures.INTRODUCTION
As a potent and reversible phosphodiesterase III inhibitor, 6-[4-(1-cyclohexyl-1H-tetrazol-5-yl)butoxy]-
3,4-dihydro-2(1H)-quinolinone (1, cilostazol), is used in clinic for the treatment of intermittent claudication and the control of its impurities is important to clinical safety.1 The two key steps for the preparation of cilostazol 1 (Scheme 1) involved Friedel-Crafts intramolecular cyclization2 of 3-chloro-N-(4-methoxyphenyl)propanamide 2 to give the corresponding 3, 4-dihydrocarbostyril 3, which was then condensed with 5-(4-chlorobutyl)-1-cyclohexyl-1H-tetrazole 4 in the presence of potassium hydroxide in DMF to give cilostazol 1.3 Besides known impurities, we detected two unreported impurities in the final crude product during our process development of cilostazol 1 (Scheme 1).
RESULTS AND DISCUSSION
Four major impurities detected by LC-MS were shown in Table 1. By indication of molecular ion peak and possible formation mechanisms, structures of unknown impurities were suggested. Compound 55 (M+161) was a known impurity of cilostazol, resulting from the trace amount of 5-(4,4-dichlorobutyl)-1- cyclohexyl-1H-tetrazole in the starting material 4. The unknown impurity 6 (M-2), derived from 9, was identical to 6-[4-(1-cyclohexyl-1H-tetrazol-5-yl)butoxy]-1H-quinolin-2-one (OPC-13015, the known active metabolite of cilostazol). The impurity 7 (M+220) was formed by the reaction of compound 11 with two equivalents of 4. Another known impurity 8 (M+206) was formed due to double linking of compound 3 with two equivalents of 4 in the alkaline condition.
In the Friedel-Crafts reaction of preparing 3, the possible coordination of the carbonyl with AlCl3 led to the conversion of the amide group on 3, from an electron donating substituent into an electron withdrawing group, and reduced the activity of the aromatic ring.4 Excess of AlCl3 (3 equivalents) and high temperature (150 °C) were required to improve the yield of 3.2
While it remains unclear how 9 was formed during the Friedel-Crafts reaction. Two pathways can be postulated as the formation mechanisms of 9. Dehydrogenation of 3,4-dicarbostyril was well-documented in the literatures.7 Lewis acids such as AlCl3 and SbCl5 sometimes were used as the powerful dehydrogenating agents.8-10 One possible mechanism was AlCl3-mediated dehydrogenation of 3 (Scheme 2). The other possible mechanism was plausible via the auto-oxidation of 3 at high temperature due to the presence of adventitious O2 or other oxidants (Scheme 3).
The LC-MS analysis showed that no N-Oxide existed in the starting material of 4-methyoxyaniline. The literatures11 indicated that direct oxidation of 2-quinolinone by m-CPBA at room temperature led to 1% hydroxamic acid derivative. We postulated that N-O bond of 11 was formed by auto-oxidation of 9. Coordination of the amide carbonyl with Lewis acid leads to a polarized complex 10. The polarization facilitated the oxidation on N atom of quinolinol segment, by radical oxygen, to hydroxamic acid 11 (Scheme 4).
Based on the above speculation, compounds 6 and 7 were synthesized. Compound 6 was conveniently obtained by reaction of 6-hydroxy-1H-quinolin-2-one 912 with 4 (Scheme 5).
Attempts13 to synthesis of hydroxamic acid structure 11 were achieved by oxidation of quinoline, with H2O2 in acetic acid, to its N-oxide and further oxidation of the resulted N-oxide with lead tetraacetate (Scheme 6). Though the oxidation of 12 with H2O214 in acetic acid led to 6-hydroxyl-1-oxyquinoline in good yield, the following oxidation of the resulted oxyquinoline 14 (R=H) with lead tetraacetate 15 did not give satisfactory result according to the literature procedure, due to the presence of the unprotected hydroxyl group on 14 (R=H) . We then modified the strategy, and firstly protected the hydroxyl group on 6-quinolinol by using 4. The resulted 6-[4-(1-cyclohexyl-1H-tetrazol-5-yl)butoxy]quinoline 13 was oxidized with m-CPBA16 in chloroform at 0 °C to give 6-[4-(1-cyclohexyl-1H-tetrazol-5-yl)butoxy]-1- oxyquinoline 14 in good yield. 6-[4-(1-Cyclohexyl-1H-tetrazol-5-yl)butoxy]-1-hydroxyquinolin-2-one 15 was subsequently obtained by oxidation of the oxy-quinoline derivative 14 with lead tetraacetate15 in presence of CaCO3 in refluxing benzene. Following the procedure similar to preparation of 6, the target compound 7 was obtained in 75% yield by the treatment of 15 with 4 in the presence of potassium carbonate.
