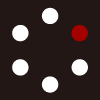
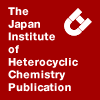
HETEROCYCLES
An International Journal for Reviews and Communications in Heterocyclic ChemistryWeb Edition ISSN: 1881-0942
Published online by The Japan Institute of Heterocyclic Chemistry
e-Journal
Full Text HTML
Received, 27th August, 2008, Accepted, 22nd September, 2008, Published online, 22nd September, 2008.
DOI: 10.3987/COM-08-S(D)13
■ Synthesis, Structure and Catalytic Activity of Macrocyclic NHC Pd Pincer Complexes
Noriaki Watarai, Hiroyasu Kawasaki, Isao Azumaya, Ryu Yamasaki, and Shinichi Saito*
Department of Chemistry, Faculty of Science, Tokyo University of Science, Kagurazaka, Shinjuku, Tokyo 162-8601, Japan
Abstract
We synthesized a series of new N-heterocyclic carbene (NHC) macrocyclic Pd pincer complexes (17-22) by the reaction of Pd(OAc)2 or Pd2(dba)3 with the corresponding macrocycles, which were prepared by the coupling reaction of dibromides with bisimidazoles. The complex adopted twisted conformation, and the activation energy of the conformational interconversion of the macrocycle was determined by VT-NMR. We also examined the catalytic activity of these Pd complexes in Mizoroki-Heck and oxidative alkynyl-alkynyl homocoupling reactions.INTRODUCTION
Transition metal complexes containing N-heterocyclic carbenes (NHCs) are attractive compounds for organic- and organometallic chemists because of its unique structural and catalytic properties.1 Since the first preparation of transition metal complexes containing NHCs was reported by Öfele and Wanzlick,2 the applications of NHC ligands for metal complexes were studied extensively, especially as promising coordinates for homogeneous catalysts in organic synthesis. NHC-Pd complexes turned out to be active catalysts for an enormous number of C-C bond formation reactions such as Mizoroki-Heck reaction,3 Sonogashira coupling,4 Kumada-Corriu coupling,5 Suzuki-Miyaura coupling,6 etc. The structure of the true catalytic species was extensively discussed, and in some reactions, the Pd(0) nanoparticles, instead of the Pd complex, appear to be the true active catalyst.7
Pd pincer complexes are also efficient (pre)catalysts for various reactions and pincer complexes with various ligands have been reported.8 Macrocyclic Pd9 (and Pt)10 pincer complexes were also prepared and the structures and the interaction of the complexes with various compounds were examined. The carbene ligands were also built into a pincer skeleton,11 and recently a series of tridentate NHC Pd pincer complexes have been reported by Crabtree et al.12 These complexes have left- and right-hand twisted conformation, and the interconversion was observed by 1H-NMR spectroscopy. The dependence of the rate of the conformational change on the solvent and temperature has been examined. The complexes were reported as efficient catalysts for some C-C bond formation reactions. While a number of NHC Pd pincer complexes has been reported in the literature,11 the macrocyclic tridentate NHC Pd pincer complexes have not been reported, and the macrocyclic effects on the conformation, stability and catalytic activity of these Pd complexes remains to be explored. Compared to the acyclic complexes, the stability as well as the structure of the macrocyclic complexes would be different, and the catalytic activity of the complex might be of interest. In this paper we report the synthesis, structure and the catalytic activity of the new macrocyclic tridentate NHC Pd pincer complexes.
RESULTS AND DISCUSSION
Synthesis of Macrocyclic Palladium Complexes
We synthesized macrocyclic palladium complexes, which consisted of cyclic CNC-type (CNC = (η3-C,C’,N)(2,6-bis{[N-methyl-N’-methylene]-imidazol-2-ylidene}pyridine)) or CCC-type (CCC = (η3-C,C’,C’’)(2,6-bis{[N-methyl-N’-methylene]imidazol-2-ylidene}phenyl)) pincer moiety.12 The general protocol for the synthesis of macrocyclic precursors was shown in Schemes 1 and 2. Diene 1 was obtained by the coupling reaction13 of α,α’-dibromo-m-xylene with 1-undecenylmagnesium bromide. Hydroboration14 of 1 resulted in the formation of the diol 3, which was converted to the bromide 4 in the presence of bromine and triphenylphosphine.15 Treatment of compound 4 with potassium salt of imidazole in refluxing THF16 resulted in the formation of bisimidazole 5 in 86% yield. We also synthesized bisimidazoles 8 and 10. Thus, diene 2 was prepared by the reaction of resorcinol with 11-bromo-1-undecene in the presence of K2CO3, which was converted to the oxygen containing precursor 8. Compound 9 was prepared by the treatment of resorcinol with 1,6-dibromohexane in the presence of K2CO3 as a base. The reaction of 9 with imidazole led to the formation of 10 in 93% yield.
Macrocyclization was carried out by the coupling reaction of 5, 8, or 10 with 2,6-bis(bromomethyl)pyridine or 1-bromo-2,6-bis(bromomethyl)benzene (Scheme 2). Optimization of the reaction condition led to the isolation of macrocyclic ligands in 43-75% yields when the reaction was carried out under at low concentration (3 mM). Interestingly, the yields of the cyclization reactions were generally higher than those we expected. The observed high yields could be explained in terms of the
intramolecular electrostatic interaction between the imidazole/imidazolium components.17 Thus, the initially formed monoimidazolium salt contains a substituted imidazole and an imidazolium cation moiety, and between these functional groups a favorable attractive interaction might exist (Figure 1). This interaction would accelerate the second intramolecular reaction, leading to the isolation of monomeric 1 : 1 cycloadduct as the major product. Following the reported procedure,12 these macrocycles were reacted with palladium compounds. The CNC-type Pd complexes (17-19) were synthesized by the reaction of 11-13 with Pd(OAc)2 and CCC-type Pd complexes (20-22) were prepared from 14-16 and Pd2(dba)3 (Scheme 3).
Structural studies of the Pd complexes by VT-NMR
We carried out the variable temperature 1H-NMR study and examined the dynamic behavior of the cyclic pincer complexes. The 1H-NMR spectra (500 MHz) of CCC macrocyclic Pd complex 20 in DMF-d7 were shown in Figure 2. Each of benzylic protons (A, in Figure 2) was not equivalent at 20 °C because the interconversion of left- and right-hand twisted conformations was very slow. At the higher temperature, the rate of the interconversion was faster and the coalescence point was determined to be 100 °C. The interconversion was very fast on the 1H-NMR time scale at 100 °C, and the benzylic protons appeared as a singlet.
