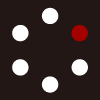
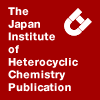
HETEROCYCLES
An International Journal for Reviews and Communications in Heterocyclic ChemistryWeb Edition ISSN: 1881-0942
Published online by The Japan Institute of Heterocyclic Chemistry
e-Journal
Full Text HTML
Received, 19th August, 2008, Accepted, 19th September, 2008, Published online, 25th September, 2008.
DOI: 10.3987/COM-08-11530
■ Synthesis of Cyclopenteno[c] Fused 2,2’-Bipyridine Based Cyclophane by Ring-Closing Metathesis (RCM)
Justyna Lawecka, Danuta Branowska, Zbigniew Karczmarzyk, and Andrzej Rykowski*
Department of Chemistry University of Podlasie, 08-110 Siedlce, Poland
Abstract
A route to cyclophane 7 incorporating mixed 2,2’-bipyridine 3, consisting of the two different heterocyclic units, i.e. uncondensed pyridine and cyclopenta[c]pyridine, using as a crucial step ring-closing metathesis is reported.2,2′-Bipyridines (bpy) and their fused analogues are utilized as molecular hosts in bpy based macrocycles, in particular crown macrocycles.2 Such chelating ligands possessing bpy subunit encapsulate specific metal ions3 or various organic and inorganic substrates4 and have been successfully applied as building blocks for larger supramolecular species.5 Recently, Kwong and coworkers reported a series of C2 – symmetric bpy crown ethers.6 These macrocycles exhibited enantioselective binding towards the amino acid derivatives and chiral organic ammonium salts. The similar interactions of bpy thiacrown ethers with neutral and cationic substrates have never been investigated. In view of these interesting results and our continuing interest in the synthesis of bpy thiacrown ethers,7 we began exploring the possibilities of preparation of the monoannulated bpy cyclophane 7 (Scheme 1), consisting of the two different heterocyclic units: uncondensed pyridine and cyclopenta[c]pyridine. Attachment of the cyclopentene into one of the pyridine rings in 7 can result in a higher efficiency of a ligand field, since the basicity of this ring nitrogen is enhanced.8
In an early report we have demonstrated the use of easily available 3,3′-bis(methylsulfanyl)-5,5′-bi-1,2,4-triazine 1 as a convenient precursor for the preparation of monoannulated bpy derivative 3.9 This approach is based on the regioselective intermolecular Diels-Alder/retro Diels-Alder reaction of 1 with 1-vinylimidazole to give pyridotriazine 2 in good yield. The reaction of the latter with 1-pyrrolidino-1-cyclopentene leads to unsymmetrical bpy derivative 3 with attached cycloalkene ring. On the basis of this prior experience, we have elaborated a route to 7 starting from 3 and using as the key steps: (1) S-transalkylation of 3 with ethyl bromoacetate, and (2) ring-closing metathesis of the resulting alkenyl ether 6. The essential features of the strategy are summarized in Scheme 1.
The utility of S-transalkylation of bpy alkyl sulfides with alkylating agents for the synthesis of functionalized bipyridines is well documented.10, 11 To establish optimal conditions for S-transalkylation of annulated bipyridine 3, the reaction of this compound with benzyl bromide was first investigated. After 7 h stirring at reflux, the desired bis(benzylthio) derivative 4a was formed in 78% yield. As expected, the 1H NMR spectrum of 4a revealed two methylene peaks at 4.58 and 4.63 ppm, which suggest two nonequivalent benzyl groups are present. The reaction of compound 3 and ethyl bromoacetate under the same reaction conditions showed the generality of this process, since the bpy bis(carboxylate) 4b was obtained as a single product in excellent yield. The latter was next reacted with lithium aluminium hydride in THF under reflux for 6 h to provide the corresponding alcohol 5 as colorless solid. Reaction of 5 with allyl bromide in the presence of sodium hydride in DMF at room temperature for 30 min afforded alkenyl ether 6. The conversion of 6 into 2,2′-bipyridine based cyclophane 7 could be easily achieved by ring-closing metathesis. Treatment of 6 with Grubbs’ catalyst I (10 mol %) in a 0.01 M solution of methylene chloride under reflux resulted in the formation of the olefin cyclophane 7 in 70% yields. The ratio of the E/Z isomers in 7 was 10:1. The assignment of configuration in 7 was made by analyzing 13C satellites in its 1H NMR spectrum.12 The structure of the predominant E-isomer (Figure 1) was determined by energy minimization and optimization of all geometrical parameters using AM1 semiempirical method13 implemented in the program package HyperChem.14
The results of these calculations suggested that the pyridine rings are twisted relative to each other by 51.6º. This conformation is probably constrained by steric interactions of the methine hydrogen atoms in both pyridine rings as well by the strain in the chain of the thioether crown caused by rigid vinyl bond.