Finally, the LC-MS analysis showed that compound 6 and 7 respectively had the same molecular ion peak and relative retention time as the unknown byproducts. Thus, the two unknown byproducts were identical with the speculated structures.
EXPERIMENTAL
Melting points were determined on a WRR melting point apparatus and are uncorrected. NMR spectra were determined using a Mercury 300 MHz, FT NMR spectrometer. Elemental analyses were performed on Elementar Vario EL. Reaction solvents were purchased without further purification. LC-MS analysis was carried out with Perkin-Elmer triple quadrupole mass spectrometer coupled with Agilent 1100 with VWD UV-vis detector. The ion spray voltage of LC-MS spectrum was set at 4.5 kv and temperature was kept at 500 °C and spectra data were acquired from m/z 60 to 800. A YMC C8, 250 mm×4.6 mm 5 μm particle diameter column, and water-acetonitril (60:40, v: v) and acetonitril as gradient eluents were used for the separation analysis. Detection was carried out at 254 nm and flow rate was maintained at 1.0 mL/min.
6-[4-(1-Cyclohexyl-1H-tetrazol-5-yl)butoxy]-1H-quinolin-2-one (6)
A mixture of 6-hydroxy-1H-quinolin-2-one 9 (207 mmol, 33 g), 5-(4-chlorobutyl)-1-cyclohexyl-1H- tetrazole 4 (228 mmol, 55 g) and potassium carbonate (414 mmol, 57 g) in DMF (300 mL) was stirred at 80 °C under nitrogen for 7 h. The reaction mixture was cooled to 25 °C and diluted with water (1.5 L), resulting in the formation of a thick brown precipitate. The precipitate was isolated by filtration, washed with H2O (3×100 mL), and recrystallized from EtOAc to give 6 (64 g, 85%) as off-white solid, mp 181-183 °C (lit.,17 177.5-178.5 °C). 1H NMR (DMSO-d6): δ 1.14-2.02 (m, 14H), 2.98 (t, 2H, J = 6.3 Hz), 4.04 (t, 2H, J = 6.0 Hz), 4.40 (m, 1H), 6.48 (d, 1H, J = 9.8 Hz), 7.10-7.27 (m, 3H), 7.82 (d, 1H, J = 9.4 Hz), 11.63 (s, 1H). 13C NMR (CDCl3): δ 22.93, 23.82, 24.70, 25.22, 28.36, 32.81, 57.53, 67.56, 109.37, 114.71, 119.52, 120.60, 121.17, 131.49, 136.90, 154.61, 156.94. ESI m/z 368.2 (M+ + H). Anal. Calcd for C20H25N5O2: C, 65.37; H, 6.86; N, 19.06. Found: C, 65.29; H, 6.99; N, 18.82.
6-[4-(1-Cyclohexyl-1H-tetrazol-5-yl)butoxy]quinoline (13)
13 was obtained in 80% yield as a white solid by the same procedure as described for 6, mp 106-108 °C. 1H NMR (DMSO-d6): δ 1.20-2.16 (m, 14H), 2.95 (t, 2H, J = 7.4 Hz), 4.14 (t, 2H, J = 6.0 Hz), 4.05-4.20 (m, 1H), 7.06 (d, 1H, J = 2.9 Hz), 7.33 (dd, 1H, J1 = 6.3 Hz, J 2 = 2.9 Hz), 7.36 (dd, 1H, J1 = 6.3 Hz, J2 = 4.1 Hz), 8.01 (d, 1H, J = 9.3 Hz), 8.05 (dd, 1H, J1 = 8.4 Hz, J2 = 1.5 Hz), 8.76 (dd, 1H, J1 = 4.1 Hz, J2 = 1.5 Hz). 13C NMR (CDCl3): δ 22.89, 23.86, 24.65, 25.16, 28.27, 32.77, 57.46, 67.24, 105.73, 121.31, 122.22, 129.17, 130.73, 134.71, 144.22, 147.87, 153.40, 156.71. ESI m/z 352.2 (M+ + H). Anal. Calcd for C20H25N5O: C, 68.35; H, 7.17; N, 19.93. Found: C, 68.33; H, 7.18; N, 20.22.