The coalescence points of other compounds were also measured, and activation energies (ΔG‡) of these dynamic processes were calculated by Oki method.18 The results are summarized in Table 1. The coalescence points of the CCC complexes were about 100 K higher than those of the corresponding CNC complexes (entries 1-3 vs. 4-6). As a result, the ΔG‡ of CCC complexes are higher than that of the corresponding CNC complexes. Faller, Eisenstein, Crabtree and coworkers studied the interconversion of acyclic CNC and CCC complexes and concluded that the mechanism of the interconversion of the CNC complexes is quite different from that of the CCC complexes.12 The interconversion of the CNC complexes proceeds via the coordination of the counter anion to the Pd atom, followed by the decoordination of the pyridyl group. This reaction proceeds faster compared to the interconversion of the CCC complexes which would proceed without the coordination/decoordination of the ligands, and it would be possible to explain our results in a similar manner. As shown by the comparison of entry 2 (38-membered cycle) with entries 7 and 8 (Crabtree's acyclic complexes: wingtip group = Me for 23, and n-Bu for 24), the increased steric bulkiness of the R group leads to the increase in the activation energy of the CNC complexes. The effect of the macrocyclic structure, however, was small, and the ΔG‡ of the macrocyclic complexes reported in entries 1-3 were similar to that of an acyclic complex 24, which was reported by Crabtree et al. (entry 8).12
The activation energies of the interconversions of the CCC complexes 20-22 were also determined and a similar trend was observed. Compared to the ΔG‡ of the acyclic complex bearing methyl group as a
wingtip group (25, entry 9), the ΔG‡ of the cyclic complexes increased (entries 4-6). The difference of the values, however, could be explained in terms of the bulkiness of the substituent rather than the macrocyclic effect. The ΔΔG‡ between 23 (entry 7) and 24 (entry 8), which would be caused by the presence of a bulkier butyl group instead of the small methyl group, is 4.5 kJ/mol, and this value is not significantly different from the ΔΔG‡ (6.1 kJ/mol) between 21 (entry 5) and 25 (entry 9). The results indicate that only a small macrocyclic effect, if any, was observed. The ring size of the macrocyclic complexes might be too large to influence the activation energy.
Catalytic Activity of the Pd complexes
Since recent extensive studies of the NHC-carbene palladium complexes revealed that these complexes were efficient catalysts for various coupling reactions,3-10 we examined the catalytic activity of the Pd complexes in Mizoroki-Heck reaction and oxidative alkynyl-alkynyl homocoupling reaction. The results of Mizoroki-Heck reactions were summarized in Table 2. Treatment of a mixture of 4-iodotoluene (1.0 equiv), butyl acrylate (1.4 equiv), NaOAc (1.1 equiv), n-Bu4NBr (1.0 equiv) and 10 mol % of 17 in DMA at 120 °C3c,8a resulted in the formation of the coupling product 26 in 58% yield (entry 1). The reaction also proceeded when 19, 20, and 22 were used as the catalysts and the product was isolated in 54%, 43%, and 60% yields, respectively (entries 2-4). The differences in the structure of Pd complex and ring size had little effect on the catalytic activity of the complexes. Though we expected the higher activity
(larger turnover number) of the macrocyclic complexes by the stabilizing effect of the macrocyclic structure, the observed catalytic activity of the complexes was not very high. We further examined various reaction conditions and found that the Mizoroki-Heck reaction proceeded smoothly in the presence of sodium formate:19 the higher catalytic activity of 17 and 20 was observed, and only 2 mol % of the catalyst was required. The reaction completed in a short period at 80 °C, the isolated yields of the products were very high (entries 5-6). The results indicate that the formation of the reduced NHC-Pd complex, which would be the active catalytic species for this reaction, is accelerated in the presence of the formate: the low catalytic activity of the Pd complexes under the reaction conditions described in entries 1-4 might be explained in terms of the slow formation of the reduced NHC-Pd complex.20,21
Secondly, we investigated the oxidative alkynyl-alkynyl homocoupling reaction and the results are summarized in Table 3. The reaction of phenylacetylene (27a, 1.0 equiv), Et3N (0.3 equiv), 2 mol% of CuI and 10 mol% of palladium complex in dry DMF22 under O2 atmosphere proceeded efficiently (entry 1-4). The catalytic activity of the CNC-Pd complexes (entries 1-2) was significantly higher than that of the corresponding CCC-Pd complexes (entries 3-4). The low catalytic activity of the CCC-Pd complexes might be attributed to the oxidation state of the Pd complexes: the CNC-Pd complexes would be easily converted to bis(ethynyl) Pd(II) species which should be a key intermediate of this reaction,23 while the CCC-Pd complex would not be easily converted to the corresponding Pd species because of the presence of the Pd-C bond.24 When p-methoxyphenylacetylene (27b) was used as the substrate, the progress of the reaction was slow and it was necessary to carry out the reactions for longer periods (84-124 h) at 50 °C
(entries 5-6). We also carried out the homocoupling reaction25 of 1-iodophenylacetylene (27c) in the presence of 19 or 22 under Ar, and obtained the corresponding coupling product in moderate yields (entries 7-8). It may be possible to explain the observed low catalytic activity in terms of the steric hindrance induced by the macrocyclic ligand, which might retard the oxidative addition of the Pd complex to the C-halogen bond of the substrate.
In conclusion, we synthesized a series of macrocyclic Pd pincer complexes and determined the thermodynamic parameters for the interconversion of the conformers by variable temperature 1H-NMR study. The relationship between the structure and thermodynamic parameters has been examined. The study revealed the macrocyclic effect on the fluxionality of the Pd pincer complexes. Catalytic activity of the Pd complexes in Mizoroki-Heck reactions as well as the alkynyl-alkynyl homocoupling reactions was studied. The high catalytic activity of the macrocycle CNC-type complexes was demonstrated. The relationship between the structure of the catalyst and the catalytic activity of the complex has been discussed.
EXPERIMENTAL
General Methods
All reactions were carried out under Argon atmosphere unless otherwise noted. 1-Bromo-2,6-bis(bromomethyl)benzene26 was prepared according to the literature procedures. NMR spectra were recorded on a JEOL JNM-EX270L FT NMR SYSTEM (270 MHz), a JEOL JNM-EX300L FT NMR SYSTEM (300 MHz), a JEOL JNM-EX500L FT NMR SYSTEM (500 MHz) or a Bruker AM-600 (600 MHz). Chemical shifts were reported in delta units (δ) relative to chloroform-d (7.24 ppm for 1H NMR and 77.0 ppm for 13C NMR) or DMSO-d6 (2.49 ppm for 1H NMR and 39.7 ppm for 13C NMR). Coupling constants, J, are reported in Hz. IR spectra were recorded on a JASCO FT/IR-410 (FT-IR). Elemental analyses were carried out by a YANACO CHN-recorder MT-6. Gel permeation chromatography (GPC) were carried out by a JAPAN ANALITICAL INDUSTRY LC-928 Recycling Preparative HPLC. Thin layer chromatography (TLC) was performed on a Merck silica gel 60F-254 plate. Column chromatography was performed using Kanto Chemical Silica gel 60N (spherical, neutral, 63-210 μm) or Merck Aluminum oxide 90 active neutral.
Synthesis of 1-10.