Further studies on asymmetrical sulfoxidation of 7 with chiral oxidants are under investigations.
EXPERIMENTAL
Melting points were determined in open capillaries and are uncorrected. 1H NMR spectra were recorded on a Varian Gemini 200MHz spectrometr using tetramethylsilane as the internal standard. The IR spectra were measured with a Magna IR-760 spectrophotometr in KBr pellets. Mass spectra were measured on AMD 604 spectrometr (electron impact, 70eV). Elemental analyses were obtained on Perkin-Elmer 2400-CHN analyzer and the results for the indicated elements were within 0.3 % of the calculated values.
Compound 3 was synthesized according to literature procedure.15
Synthesis of 1-(benzylthio)-3-(6-(benzylthio)pyridin-2-yl)-6,7-dihydro-5H-cyclopenta[c]pyridine (4a).
A stirred solution of 3 (130 mg, 0.45 mmol) in benzyl bromide (0.5 mL, 4.21 mmol) was heated under reflux for 7 h. After this time the reaction mixture was cooled and Et2O was added. The precipitate 4a was filtered off and the crude product was purified by column chromatography using CH2Cl2/hexane (10:3) as the eluent. Analytically pure compound, white solid, was recrystallized from MeOH, to provide 154 mg (78%) of 4a. mp 228 ˚C; 1H NMR (CDCl3) δ: 2.14 (qui, 2H, J = 7.4 Hz), 2.85 (t, 2H, J = 7.4 Hz), 2.99 (t, 2H, J = 7.4 Hz), 4.58 (s, 2H), 4.63 (s, 2H), 7.15 (d, 1H, J = 7.8 Hz), 7.27 – 7.48 (m, 10H), 7.57 (t, 1H, J = 7.8 Hz), 8.05 (s, 1H), 8.15 (d, 1H, J = 7.8 Hz); 13C NMR (CDCl3) δ: 24.2, 30.1, 33.0, 33.5, 34.4, 113.4, 116.8, 121.5, 126.9, 127.0, 128.4, 128.7, 128.8, 136.7, 137.2,138.3, 138.7, 152.9, 153.4 154.0, 155.9, 157.5; IR (KBr) cm-1: 1598; 1137; HRMS (m/z) for C27H24N2S2: 440.1376 [M+]; Calcd. 440.1380.
Synthesis of ethyl 2-(6-(1-(2-ethoxy-2-oxoethylthio)-6,7-dihydro-5H-cyclopenta[c]pyridin-3-yl)-pyridin-2-ylthio)acetate (4b).
A stirred solution of 3 (6 g, 20.8 mmol) in ethyl bromoacetate (25 mL, 226 mmol) was heated under reflux for 10 h. After this time the reaction mixture was cooled and Et2O was added. The precipitate 4b was filtered off and the crude product was purified by column chromatography using CH2Cl2 as the eluent. Analytically pure compound, white solid, was recrystallized from a mixture of CH2Cl2/hexane, to provide 8.1 g (90%) of 4b. mp 123 - 125 ˚C; 1H NMR (CDCl3) δ: 1.22 (t, 3H, J=7.2Hz), 1.25 (t, 3H, J=7.1Hz), 2.16 (qui, 2H, J = 7.7 Hz), 2.85 (t, 2H, J = 7.5 Hz), 2.99 (t, 2H, J = 7.7 Hz), 4.02 (s, 2H), 4.03 (s, 2H), 4.17 (q, 2H, J = 7.2 Hz), 4.18 (q, 2H, J = 7.2 Hz), 7.19 (dd, 1H, J = 0.8 Hz, J = 7.9 Hz), 7.58 (t, 1H, J = 7.8 Hz), 8.1 (s, 1H) 8.15 (dd, 1H, J = 0.7 Hz, J = 7.7 Hz); 13C NMR (CDCl3) δ: 14.1, 24.34, 29.9, 32.1, 32.5, 32.9, 61.4, 113.6, 117.0, 121.4, 136.7, 137.2, 151.2, 153.4, 154.2, 155.7, 155.8, 169.6, 169.8; IR (KBr) cm-1: 1744, 1731, 1175, 1144; HRMS (m/z) for C21H24N2S2O4: 432.1173 [M+]; Calcd. 432.1177; Anal. Calcd for C21H24N2S2O4: C, 58.34; H, 5.56; N, 6.48. Found C, 58.50; H, 5.69; N, 6.60.
