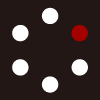
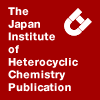
HETEROCYCLES
An International Journal for Reviews and Communications in Heterocyclic ChemistryWeb Edition ISSN: 1881-0942
Published online by The Japan Institute of Heterocyclic Chemistry
e-Journal
Full Text HTML
Received, 29th August, 2008, Accepted, 6th October, 2008, Published online, 9th October, 2008.
DOI: 10.3987/COM-08-11536
■ First Formation of Thiepino[2,3-b]- and Thiepino[3,2-a]indolizine Derivatives
Hidetoshi Isawa, Hiroyuki Suga, and Akikazu Kakehi*
Department of Chemistry and Material Engineering, Faculty of Engineering, Shinshu University, Wakasato, Nagano 380-8553, Japan
Abstract
The reactions of potassium 1,3-bis(ethoxycarbonyl)indolizine-2-thiolates with ethyl 4-bromocrotonate afforded the corresponding indolizine derivatives having (E)-(3-ethoxycarbonyl-2-propenyl)thio, (E)- and (Z)-(3-ethoxycarbonyl-1-propenyl)thio group at the 2-position. The alkaline treatment of these S-alkylated indolizines gave the title compounds in good yields.We previously reported that potassium indolizine-2-thiolates bearing electron-withdrawing groups at the 1-, and 3-positions were useful precursors for the preparation of some novel nitrogen-bridged heterocycles such as thieno[2,3-b]-, thieno[3,2-a]-, 2,3 and thiino[2,3-b]indolizine derivatives.4 The key step in above reactions was the intramolecular nucleophilic addition of the carbanion species generated in the 2-substituent onto a cyano or an acyl carbonyl group at the 1- or 3-position. Subsequent hydrogen transfer or elimination from the primary cycloadducts provided the corresponding heterocycles. As an extension of this reaction, we were interested in the behavior of indolizine derivatives having a vinylogous carbanion (allyl anion) in the 2-substituent, because species such as Ia should form 2-vinylthiophenes II, but those such as Ib should provide thiepins III which are not readily accessible (see Figure 1). In general, the route leading to five-membered heterocycles such as II is more favorable than that to provide seven-membered heterocycles such as III because of the smooth approach of the reaction sites in the transition state and of ready stabilization (aromatization) of the primary adducts. However, an example in which seven-membered products were exclusively formed is also known.5 Here we describe the first formations of thiepino[2,3-b]- and thiepino[3,2-a]indolizine derivatives from the alkaline treatment of diethyl 1,3-indolizinedicarboxylates having an (E)-(3-ethoxycarbonyl-2- propenyl)thio, (E)- and (Z)-(3-ethoxycarbonyl-1- propenyl)thio group at the 2-position.