Compound 1. To Mg turnings (3.16 g, 130 mmol) was added a solution of 11-bromo-1-undecene (17.9 g, 77 mmol) in THF (125 mL) by dropwise addition. Exothermic reaction occurred spontaneously. To a solution of α,α’-dibromo-m-xylene (6.86 g, 20 mmol) and THF (50 mL) was added the Grignard reagent at -70 °C slowly, followed by the addition of Li2CuCl4 (0.1 M in THF, 3.6 mL, 0.36 mmol). The cooling bath was removed, and the reaction mixture was stirred at rt for one night. To the mixture was added saturated NH4Cl aq., water, and the mixture was extracted with Et2O. The organic layer was washed with water, brine, and dried over MgSO4. Evaporation of the solvent gave a colorless oil, which was purified by silica gel column chromatography (n-hexane) to give 1 (6.09 g, 57%): 1H NMR (300 MHz, CDCl3) 7.19-7.13 (t, 1H), 6.98-6.96 (m, 3H), 5.86-5.73 (m, 2H), 5.00-4.89 (m, 4H), 2.57-2.52 (t, J = 7.5, 4H), 2.05-1.99 (q, J = 6.6, 4H), 1.69-1.25 (m, 32H); 13C NMR (75 MHz, CDCl3) 124.8, 139.2, 128.6, 128.0, 125.6, 114.0, 36.0, 33.8, 31.6, 29.6, 29.5(overlap), 29.4 (overlap), 29.1, 28.9; IR (neat) 3076, 2977, 2925, 2853, 1640, 1607, 1588, 1488, 1465, 992, 909, 786, 721, 702, 635 cm-1; Anal. Calcd for C30H50: C, 87.73; H, 12.27. Found: C, 87.38; H, 12.52.
Compound 2. To a stirred solution of resorcinol (1.65 g, 15 mmol), 11-bromo-undec-1-ene (10.50 g, 45 mmol) and acetone (75 mL) was added K2CO3 (5.19 g, 37.5 mmol). After being refluxed for 3 days, the solvent was evaporated. Water was added to the crude product, and the mixture was extracted with EtOAc. The organic layer was washed with water, 3 M NaOH aq., and dried over Na2SO4. Evaporation of the solvent gave a white solid, which was purified by silica gel column chromatography (n-hex : AcOEt = 30 : 1) to give 2 (4.65g, 75%): mp 46-47 °C; 1H NMR (300 MHz, CDCl3) 7.16-7.11 (t, J = 8.1, 1H), 6.48-6.45 (m, 3H), 5.87-5.73 (m, 2H), 5.02-4.90 (m, 4H), 3.93-3.89 (m, 8H), 2.07-2.00 (m, 4H), 1.80-1.71 (m, 4H), 1.50-1.30 (m, 20H); 13C NMR (75 MHz, CDCl3) 160.3, 139.2, 129.7, 114.1, 106.6, 101.3, 67.9, 33.8, 29.5, 29.4, 29.35, 29.4, 29.1, 28.9, 26.0; IR (KBr) 3076, 2924, 2849, 1642, 1621, 1600, 1580, 1495, 1388, 1288, 1186, 1160, 1068, 994, 911, 850, 765, 722, 689, 626 cm-1; Anal. Calcd for C28H46O2: C, 81.10; H, 11.18. Found: C, 80.85; H, 11.48.
Compound 3. To a stirred solution of 1 (5.34 g, 13 mmol) in dry THF (26 mL) at 0 °C was added BH3-THF (0.93 M in THF, 12.4 mL, 11.5 mmol). The mixture was stirred for 5 h at rt, followed by the addition of MeOH (30 mL), 3 M NaOH aq. (4.7 mL), and 30% H2O2 aq. (4.2 mL), keeping the temperature below 40 °C. The mixture was heated to 50 °C and stirred for 1 h, poured into water, and extracted with CH2Cl2. The organic layer was dried over MgSO4, and evaporated. The residue (white solid) was used for the next step without further purification (yield 5.51 g, 95%): mp 54-57 °C; 1H NMR (300 MHz, CDCl3) 7.18-7.13 (t, J = 8.4, 1H), 6.98-6.96 (m, 3H), 3.64-3.60 (t, J = 6.6, 4H), 2.58-2.52 (t, J = 7.5, 4H), 1.64-1.16 (m, 40H); 13C NMR (75 MHz, CDCl3) 142.8, 128.5, 128.0, 125.6, 63.1, 36.0, 32.8, 31.6, 29.6 (overlap), 29.4, 25.7; IR (KBr) 3366, 2920, 2849, 1466, 1347, 1058, 798,723, 698 cm-1; Anal. Calcd for C30H54O2: C, 80.65; H, 12.18. Found: C, 80.78; H, 12.39.
Compound 4. To a stirred solution of PPh3 (8.18 g, 31.2 mmol) in dry CH2Cl2 (45 mL) was added Br2 (1.6 mL, 31.2 mmol). To this mixture was added dropwise a solution of 3 (5.36 g, 12 mmol), pyridine (1.94 mL, 24 mmol) and dry CH2Cl2 (25 mL). After being stirred for 12 h at rt, the mixture was poured into water and extracted with CH2Cl2. The organic layer was washed with saturated Na2S2O3 aq., dried over MgSO4, and evaporated. The crude product was passed through a short silica gel column (CH2Cl2) to remove PPh3O, and evaporated. The crude product was purified by silica gel column chromatography (n-hexane) to give 4 (6.07 g, 88%) as a colorless oil: 1H NMR (300 MHz, CDCl3) 7.19-7.13 (m, 1H), 6.98-6.96 (m, 3H), 3.41-3.63 (t, J = 7.8, 4H), 2.58-2.52 (t, J = 8.1, 4H), 1.89-1.78 (m, 8H), 1.61-1.25 (m, 32H); 13C NMR (75 MHz, CDCl3) 142.8, 128.5, 128.0, 125.6, 36.0, 34.0, 32.8, 31.6, 29.6, 29.5, 29.4 (overlap), 28.8, 28.2; IR (neat) 3076, 3000, 2977, 2925, 2853, 1640, 1607, 1488, 1464, 1444, 992, 909, 786, 721, 702 cm-1; Anal. Calcd for C30H52Br2: C, 62.93; H, 9.15. Found: C, 62.78; H, 9.34.
Compound 5. To a stirred solution of imidazole (2.04 g, 30 mmol) and dry THF (20 mL) was added potassium (1.17 g, 30 mmol). To this mixture was added a solution of 4 (5.50 g, 9.6 mmol) and dry THF (10 mL). After being refluxed for 15 h, the mixture was poured into water and extracted with CH2Cl2. The combined organic layers were dried over MgSO4 and evaporated. The crude product was purified by silica gel column chromatography (CH2Cl2 : MeOH = 20 : 1) to give 5 as a colorless oil (4.50 g, 86%): 1H NMR (300 MHz, CDCl3) 7.43 (s, 2H), 7.18-7.12 (m, 1H) 7.03 (s, 2H) 7.00-6.97 (m, 3H), 6.87 (s, 2H) 3.91-3.86 (t, J = 7.2, 4H), 2.57-2.51 (t, J = 7.5, 4H), 1.78-1.74 (m, 4H), 1.59-1.52 (m, 4H), 1.27-1.23 (m, 32H) ; 13C NMR (75 MHz, CDCl3) 142.8, 137.0, 129.3, 128.5, 128.0, 125.6, 118.7, 47.0, 35.9, 31.5, 31.0, 29.55, 29.51, 29.47 (overlap), 29.4 (overlap), 29.0, 26.5; IR (neat) 3398, 3106, 3020, 2924, 2853, 1682, 1606, 1587, 1507, 1464, 1369, 1282, 1228, 1108, 1077, 1031, 906, 808, 727, 702, 664, 623 cm-1; HRMS(EI) for C36H58N4: 546.4661. Found: 546.4671.