Synthesis of 2-(6-(1-(2-hydroxyethylthio)-6,7-dihydro-5H-cyclopenta[c]pyridin-3-yl)pyridin-2-ylthio)ethanol (5).
To a suspension of LiAlH4 (250 mg, 6.58 mmol) in THF (14 mL) was added 4b (1.5 g, 3.47 mmol) in THF (40 mL). The reaction mixture was heated under reflux for 4 h under nitrogen. After cooling, THF saturated with water (4 mL), water (4 mL) and KOH 15% aqueous solution (28 mL) was added. The whole was extracted with THF (4 × 15 mL). The combined organic layers were dried over MgSO4. The solvent was evaporated under reduced pressure and the crude product 5 was purified by column chromatography using CH2Cl2/acetone (30:1) as the eluent to give 725 mg (60%) of 5. mp 101 ˚C; 1H NMR (CDCl3) δ: 2.15 (qui, 2H, J = 7.4 Hz), 2.85 (t, 2H, J = 7.5 Hz), 2.95 (t, 2H, J = 7.7 Hz), 3.42 -3.49 (m, 4H), 3.90 – 4.01 (m, 4H), 4.8 – 5.0(brs, 2H) 7.24 (d, 1H, J = 8.4 Hz), 7.59 (t, 1H, J = 7.8 Hz), 7.78 (s, 1H), 7.84 (d, 1H, J = 7.6 Hz); 13C NMR (CDCl3) δ: 24.1, 30.3, 32.7, 33.0, 33.2, 62.7, 63.3, 114.3, 117.4, 122.3, 137.0, 138.0, 153.4, 153.6, 154.5, 155.7, 158.0; IR (KBr) cm-1: 3355.2, 1554,6 1143.9; Anal. Calcd for C17H20N2S2O2: C, 58.62; H, 5.75; N, 8.04. Found C, 58.58; H, 5.93; N, 8.03.
Synthesis of 1-(2-(allyloxy)ethylthio)-3-(6-(2-(allyloxy)ethylthio)pyridin-2-yl)-6,7-dihydro-5H-cyclopenta[c]pyridine (6).
To a mixture of 5 (1.16 g, 3.3 mmol) and NaH (0.46 g, 19.2 mmol) in dry DMF (50 mL), allyl bromide (1.4 mL, 16.2 mmol) in DMF (10 mL) was added. The mixture was stirred at rt for 6 h. The reaction mixture was poured into ice/H2O and acidified with AcOH. For 6 the water layer was extracted with Et2O. The organic layers were dried over MgSO4 and evaporated in vacuo. The crude product 6 was purified by column chromatography using CH2Cl2/acetone (100:1) as the eluent to provide 0.772 g (54%) of 6 as a white oil; 1H NMR (CDCl3) δ: 2.15 (qui, 2H, J = 7.7 Hz), 2.84 (t, 2H, J = 7.4 Hz), 2.92 (t, 2H, J = 7.6 Hz), 3.48 – 3.59 (m, 4H), 3.75 – 3.82 (m, 4H), 4.04 – 4.08 (m, 4H), 5.14 – 5.34 (m, 4H), 5.87 – 6.00 (m, 2H), 7.16 (dd, 1H, J = 0.7 Hz, J = 7.8 Hz), 7.57 ( t, 1H, J = 7.8 Hz), 8.04 (s, 1H), 8.11 (dd, 1H, J = 0.8 Hz, J = 7.7 Hz); 13C NMR (CDCl3) δ: 24.2, 28.6, 29.1, 30.0, 33.0, 69.1, 69.2, 71.9, 113.1, 116.5, 117.0, 121.7, 134.5, 134.6, 136.6, 137.5, 152.4, 153.4, 153.9, 159.0,157.0; IR (KBr) cm-1: 1094, 1142; HRMS (m/z) for C23H28N2S2O2: 428.1603 [M+]; Calcd. 428.1592.