6-[4-(1-Cyclohexyl-1H-tetrazol-5-yl)butoxy]-1-oxyquinoline (14)
To a stirred solution of 13 (35.0 g, 0.1 mol) in CHCl3 (80 mL) was added dropwise a solution of m-CPBA
(17.3 g, 0.1 mol) in CHCl3 (40 mL) at 0 °C for 1.5 h. After further stirred for 4 h, the reaction mixture was washed sequently with 10% aqueous sodium sulfite, saturated aqueous sodium bicarbonate solution and water. After removal of the chloroform in vacuo, the crude product was crystallized from EtOAc to give the pure product 14 (238 g, 65%) as a white solid, mp 175-176 °C. 1H NMR δ 1.28-2.15 (m, 14H), 2.94 (t, 2H, J = 7.4 Hz), 4.14 (t, 2H, J = 5.8 Hz), 4.04-4.20 (m, 1H), 7.10 (d, 1H, J = 2.6 Hz), 7.25 (dd, 1H, J1 = 8.6 Hz, J2 = 6.3 Hz), 7.34 (dd, 1H, J1 = 9.3 Hz, J1 = 2.6 Hz), 7.62 (d, 1H, J = 8.6 Hz), 8.38 (d, 1H, J = 6.3 Hz), 8.65 (d, 1H, J = 9.3 Hz). 13C NMR (DMSO-d6): δ 21.80, 23.17, 24.59, 27.79, 32.45, 56.06, 67.61, 107.32, 120.61, 122.34, 122.38, 124.06, 131.86, 133.29, 136.38, 154.08, 158.06. ESI m/z 368.3 (M+ + H). Anal. Calcd for C20H25N5O2: C, 65.37; H, 6.86; N, 19.06. Found: C, 65.37; H, 6.89; N, 19.10.
6-[4-(1-Cyclohexyl-1H-tetrazol-5-yl)butoxy]-1-hydroxyquinolin-2-one (15)
Lead tetraacetate (15.0 g, wet with acetic acid) and calcium carbonate (0.8 g) were added portionwise to a solution of quinoline N-oxide 14 (5.5 g, 1.7 mmol) in benzene (150 mL) at 0 °C for 10 min. The mixture was heated at reflux for 6 h. After cooling, the reaction mixture was filtered to remove inorganic salts. The filtrate was concentrated in vacuo to dryness. 3% Dilute HCl (50 mL) was added to the residue, and the mixture heated to 80 °C for 2 h, resulting in the formation of a brown solid. After filtration, the crude product was recrystallized from EtOAc/CHCl3 to yield 15 (4.1 g, 72%) as a white solid, mp 135-137 °C. 1H NMR (CDCl3): δ 1.22-2.15 (m, 14H), 2.94 (t, 2H, J = 7.5 Hz), 4.08 (t, 2H, J = 6.0 Hz), 4.13 (m, 1H), 6.84 (d, 1H, J = 9.3 Hz), 7.04 (d, 1H, J = 2.7 Hz), 7.34 (dd, 1H, J1 = 9.3 Hz, J2 = 2.7 Hz), 7.68 (d, 1H, J = 9.6 Hz), 7.76 (d, 1H, J = 9.6 Hz). 13C NMR (CDCl3): δ 22.93, 23.82, 24.70, 25.22, 28.36, 32.81, 57.53, 67.56, 109.37, 114.71, 119.52, 120.60, 121.17, 131.49, 136.90, 154.61, 156.94. ESI m/z 384.3 (M+ + H). Anal. Calcd for C20H25N5O3: C, 62.65; H, 6.57; N, 18.26. Found: C, 62.62; H, 6.61; N, 18.31.