Compound 6. The procedure reported for the synthesis of 3 was generally followed to give 6 as a white solid (yield 93%): mp 96-97 °C; 1H NMR (300 MHz, CDCl3) 7.15-7.10 (t, J = 7.8, 1H), 6.47-6.43 (m, 3H), 3.93-3.88 (t, J = 6.3, 4H), 3.64-3.60 (t, J = 6.6, 4H), 1.79-1.70 (m, 4H), 1.59-1.28 (m, 32H); 13C NMR (75 MHz, CDCl3) 160.3, 129.7, 106.6, 101.4, 67.9, 63.1, 32.8, 29.6, 29.51, 29.48, 29.4, 29.3, 29.2, 26.0, 25.7; IR (KBr) 3422, 3361, 2920, 2848, 1618, 1599, 1580, 1497, 1463, 1389, 1288, 1187, 1161, 1059, 850, 765, 723, 690, 626 cm-1; Anal. Calcd for C28H50O4: C, 74.62; H, 11.18. Found: C, 74.30; H, 11.40.
Compound 7. The procedure reported for the synthesis of 4 was generally followed to give 7 as a white solid (yield 53%). The crude product was purified by silica gel column chromatography (n-hexane/CH2Cl2 5:1). Compound 7: mp 63-64 °C; 1H NMR (300 MHz, CDCl3) 7.16-7.10 (t, J = 8.4, 1H), 6.48-6.43 (m, 3H), 3.93-3.89 (t, J = 6.3, 4H), 3.41-3.37 (t, J = 6.9, 4H), 1.88-1.68 (m, 8H), 1.43-1.28 (m, 28H); 13C NMR (75 MHz, CDCl3) 160.3, 129.7, 106.6, 101,4, 67.9, 34.1, 32.8, 29.5, 29.4, 29.38, 29.3, 29.2, 28.7, 28.2, 26.0; IR (KBr) 2919, 2848, 1618, 1600, 1579, 1494, 1462, 1288, 1188, 1160, 1069, 1050, 850, 765, 722, 689, 647, 626 cm-1; Anal. Calcd for C28H48Br2O2: C, 58.34; H, 8.39. Found: C, 58.22; H, 8.34.
Compound 8. The procedure reported for the synthesis of 5 was generally followed to give 8 as a white solid (yield 78%). Compound 8: mp 76-77 °C; 1H NMR (300 MHz, CDCl3) 7.43 (s, 2H), 7.15-7.09 (t, J = 8.4, 1H), 7.02 (s, 2H), 6.87 (s, 2H), 6.47-6.42 (m, 3H), 3.92-3.81 (m, 8H), 1.78-1.69 (m, 8H), 1.45-1.26 (m, 28H); 13C NMR (75 MHz, CDCl3) 160.3, 137.0, 129.7, 129.3, 118.7, 106.5, 101,3, 67.9, 47.0, 31.0, 29.4, 29.38, 29.35, 29.29, 29.22, 29.0, 26.5, 26.0; IR (KBr) 3107, 2917, 2848, 1619, 1599, 1578, 1508, 1493, 1465, 1386, 1289, 1227, 1185, 1159, 1068, 909, 850, 805, 763, 723, 688, 661, 627 cm-1; Anal. Calcd for C34H54N4O2: C, 74.14; H, 9.88; N, 10.17. Found: C, 74.13; H, 9.98; N, 10.13.
Compound 9. To a stirred solution of resorcinol (2.2 g, 20 mmol), 1,6-dibromohexane (14.6 g, 60 mmol) in acetone (80 mL) was added K2CO3 (7.0 g, 50 mmol). The mixture was refluxed for 3 days and evaporated. The crude product was poured into water and extracted with EtOAc. The organic layer was washed with water and 3 M NaOH aq., and dried over Na2SO4. Evaporation of the solvent gave a white solid, which was purified by silica gel column chromatography (CH2Cl2/n-hexane 1:1) to give 9 (7.24 g, 83%). Compound 9: mp 37-38 °C; 1H NMR (300 MHz, CDCl3) 7.17-7.11 (t, J = 9.0, 1H), 6.48-6.42 (m, 3H), 3.95-3.90 (t, J = 7.2, 4H), 3.43-3.38 (t, J = 7.5, 4H), 1.93-1.72 (m, 8H), 1.51-1.41 (m, 8H); 13C NMR (75 MHz, CDCl3) 160.3, 129.8, 106.6, 101,4, 67.7, 33.8, 32.7, 29.1, 27.9, 25.3; IR (KBr) 2962, 2932, 2848, 1615, 1597, 1581, 1496, 1462, 1451, 1388, 1287, 1188, 1160, 1070, 1031, 850, 764, 724, 689, 649, 625 cm-1; Anal. Calcd for C18H28Br2O2: C, 49.56; H, 6.47. Found: C, 49.67; H, 6.65.
Compound 10. The procedure reported for the synthesis of 5 was generally followed to give 10 as a colorless oil (yield 93%). Compound 10: 1H NMR (300 MHz, CDCl3) 7.44 (s, 2H), 7.16-7.10 (t, J = 9.0, 1H), 7.03 (s, 2H), 6.88 (s, 2H), 6.47-6.39 (m, 3H), 3.94-3.87 (m, 8H), 1.84-1.68 (m, 8H), 1.53-1.27 (m, 8H); 13C NMR (75 MHz, CDCl3) 160.2, 137.0, 129.9, 129.4, 118.8, 106.6, 101.3, 67.5, 46.9, 31.0, 29.1, 26.3, 25.6; IR (neat) 3387,3107, 2937, 2860, 1602, 1508, 1492, 1469, 1391,1334, 1286, 1264, 1229, 1183, 1153, 1109, 1179, 1037, 907, 822, 764, 739, 688, 665, 626 cm-1 ; HRMS(EI) Calcd for C24H34N4O2: 410.2682. Found: 410.2687.