When potassium 1,3-bis(ethoxycarbonyl)indolizine-2-thiolate (2a), generated in situ by the reactions of diethyl 2-[(2-ethoxycarbonylethyl)thio]indolizine-1,3-dicarboxylate (1a) with potassium tert-butoxide in
DMF, was treated with ethyl 4-bromocrotonate (3), a mixture of diethyl 2-[(E)-(3-ethoxycarbonyl-2- propenyl)thio]- (4a), diethyl 2-[(E)-(3-ethoxycarbonyl-1-propenyl)thio]- (5a), and diethyl 2-[(Z)-(3-ethoxycarbonyl-1-propenyl)thio]indolizine-1,3-dicarboxylates (6a) was obtained in a 49% yield as a pale yellow oil, and its ratio was 100:6:3.6,7 Similar treatment of S-protected indolizines 1b,c with 3 afforded the mixtures of the corresponding S-alkylated indolizines 4b+5b+6b (its ratio is 100:4:2) and 4c+5c+6c (its ratio is 50:11:9) in 40% and 33% yields.6,7 Since all of indolizines 4a—c, 5a—c, and 6a—c thus obtained are potential precursors for vinylogous carbanions such as 7a—c and/or 8a—c, we next examined their deprotonation. The reactions of 4a+5a+6a and 4b+5b+6b with potassium tert-butoxide in DMF at room temperature proceeded smoothly to give the corresponding products 11a,b as unstable yellow powders in 85% and 89% yields.8,9 These compounds were not diethyl 5-oxo-4,5- dihydrothiepino[2,3-b]indolizine-4,11-dicarboxylates (9a,b) which were initially expected from the nucleophilic attack of the carbanions 7a,b on the ester carbonyl group at the 3-position of the indolizine ring followed by the elimination of an ethoxide ion, but were diethyl 5-oxo-2,5-dihydrothiepino[2,3-b]indolizine-4,11-dicarboxylates (11a) and its 9-methyl derivatives 11b which were derived from the proton transfer of the cycloadducts 9a,b. Similar reaction of 4c+5c+6c provided diethyl 6,8-dimethyl-5-oxo-2,5-dihydrothiepino[3,2-a]indolizine-4,11-dicarboxylate (12c) in a 68% yield as an unstable yellow powder.8.9 In these reactions, however, the alternative 2-vinylthieno[2,3-b]- (9a,b) or 2-vinylthieno[3,2-a]indolizine derivative (10c) which can be formed via the intermediate 8a—c could not be obtained at all. These results are shown in Scheme 1.
The structural assignments for products 4a—c, 5a—c, 6a—c, 11a,b, and 12c were performed mainly by 1H-NMR spectral analyses. In particular, the structures of major S-alkylated indolizines 4a—c could readily be determined by the presence of the S-methylene triplet (J=7.6 Hz) at δ 3.63—3.75 ppm and of the vinyl protons with a trans-coupling constant (15.4 Hz) near δ 5.7 and 6.9 ppm respectively, together with the signals of three ethoxycarbonyl and indolizine ring protons. Similarly, the structures of minor products 5a—c and 6a—c were assigned as the E- and Z-isomers of the 2-[(3-ethoxycarbonyl-1- propenyl)thio] group by the appearances of the methylene triplet (J=6.8—7.2 Hz) at higher magnetic regions (δ 3.08 (trans) and 3.32 or 3.33 (cis) ppm) and of the S-vinyl protons with a trans- (15.2—15.4 Hz) or cis-coupling constant (9.4 —9.6 Hz) near δ 5.8 and 6.2 ppm. On the other hand, 1H-NMR spectra
of thiepino[2,3-b]- 11a,b and thiepino[3,2-a]indolizines 12c exhibited the presence of only two ethoxy groups and the loss of an ethoxy group at lower magnetic regions. This is assignable to the ethoxycarbonyl group at the 1- or 3-position of the indolizine ring. This fact strongly suggested that carbanion 7 or 8 interacted with the ethoxycarbonyl group at the 1- or 3-position with the elimination of an ethoxide ion during these reactions. The absence of the AB type signals due to the exocyclic vinyl group as shown in 2-vinylthienoindolizines 15a,b and 16c and the presence of the AX2 type signals (δ 3.39-3.43 (d, SCH2) and 7.07-9.19 ppm (t, 3-H)) with a vicinal coupling constant (7.3 Hz) supported the thiepin structure for 11a,b and 12c. Furthermore, the comparisons of the chemical shifts of the protons on the indolizine ring in 11a,b and 12c with those in S-alkylated indolizines 4a—c disclosed the orientation of these cyclizations. That is, the chemical shifts for each 7-proton in 11a,b were shifted to a higher magnetic region (ca. δ 0.5 ppm) in comparison with those of 4a,b, while the chemical shifts of each 10-proton in 11a,b and of the 9-proton in 12c were grossly similar to those in 4a—c. These facts indicate that the nucleophilic attacks of the anions on the 3-ester carbonyl carbon in 7a,b and on the 1-ester one in 7c occurred. The same orientation of the cyclization on the indolizine ring have already been observed in the transformation reactions from diethyl 2-(acylmethylthio)indolizine- 1,3-dicarboxylates to the corresponding 2-acyltheino[2,3-b]- and 2-acylthieno[3,2-a]indolizine derivatives, and the electronic and steric effects leading to such orientations have been also described.3 The reason for the exclusive formation of thiepino[2,3-b]- 11a,b and thiepino[3,2-a]indolizine 12c from the alkaline treatment of S-alkylated indolizines 4a—c, 5a—c, and 6a—c is unclear, but the larger contribution of the allyl anions 7a—c, which are stabilized by resonance and inductive effects, over the alternative anions 8a—c may be considered. Further investigation of this reaction is now in progress.