Synthesis of 3,4,6,9,11,12,22,23-octahydro-21H-18,14:19,1-diepiazenocyclopenta[f]-[1,18,4,15]dioxadithiacyclodocosine (7)
A solution each of the substrate 6 (150 mg, 0.35 mmol) in CH2Cl2 (c = 0.01M) and Grubbs’ catalyst I (80 mg, 10 mol %) was heated under reflux for 6 h. The solvent was removed in vacuo and the crude product was separated by column chromatography, using CH2Cl2/hexane (100:1) as the eluent. Analytically pure compound 6 was recrystallized from EtOH as white solid to provide 98 mg (70%) of 7. mp 204 – 206 ºC; 1H NMR (CDCl3) δ: 2.10 (qui, 2H, J = 7.2 Hz), 2.84 (t, 2H, J = 7.6 Hz), 2.99 (t, 2H, J = 7.8 Hz), 3.34 – 3.43 (m, 4H), 3.55 – 3.66 (m, 4H), 3.88 – 4.10 (m, 4H), 5.63 – 5.86 (m, 2H), 7.04 (dd, 1H, J = 0.7 Hz, J = 7.4 Hz), 7.48 (t, 1H, J = 7.8 Hz), 7.95 (s, 1H), 8.01 (dd, 1H, J = 0.7 Hz, J = 7.2 Hz); 13C NMR (CDCl3) δ: 24.23, 26.8, 29.3, 33.0, 51.2, 59.2, 68.9, 70.0, 70.5, 70.6, 70.7, 113.1, 116.5, 121.6, 129.5, 138.4, 137.5, 153.9, 154.3, 155.8, 157.5; IR (KBr) cm-1: 1104, 1142; HRMS (m/z) for C21H24N2S2O2: 400.1273 [M+]; Calcd. 400.1226.
References
1. Part 43 in “1,2,4-Triazines in organic synthesis”. For Part 42, see J. Lawecka, B. Bujnicki, J. Drabowicz, J. Luczak, and A. Rykowski, Phosphorus, Sulfur, Silicon and the Related Elements, 2008, (in print).
2. C. Kaes, A. Katz, and M. W. Hoseini, Chem. Rev., 2000, 100, 353; CrossRef S. H. Bossmann, H. J. Dürr, and M. R. Pokhrel, Synthesis, 2005, 907, and references cited therein. CrossRef
3. N. R. M. Simpson, M. D. Ward, A. F. Morales, B. Ventara, and F. J. Barigeletti, J. Chem. Soc., Dalton Trans., 2002, 2455; CrossRef S. H. Bossmann, H. Seiler, and H. J. Dürr, J. Phys. Org. Chem., 1992, 5, 64. CrossRef
4. J. M. Lehn, Supramolecular Chemistry, Concepts and Perspectives, VCH: Weinheim, 1995.
5. H. Durr and S. H. Bossmann, Acc. Chem. Res., 2001, 34, 905. CrossRef
6. C.-S. Lee, P.-F. Teng, W.-L. Wong, H.-L. Kwong, and A. S. C. Chan, Tetrahedron, 2005, 49, 7924. CrossRef
7. J. Lawecka, E. Olender, P. Piszcz, and A. Rykowski, Tetrahedron Lett., 2008, 49, 723; CrossRef D. Branowska, I. Buczek, K. Kalinska, J. Nowaczyk, and A. Rykowski, Tetrahedron Lett., 2005, 46, 8539. CrossRef
8. L. Summers, Adv. Heterocycl. Chem., 1984, 35, 281. CrossRef
9. D. Branowska, Synthesis, 2003, 2096. CrossRef
10. J. Lawecka, B. Bujnicki, J. Drabowicz, and A. Rykowski, Tetrahedron Lett., 2008, 49, 719. CrossRef
11. D. Branowska, W. Wysocki, and A. Rykowski, Tetrahedron Lett., 2005, 46, 6223. CrossRef
12. J. B. Lambert, H. F. Shuvrell, L. Verbit, R. G. Cooks, and G. H. Stout, in Organic Structural Analysis, Macmillan Publishing: New York, 1976, pp. 91-93.
13. M. J. S. Dewar, E. G. Zoebish, E. F. Healy, and J. P. Stewart, J. Am. Chem. Soc., 1985, 107, 3902. CrossRef
14. HYPERCHEM, rel. 4,5, Hypercube Inc., Waterloo, Ont., Canada, 1998.