A Representative Procedure for the Synthesis of Macrocycles 11-16. Synthesis of 11. To a stirred solution of 5 (1.09 g, 2.0 mmol) and acetone (640 mL) was added 2,6-bis(bromomethyl)pyridine (0.530 g, 2.0 mmol) or (0.685 g, 2.0 mmol). After being refluxed for 12 h, the mixture was cooled to rt, passed through grass filter and the residue was evaporated. The crude product was white solid, which was purified by alumina gel column chromatography (CH2Cl2/MeOH 20:1) to give 11 (0.882 g, 54%): mp 109-110 °C; 1H NMR (300 MHz, DMSO-d6) 9.35 (br, 2H), 7.99-7.94 (t, J = 7.5, 1H), 7.82 (br, 2H), 7.70 (br, 2H), 7.54-7.51 (d, J = 7.5, 2H), 7.17-7.12 (t, J = 8.4, 1H), 6.97-6.94 (m, 3H), 5.55 (br, 4H), 4.19 (br, 4H), 2.495 (m, 4H, overlapped with the signal of CD2HSOCD3), 1.77(br, 4H), 1.53 (br, 4H), 1.25-1.19 (br, 32H); 13C NMR (150 MHz, DMSO-d6) 153.6, 141.9, 139.0, 136.8, 128.8, 128.4, 125.9, 123.5, 122.5, 122.3, 52.7, 49.0, 35.0, 30.8, 29.7, 29.2, 29.1 (overlap), 29.0, 28.7, 28.1, 25.8; IR (KBr) 3388, 3127, 2920, 2850, 1631, 1599, 1561, 1523, 1461, 1438, 1353, 1327, 1257, 1160, 1097, 1066, 762, 721, 703, 663, 639; HRMS(FAB+) Calcd for C43H65BrN5: 730.4423. Found: 730.4423.
Compound 12. white amorphous (70% yield): mp 74-76 °C; 1H NMR (300 MHz, DMSO-d6) 9.51 (s, 2H), 7.98-7.93 (t, J = 7.8, 1H), 7.83 (s, 2H), 7.74 (s, 2H), 7.51-7.49 (d, J = 7.8, 2H), 7.15-7.10 (t, J = 7.8, 1H) 6.48-6.43 (m, 3H), 5.56 (s, 4H), 4.25-4.20 (t, J = 10.5, 4H) 4.00-3.92 (t, J = 6.0, 4H), 1.80-1.64 (m, 8H), 1.38-1.24 (m, 28H); 13C NMR (150 MHz, DMSO-d6) 153.7, 142.0, 139.1, 136.9, 128.9, 128.5, 126.0, 123.6, 122.6, 122.3, 52.8, 49.1, 35.0, 30.8, 29.8, 29.3, 29.2 (overlap), 29.0, 28.7, 28.1, 25.9; IR (KBr) 3423, 3123, 3066, 2926, 2853, 1727, 1604, 1591, 1552, 1492, 1463, 1386, 1335, 1288, 1265, 1183, 1156, 1066, 1028, 997, 838, 825, 759, 684, 661, 640; HRMS(FAB+) Calcd for C41H61BrN5O2: 734.4009. Found: 734.4005.
Compound 13. white amorphous (43% yield): 1H NMR (500 MHz, DMSO-d6) 9.59 (s, 2H), 7.94-7.91 (t, J = 7.5, 1H), 7.83 (s, 2H), 7.73 (s, 2H), 7.46-7.45 (d, J = 7.5, 2H), 7.14-7.11 (t, J = 7.5, 1H), 6.47-6.45 (m, 3H), 5.52 (s, 4H), 4.29-4.23 (t, J = 7.0, 4H), 3.98-3.96 (t, J = 6.5, 4H), 1.85-1.79 (m, 4H), 1.71-1.66 (m, 4H), 1.48-1.42 (m, 4H), 1.33-1.27 (m, 4H); 13C NMR (75 MHz, DMSO-d6) 160.0, 137.1, 135.2, 131.5, 130.3, 129.1, 125.0, 123.2, 106.9, 101.7, 67.1, 52.8, 49.1, 48.9, 29.1, 28.2, 24.8, 24.7. Compound 13 was very hygroscopic and was used for the next step without further purification.
Compound 14. white amorphous (58% yield): 1H NMR (300 MHz, DMSO-d6) 9.33 (s, 2H), 7.86 (s, 2H), 7.76 (s, 2H), 7.58 (m, 3H), 7.16-7.11 (t, J = 7.8, 1H) 6.95-6.93 (m, 3H), 5.55 (s, 4H), 4.20-4.15 (t, J = 6.9, 4H), 1.76-1.71 (m, 4H), 1.54-1.44 (m, 4H), 1.35-1.10 (m, 36H); 13C NMR (75 MHz, CDCl3) 142.5, 136.5, 133.9, 133.0, 129.4, 128.6, 128.0, 126.8, 125.6, 123.8, 121.5, 53.4, 50.0, 35.6, 31.2, 30.3, 29.3, 29.2 (overlap), 28.8, 28.7, 26.1; IR (KBr) 3386, 3134, 3096, 3027, 2922, 2851, 1735, 1627, 1560, 1458, 1436, 1341, 1254, 1159, 1113, 1029, 835, 750, 620; mp 132-134 °C; HRMS(FAB+) Calcd for C44H65Br2N4: 807.3576. Found: 807.3577.
Compound 15. white amorphous (75% yield): mp 85-86 °C; 1H NMR (300 MHz, DMSO-d6) 9.33 (s, 2H), 8.31 (s, 2H), 7.86 (s, 2H), 7.73 (s, 2H), 7.58-7.53 (t, J = 7.5, 1H), 7.46-7.42 (d, J = 7.2, 2H), 7.15-7.10 (t, J = 8.4, 1H), 6.47-6.41 (m, 3H) 5.55 (s, 4H), 4.20-4.16 (t, J = 6.9, 4H), 3.93-3.89 (t, J = 6.0, 4H), 1.76-1.63 (m, 8H), 1.37-1.22 (m, 26H) ; 13C NMR (150 MHz, DMSO-d6) 159.9, 136.9, 135.0, 131.6, 130.0, 129.0, 124.9, 122.89, 122.86, 106.6, 101.1, 79.2, 67.2, 52.6, 49.0, 29.2, 28.8, 28.7, 28.6, 28.5, 28.1, 25.4, 25.3; IR (KBr) 3399, 3133, 3076, 2990, 2924, 2853, 1597, 1561, 1491, 1466, 1435, 1383, 1335, 1285, 1263, 1153, 1112, 1077, 1027, 882, 833, 776, 688, 654, 621; HRMS(FAB+) Calcd for C42H61Br2N4O2: 811.3161. Found: 811.3157.
Compound 16. white amorphous (64% yield): 1H NMR (300 MHz, DMSO-d6) 9.35 (s, 2H), 7.88 (s, 2H), 7.76 (s, 2H), 7.46-7.43 (t, J = 8.0, 1H), 7.34-7.32 (d, J = 7.5, 2H), 7.15-7.11 (t, J = 8.5, 1H), 6.46-6.39 (d, J = 8.5, 2H), 6.39 (s, 1H), 5.50 (s, 4H), 4.24-4.22 (t, J = 5.5, 4H), 3.89-3.86 (t, J = 6.0, 4H), 1.80-1.76 (m, 4H), 1.66-1.61 (m, 4H), 1.39-1.34 (m, 4H), 1.21-1.17 (m, 4H); 13C NMR (150 MHz, DMSO-d6) 160.0, 137.0, 125.2, 131.4, 130.2, 129.1, 124.8, 123.2, 123.1, 106,8, 101.6, 67.1, 52.7, 49.0, 29.1, 28.2, 24.8, 24.6. Compound 16 was very hygroscopic and was used for the next step without further purification.
A Representative Procedure for the Synthesis of the CNC Type Pd Complexes 17-19.