References
1. Preparation of new nitrogen-bridged heterocycles. 65. For part 64 of this series, see A. Kakehi, H. Suga, and S. Sato, Heterocycles, 2009, 77, 471. CrossRef
2. A. Kakehi, S. Ito, N. Yamada, and K. Yamaguchi, Chem. Pharm. Bull., 1990, 38, 1527.
3. A. Kakehi, S. Ito, T. Fujii, T. Ueda, and T. Hirata, Chem. Pharm. Bull., 1992, 40, 2313.
4. A. Kakehi, S. Ito, H. Suga, H. Takahashi, and K. Dobashi, Heterocycles, 2000, 52, 215. CrossRef
5. K. Marx and W. Eberbach, Tetrahedron, 1997, 53, 14687. CrossRef
6. 4a, 1H NMR (CDCl3) δ: 1.22, 1.46, and 1.47 (each 3H, t, J=7.1 or 7.2 Hz, 3xOCH2CH3), 3.75 (2H, dd, J=7.6, and 1,2 Hz, SCH2), 4.11, 4.44, and 4.47 (each 2H, q, J=7.1 or 7.2 Hz, 3xOCH2CH3), 5.68 (1H, dt, J=15.4, 1.3, and 1.3 Hz, =CHCO2Et), 6.91 (1H, dt, J=15.4, 7.6, and 7.6 Hz, SCH2CH=CH), 6.93 (1H, dt, J=7.1, 7.1, and 1.2 Hz, 6-H), 7.27 (1H, ddd, J=9.2, 6.8, and 1.1 Hz, 7-H), 8.26 (1H, dt, J=9.3, 1.1, and 1.1 Hz, 8-H), and 9.42 (1H, dt, J=7.1, 1.1, and 1.1 Hz, 5-H).
4b, 1H NMR (CDCl3) δ: 1.23, 1.46, and 1.47 (each 3H, t, J=7.1 Hz, 3xOCH2CH3), 2.43 (3H, d, J=1.0 Hz, 7-Me), 3.74 (2H, dd, J=7.6 and 1.2 Hz, SCH2), 4.12, 4.44, and 4.46 (each 2H, q, J=7.1 or 7.2 Hz, 3xOCH2CH3), 5.67 (1H, dt, J=15.4, 1.3, and 1.3 Hz, =CHCO2Et), 6.77 (1H, dd, J=7.3 and 1.9 Hz, 6-H), 6.91 (1H, dt, J=15.4, 7.6, and 7.6 Hz, SCH2CH=CH), 8.06 (1H, quint, J=1.0 Hz, 8-H), and 9.32 (1H, d, J=7.2 Hz, 5-H).
4c, 1H NMR (CDCl3) δ: 1.24, 1.42, and 1.47 (each 3H, t, J=7.1 Hz, 3xOCH2CH3), 2.30 (3H, s, 6-Me), 2.42 (3H, s, 8-Me), 3.63 (2H, dd, J=7.6 and 1.2 Hz, SCH2), 4.14, 4.42, and 4.45 (each 2H, q, J=7.2 Hz, 3xOCH2CH3), 5.66 (1H, dt, J=15.4, 1.2, and 1.2 Hz, =CHCO2Et), 6.80 (1H, br s, 7-Me), 6.93 (dt, J=15.4, 7.7, and 7.7 Hz, SCH2CH=CH), 9.19 (1H, br s, 5-H).