1,6-Bis[4-(1-cyclohexyl-1H-tetrazol-5-yl)butoxy]-1H-quinolin-2-one (7)
A mixture of 6-[4-(1-cyclohexyl-1H-tetrazol-5-yl)butoxy]-1-hydroxyquinolin-2-one 15 (4 g 10.4 mmol), 5-(4-chlorobutyl)-1-cyclohexyl- 1H-tetrazole 4 (2.8 g, 11.5 mmol) and potassium carbonate (2.9 g, 20.8 mmol) in DMF (25 mL) was stirred at 80 °C under nitrogen for 7 h. The reaction mixture was cooled to 25 °C and diluted with water (200 mL), resulting in the formation of a brown precipitate. The precipitate was isolated by filtration, washed with H2O (3×50 mL), and recrystallized from EtOAc to give 7 (4.6 g, 75%) as a white solid, mp 98-99 °C. 1H NMR (CDCl3): δ 1.20-2.23 (m, 28H), 2.93 (t, 2H, J = 7.5 Hz), 3.11 (t, 2H, J = 7.5 Hz), 4.06 (t, 2H, J = 6.0 Hz), 4.13 (m, 1H), 4.29 (t, 2H, J = 6.0 Hz), 4.34 (m, 1H), 6.73 (d, 1H, J = 9.3 Hz), 7.01 (d, 1H, J = 2.7 Hz), 7.19 (dd, 1H, J1 = 9.3 Hz, J2 = 2.7 Hz), 7.47 (d, 1H, J = 9.6 Hz), 7.60 (d, 1H, J = 9.6 Hz). 13C NMR (DMSO-d6): δ 21.67, 21.83, 24.59, 26.69, 27.94, 32.46, 32.51, 55.98, 56.06, 67.48, 74.03, 111.15, 112.91, 120.39, 122.66, 132.57, 138.23, 154.09, 156.32. ESI m/z
590.3 (M+ + H). Anal. Calcd for C31H43N9O3: C, 63.14; H, 7.35; N, 21.38. Found: C, 63.30; H, 7.33; N,
21.61.
References
1. D. Jacoby and E. R. Mohler III, Drugs, 2004, 64, 1657. CrossRef
2. M. R. Bell, U.S. Patent 3,819,637, 1974.
3. T. Nishi and K. Nakagawa, U.S. Patent 4,277,479, 1981.
4. V. Naddaka, G. Davidi, S. Saeed, O. Arad, and J. Kaspi, U.S. Patent 2005/0222202 A1.
5. A. S. Jadhav, D. B. Pathare, and M. S. Shingare, Drug Development and Industrial Pharmacy, 2007, 33, 173. CrossRef
6. M. Mendelovici, G. Pilarsky, T. Nidam, and B. Z. Dolitzky, U.S. Patent, 2002/0317936 A1.
7. T. Nishi, K. Yamamoto, T. Shimizu, T. Kanbe, Y. Kimura, and K. Nakagawa, Chem. Pharm. Bull., 1983, 31, 798. CrossRef
8. E. D. Barnett, J. W. Cook, and I. G. Nixon, J. Chem. Soc., 1927, 504. CrossRef
9. J. Holmes and R. Pettit, J. Org. Chem., 1963, 28, 1695. CrossRef
10. P. P. Fu and R. G. Harvey, Chem. Rev., 1978, 78, 317. CrossRef
11. W. A. Lott and E. Shaw, J. Am. Chem. Soc., 1949, 71, 70. CrossRef
12. A. Y. Soliman, S. E. Nagdy, H. M. Bakeer, M. M. Moustafa, and R. M. Saleh, Indian J. Chem., Sect. B, 1990, 29, 239.
13. M. Raban, V. A. Martin, and L. Craine, J. Org. Chem., 1990, 55, 4311. CrossRef
14. E. Ochiai, J. Org. Chem., 1953, 18, 534. CrossRef
15. E. Ochiai and A. Ohta, Chem. Pharm. Bull., 1962, 10, 1260. CrossRef
16. J. C. Craig and K. K. Purushothaman, J. Org. Chem., 1970, 35, 1721. CrossRef
17. T. Nishi, F. Tabusa, T. Tanaka, T. Shimizu, and T. Kanbe, Chem. Pharm. Bull., 1983, 31, 1151 CrossRef