Synthesis of 17. A solution of 11 (0.812 g, 1.0 mmol) and Pd(OAc)2 (0.269 g, 1.20 mmol) was stirred in DMSO (4.0 mL) for 2 h at rt, then for 12 h at 50 °C, and finally for 1 h at 150 °C. DMSO was removed by distillation under reduced pressure, and the residue was diluted by CH2Cl2 and passed through Celite. Evaporation of the solvent gave a crude product, which was purified by silica gel column chromatography (CH2Cl2/MeOH 20:1) to give 17 (0.44 g, 48%). Further purification was carried out by recrystallization from Et2O/MeOH. Compound 17, yellow solid: mp 287 °C; 1H NMR (300 MHz, CDCl3) 8.23-8.21 (d, J = 7.5, 2H), 7.93-7.88 (t, J = 7.5, 1H), 7.72 (s, 2H), 7.18-7.13 (t, J = 7.8, 1H), 6.97-6.94 (m, 3H), 6.86 (s, 2H), 5.80 (br, 4H), 4.33 (br, 4H), 2.58-2.53 (t, J = 7.8, 4H), 1.87 (br, 4H), 1.63-1.23 (m, 36H); 13C NMR (75 MHz, CDCl3) 164.4, 155.0, 142.7, 141.2, 128.6, 128.1, 126.3, 125.7, 122.5, 121.0, 54.7, 51.0, 35.8, 31.5, 31.4, 29.3, 29.12, 29.08, 29.0 (overlap), 28.9, 26.3; IR (KBr) 3422, 2922, 2850, 1607, 1458, 1422, 1373, 1338, 1269, 1236, 1166, 779, 737, 702, 674, 620, 593 cm-1; Anal. Calcd for C43H63Br2N5Pd•2H2O: C, 54.24; H, 7.09; N, 7.35. Found: C, 54.26; H, 7.07; N, 7.30.
Compound 18. yellow solid (43% yield): mp 288 °C; 1H NMR (300 MHz, CDCl3) 8.23-8.21 (d, J = 7.8, 2H), 7.95-7.90 (t, J = 7.5, 1H), 7.70 (s, 2H), 7.24-7.12 (m, 1H), 6.86 (s, 2H), 6.48-6.45 (m, 3H), 4.32 (br, 4H), 3.95-3.91 (t, J = 6.6, 4H), 1.85 (br, 4H), 1.78-1.69 (m, 4H), 1.42-1.25 (m, 28H), four proton signals were missing, probably because of the broadening; 13C NMR (75 MHz, CDCl3) 164.4, 160.3, 155.0, 141.3, 129.8, 126.3, 122.5, 121.1, 106.7, 101,31, 67.7, 54.7, 50.9, 31.6, 29.22, 29.19, 29.0, 28.9, 28.8 (overlap), 26.3, 25.8; IR (KBr) 3422, 3154, 3119, 3088, 2922, 2851, 1604, 1491, 1458, 1422, 1336, 1287, 1264, 1184, 1149, 1031, 770, 740, 687, 674, 593 cm-1; Anal. Calcd for C41H59Br2N5O2Pd •2H2O: C, 51.50; H, 6.64; N, 7.32. Found: C, 51.90; H, 6.51; N, 7.07.
Compound 19, yellow solid (35% yield): mp 285 °C; 1H NMR (300 MHz, CDCl3) 8.22-8.19 (d, J = 7.8, 2H), 7.94-7.88 (d, J = 7.6, 1H), 7.70 (d, J = 1.6, 2H), 7.12-7.06 (t, J = 8.4, 1H), 6.88 (d, J = 1.9, 2H), 6.52(s, 1H), 6.45-6.42(d, J = 8.1, 2H), 6.1-5.5 (br, 4H), 4.7-3.9 (br, 4H), 4.01-3.96 (t, J = 6.2, 4H), 2.0-1.5 (m, 16H); 13C NMR (150 MHz, CDCl3) 164.4, 160.3, 155.1, 141.4, 129.6, 126.3, 122.6. 120.6, 107.5, 100.5, 67.0, 54.8, 50.5, 30.6, 27.7, 25.0, 24.5; IR (KBr) 3419, 3154, 3120, 3088, 2932, 2857, 1600, 1491, 1461, 1426, 1335, 1285, 1265, 1181, 1150, 1026, 774, 740, 685, 592 cm-1; Anal. Calcd for C31H39Br2N5O2Pd: C, 47.74; H, 5.04; N, 8.98. Found: C, 47.80; H, 5.04; N, 8.71.
A Representative Procedure for the Synthesis of the CCC Type Pd Complexes 20-22. Synthesis of 20. A solution of 14 (0.603 g, 0.68 mmol), Pd2(dba)3 (0.457 g, 0.88 mmol), Na2CO3 (0.283 g, 2.72 mmol) was stirred in degassed DMSO (10.4 mL) for 6 h at 50 °C and then 18 h at 125 °C. The solvent was removed by distillation under reduced pressure. The residue was diluted by CH2Cl2, and passed through Celite. Evaporation of the solvent gave the crude product, which was further purified by silica gel column chromatography (CH2Cl2) to give 20 (0.34 g, 41%) as white powder. Compound 20: mp 118 °C; 1H NMR (300 MHz, CDCl3) 7.24-7.17 (t, J = 12.9, 1H), 6.98 (s, 2H), 6.96-6.91 (m, 6H), 6.86-6.79 (m, 1H), 6.72 (m, 1H), 5.43-5.39 (d, J = 13.5, 2H), 4.67-4.54 (m, 4H), 4.37-4.25 (m, 2H), 2.59-2.54 (t, J = 7.8, 4H), 1.99 (br, 2H), 1.59 (br, 2H), 1.61-1.55 (m, 4H), 1.26-1.18 (m, 32H); 13C NMR (75 MHz, CDCl3) 177.0, 150.3, 142.9, 141.0, 128.6, 128.0, 125.6, 124.8, 122.7, 119.9, 119.3, 58.4, 50.4, 36.0, 31.7, 31.5, 29.32, 29.26, 29.2 (overlap), 29.13, 29.05, 28.9, 26.6; IR (KBr) 3447, 3122, 3049, 2924, 2851, 1654, 1648, 1559, 1541, 1522, 1508, 1459, 1447, 1419, 1375, 1339, 1315, 1244, 1222, 1165, 1103, 930, 758, 718, 679 cm-1; Anal. Calcd for C44H63BrN4Pd: C, 63.34; H, 7.61; N, 6.72. Found: C, 63.05; H, 7.66; N, 6.77.
Compound 21, white powder (yield 50%): mp 112-113 °C; 1H NMR (300 MHz, CDCl3) 7.17-7.11 (t, J = 8.4, 1H), 6.96-6.94 (d, J = 7.5, 2H), 6.91 (s, 2H), 6.86-6.81 (t, J = 6.6, 1H), 6.71 (s, 2H), 6.53-6.45 (m, 3H), 5.42-5.38 (d, J = 13.2, 2H), 4.60-4.56 (d, J = 13.2, 2H), 4.50-4.45 (t, J = 7.2, 4H), 3.96-3.91 (t, J = 6.3, 4H), 2.01-1.95 (m, 4H), 1.78-1.69 (m, 8H), 1.27-1.20 (m, 24H); 13C NMR (75 MHz, CDCl3) 177.4, 160.4, 152.0, 141.0, 129.7 124.6, 122.6, 120.0, 119.4, 106.8, 101.1, 67.8, 58.5, 50.9, 31.4, 29.2 (overlap), 29.0, 28.96, 28.8, 28.7, 26.3, 25.7; IR (KBr) 3447, 3155, 3129, 3089, 3045, 3031, 2921, 2850, 1603, 1493, 1459, 1412, 1377, 1335, 1313, 1291, 1264, 1182, 1156, 1028, 834, 760, 718, 687, 677, 584 cm-1; Anal. Calcd for C42H59BrN4O2Pd: C, 60.18; H, 7.09; N, 6.68. Found: C, 60.29; H, 7.15; N, 6.43.
Compound 22, white solid (yield 37%): mp 145 °C; 1H NMR (300 MHz, CDCl3) 7.07-7.01 (t, J = 8.1, 1H), 6.98-6.95 (m, 4H), 6.87-6.82 (t, J = 6.6, 1H), 6.77 (d, J = 1.8, 2H), 6.66 (s, 1H), 6.43-6.40 (d, J = 7.8, 2H), 5.45-5.41 (d, J = 13.2, 2H), 5.23-5.13 (m, 2H), 4.61-4.57 (d, J = 13.5, 2H), 4.14-4.01 (m, 4H), 3.69-3.60 (m, 2H), 2.16-2.11 (m, 2H), 1.85-1.53 (m, 14H); 13C NMR (75 MHz, CDCl3) 177.1, 160.5, 141.1, 129.2, 124.8, 122.8, 120.4, 118.7, 108.0, 99.0, 77.2, 66.6, 58.5, 50.4, 30.4, 27.2, 24.7, 23.8; IR (KBr) 3447, 3127, 3049, 2932, 2858, 1596, 1493, 1460, 1446, 1419, 1380, 1285, 1246, 1218, 1182, 1148, 1081, 1025, 819, 759, 720, 682 cm-1; Anal. Calcd for C32H39BrN4O2Pd: C, 55.06; H, 5.63; N, 8.03. Found: C, 54.90; H, 5.64; N, 7.88.
General Procedure for Mizoroki-Heck Reaction in the Presence of Bu4NBr (Table 2, entries 1-4).
To a stirred solution of 4-iodotoluene (0.10 mmol), Bu4NBr (0.10 mmol, 1.0 equiv), NaOAc (0.11 mmol, 1.1 equiv) and 10 mol% of the palladium complex (0.010 mmol) in 0.5 mL of dry DMA was added butyl acrylate (0.14 mmol, 1.4 equiv) under Argon atmosphere. After being heated at 120 °C for an appropriate period, the reaction mixture was cooled to rt. The solvent was removed under reduced pressure, and water was added to the residue. The mixture was extracted with CH2Cl2, and the combined organic layers were dried over MgSO4. Evaporation of the solvent gave colorless oil, which was further purified by silica gel column chromatography using n-hexane/CH2Cl2 3:1 as eluent.
General Procedure for Mizoroki-Heck Reaction in the Presence of HCO2Na (Table 2, entries 5-6).
To a stirred solution of 4-iodotoluene (1.0 mmol), HCO2Na (0.1 mmol), and palladium complex (0.020 mmol) in 1.0 mL of dry DMF was added i-Pr2NEt (1.5 mmol) and butyl acrylate (1.2 mmol) under argon atmosphere. After being heated at 80 °C for an appropriate period, the reaction mixture was cooled to rt. The solvent was removed under reduced pressure, and water was added to the residue. The mixture was extracted with CH2Cl2, and the combined organic layers were dried over MgSO4. Evaporation of the solvent gave colorless oil, which was further purified by silica gel column chromatography using n-hexane/CH2Cl2 3:1 as eluent.
General Procedure for the Oxidative Homocoupling Reactions of 1-alkynes (Table 3, entries 1-6).
To a stirred solution of phenylacetylene (0.10 mmol), CuI (0.0020 mmol), palladium complex (0.010 mmol) in 0.5 mL of dry DMF was added Et3N under oxygen atmosphere. After being stirred at appropriate temperature, the reaction mixture was cooled to rt, and the solvent was removed under reduced pressure. The mixture was poured into water, and extracted with CH2Cl2. The combined organic layers were dried over MgSO4. Evaporation of the solvent gave a solid, which was further purified by silica gel column chromatography using n-hexane as eluent.
General Procedure for the Homocoupling Reactions of 1-iodoalkynes (Table 3, entries 7-8).
A stirred solution of 1-iodophenylacetylene (0.10 mmol), palladium complex (0.010 mmol) in 0.5 mL of dry DMF was stirred under argon atmosphere at 50 °C for an appropriate period. The reaction mixture was cooled to rt, and the solvent was removed under reduced pressure. The mixture was poured into water, and extracted with CH2Cl2. The combined organic layers were washed with brine and dried over MgSO4. Evaporation of the solvent gave a solid, which was further purified by silica gel column chromatography using n-hexane as eluent.
References
1. For review see; (a) T. Weskamp, V. P. W. Böhm, and W. A. Herrmann, J. Organomet. Chem., 2000, 600, 12; CrossRef (b) D. Bourissou, O. Guerret, F. P. Gabbaï, and G. Bertrand, Chem. Rev., 2000, 100, 39; CrossRef (c) A. C. Hillier, G. A. Grasa, M. S. Viciu, H. M. Lee, C. Yang, and S. P. Nolan, J. Organomet. Chem., 2002, 653, 69; CrossRef (d) W. A. Herrmann, Angew. Chem. Int. Ed., 2002, 41, 1290; CrossRef (e) W. A. Herrmann, K. Öfele, D. V. Preysing, and S. K. Schneider, J. Organomet. Chem., 2003, 687, 229; CrossRef (f) E. Peris and R. H. Crabtree, Coord. Chem. Rev., 2004, 248, 2239; CrossRef (g) C. M. Crudden and D. P. Allen, Coord. Chem. Rev., 2004, 248, 2247; CrossRef (h) R. H. Crabtree, J. Organomet. Chem., 2005, 690, 5451; CrossRef (i) E. A. B. Kantchev, C. J. O‘Brien, and M. G. Organ, Angew. Chem., Int. Ed., 2007, 46, 2768. CrossRef
2. (a) K. Öfele, J. Organomet. Chem., 1968, 12, P42; CrossRef (b) H. W. Wanzlick and H. J. Schoenherr, Angew. Chem., Int. Ed. Engl., 1968, 7, 141. CrossRef
3. (a) W. A. Herrmann, M. Elison, J. Fischer, C. Köcher, and G. R. J. Artus, Angew. Chem. Int. Ed. Engl., 1995, 34, 2371; CrossRef (b) D. S. McGuinness and K. J. Cavell, Organometallics, 2000, 19, 741; CrossRef (c) E. Peris, J. Mata, J. A. Loch, and R. H. Crabtree, Chem. Commun., 2001, 201. CrossRef
4. M. Eckhardt and G. C. Fu, J. Am. Chem. Soc., 2003, 125, 13642. CrossRef
5. A. C. Frisch, F. Rataboul, A. Zapf, and M. Beller, J. Organomet. Chem., 2003, 687, 403. CrossRef
6. (a) R. Singh, M. S. Viciu, N. Kramareva, O. Navarro, and S. P. Nolan, Org. Lett., 2005, 7, 1829; CrossRef (b) O. Navarro, H. Kaur, P. Mahjoor, and S. P. Nolan, J. Org. Chem., 2004, 69, 3173; CrossRef (c) G. Altenhoff, R. Goddard, C. W. Lehmann, and F. Glorius, Angew. Chem., Int. Ed., 2003, 42, 3690. CrossRef
7. (a) M. R. Eberhard, Org. Lett., 2004, 6, 2125; CrossRef (b) D. E. Bergbreiter, P. L. Osburn, and J. D. Frels, Adv. Synth. Catal., 2005, 347, 172; CrossRef (c) K. Yu, W. Sommer, J. M. Richardson, M. Weck, and C. W. Jones, Adv. Synth. Catal., 2005, 347, 161; CrossRef (d) K. Yu, W. Sommer, M. Weck, and C. W. Jones, J. Catal., 2004, 226, 101; CrossRef (e) W. J. Sommer, K. Yu, J. S. Sears, Y. Ji, X. Zheng, R. J. Davis, C. D. Sherrill, C. W. Jones, and M. Weck, Organometallics, 2005, 24, 4351; CrossRef (f) N. T. S. Phan, M. V. D. Sluys, and C. W. Jones, Adv. Synth. Catal., 2006, 348, 609. CrossRef
8. (a) D. Morales-Morales, C. Grause, K. Kasaoka, R. Redon, R. E. Cramer, and C. M. Jensen, Inorg. Chim. Acta, 2000, 300-302, 958; CrossRef (b) D. Morales-Morales, R. Redon, C. Yung, and C. M. Jensen, Chem. Commun., 2000, 1619; CrossRef (c) M. R. Eberhard, Z. Wang, and C. M. Jensen, Chem. Commun., 2002, 818; CrossRef (d) M. Ohff, A. Ohff, M. E. van der Boom, and D. Milstein, J. Am. Chem. Soc., 1997, 119, 11687; CrossRef (e) M. Ohff, A. Ohff, M. E. van der Boom, and D. Milstein, J. Am. Chem. Soc., 1998, 120, 3273; CrossRef (f) F. Miyazaki, K. Yamaguchi, and M. Shibasaki, Tetrahedron Lett., 1999, 40, 7379; CrossRef (g) A. S. Gruber, D. Zim, G. Ebeling, A. L. Monteiro, and J. Dupont, Org. Lett., 2000, 2, 1287. CrossRef
9. (a) J. E. Kickham and S. J. Loeb, Inorg. Chem., 1994, 33, 4351; CrossRef (b) J. E. Kickham and S. J. Loeb, Inorg. Chem., 1995, 34, 5656; CrossRef (c) B. R. Cameron, S. J. Loeb, and G. P. A. Yap, Inorg. Chem., 1997, 36, 5498. CrossRef
10. J. Terheijden, G. Van Koten, J. A. M. Van Beek, B. K. Vriesema, R. M. Kellogg, M. C. Zoutberg, and C. H. Stam, Organometallics, 1987, 6, 89. CrossRef
11. Reviews for the bis- and tris-NHC carbene complexes: (a) D. Pugh and A. A. Danopoulos, Coord. Chem. Rev., 2007, 251, 610; CrossRef (b) J. A. Mata, M. Poyatos, and E. Peris, Coord. Chem. Rev., 2007, 251, 841. CrossRef
12. (a) S. Gründemann, M. Albrecht, J. A. Loch, J. W. Faller, and R. H. Crabtree, Organometallics, 2001, 20, 5485; CrossRef (b) J. R. Miecznikowski, S. Grundemann, M. Albrecht, C. Mégret, E. Clot, J. W. Faller, O. Eisenstein, and R. H. Crabtree, Dalton Trans., 2003, 831. CrossRef
13. R. R. Srivastava, R. R. Singhaus, and G. W. Kabalka, J. Org. Chem., 1999, 64, 8495. CrossRef
14. V. Percec and Y. Tsuda, Macromolecules, 1990, 23, 3509. CrossRef
15. M. Bartra and J. Vilarrasa, J. Org. Chem., 1991, 56, 5132. CrossRef
16. S. K. Sharma, M. Tandon, and J. W. Lown, J. Org. Chem., 2000, 65, 1102. CrossRef
17. The strong interaction between imidazole and imidazole cation was examined theoretically. See, S. Yan, Y. Bu, Z. Cao, and P. Li, J. Phys. Chem. A, 2004, 108, 7038. CrossRef
18. M. Oki, ‘Application of Dynamic NMR Spectroscopy to Organic Chemistry’, VCH Publishers, Florida, 1985. The relationship between the rate constant and the NMR parameters was obtained by the following equation:
(equation)
where kc is the rate constant at the coalescence point, δν is the difference in chemical shifts (in Hz), and J is coupling constant. The signals of the benzylic protons (signals of the methylene protons located between the NHC group and the phenyl group) appeared as coupled AB signals at low temperature, and they were analyzed to calculate the parameters.
19. J. Pytkowicz, S. Roland, P. Mangeney, G. Meyer, and A. Jutand, J. Organomet. Chem., 2003, 678, 166. CrossRef
20. The decomposition of the Pd complexes to colloidal Pd might have occured at the elevated temparature (120 °C), since the color of the reaction mixure turned brown. The decomposition of the Pd catalyst seems to be suppressed at 80 °C (entries 5-6).
21. Though we examined the reaction of some aryl bromides, the reactions proceeded very slowly, indicating the lower catalytic activity of the macrocyclic Pd complexes compared to the acyclic analogues. See, ref. 12a.
22. (a) S. Takano, T. Sugihara, and K. Ogasawara, Tetrahedron Lett., 1991, 32, 2797; CrossRef (b) S. Takano, T. Sugihara, and K. Ogasawara, Synlett, 1990, 453. CrossRef
23. (a) R. Rossi, A. Carpita, and C. Bigelli, Tetrahedron Lett., 1985, 26, 523; CrossRef (b) Q. Liu and D. J. Burton, Tetrahedron Lett., 1997, 38, 4371. CrossRef
24. Unless the Pd-C bond is cleaved, it is necessary to postulate a less stable Pd(III) complex as an intermediate.
25. S. V. Damle, D. Seomoon, and P. H. Lee, J. Org. Chem., 2003, 68, 7085. CrossRef
26. L. A. van de Kuil, H. Luitjes, D. M. Grove, J. W. Zwikker, J. G. M. van der Linden, A. M. Roelofsen, L. W. Jenneskens, W. Drenth, and G. van Koten, Organometallics, 1994, 13, 468 CrossRef