5a, 1H NMR (CDCl3) δ: 3.08 (2H, dd, J=7.2 and 1.3 Hz, CH2CO2Et), 5.80 (1H, dt, J=15.3, 7.2, and 7.2 Hz, SCH=CHCH2), and 6.20 (1H, dt, J=15.3, 1.4, and 1.4 Hz, SCH=CH).10
5b, 1H NMR (CDCl3) δ: 3.08 (2H, dd, J=7.2 and 1.3 Hz, CH2CO2Et), 5.79 (1H, dt, J=15.2, 7.2, and 7.2 Hz, SCH=CHCH2), and 6.20 (1H, dt, J=15.2, 1.3, and 1.3 Hz, SCH=CH).10
5c, 1H NMR (CDCl3) δ: 1.25, 1.39, and 1.41 (each 3H, t, J=7.1 or 7.2 Hz, 3xCO2CH2CH3), 3.08 (2H, dd, J=7.1 and 1.5 Hz, CH2CO2Et), 4.13 and 4.35 (each 2H, q, J=7.2 Hz, 2xCO2CH2CH3), 5.82 (1H, dt, J=15.3, 7.1, and 7.1 Hz, SCH=CHCH2), and 6.21 (1H, dt, J=15.4, 1.2, and 1.2 Hz, SCH=CH).10
6a, 1H NMR (CDCl3) δ: 3.33 (2H, dd, J=6.8 and 1.7 Hz, CH2CO2Et), 5.79 (1H, dt, J=9.5, 6.8, and 6.8 Hz, SCH=CHCH2), and 6.21 (1H, dt, J=9.5, 1.6, and 1.6 Hz, SCH=CH).10
6b, 1H NMR (CDCl3) δ: 3.33 (2H, dd, J=6.8 and 1.7 Hz, CH2CO2Et), 5.78 (1H, dt, J=9.6, 6.8, and 6.8 Hz, SCH=CHCH2), and 6.21 (1H, dt, J=9.5, 1.7, and 1.7 Hz, SCH=CH).10
6c, 1H NMR (CDCl3) δ: 1.29, 1.37, and 1.40 (each 3H, t, J=7.0 or 7.1 Hz, 3xCO2CH2CH3), 3.32 (2H, dd, J=6.9 and 1.7 Hz, CH2CO2Et), 4.18 and 4.34 (each 2H, q, J=7.2 Hz, 2xCO2CH2CH3), 5.82 (1H, dt, J=9.4, 6.8, and 6.8 Hz, SCH=CHCH2), and 6.26 (1H, dt, J=9.5, 1.6, and 1.6 Hz, SCH=CH).10.
7. The separation of these indolizine mixtures 4a+5a+6a, 4b+5b+6b, and 4c+5c+6c were unsuccessful, but they gave satisfactory elemental analyses.
4a+5a+6a (pale yellow oil). Anal. Calcd for C20H23NO6S: C, 59.25; H, 5.72; N, 3.45. Found: C, 59.41; H, 5.66; N, 3.35.
4b+5b+6b (pale yellow oil). Anal. Calcd for C21H25NO6S: C, 60.13; H, 6.01; N, 3.34. Found: C, 60.28; H, 5.98; N, 3.22.
4c+5c+6c (pale yellow oil). Anal. Calcd for C22H27NO6S: C, 60.95; H, 6.28; N, 3.23. Found: C, 60.95; H, 6.35; N, 3.16.
8. 11a: mp 134-137 °C, ν (KBr) cm-1 1698 and 1747. 1H NMR (CDCl3) δ: 1.31 and 1.44 (each 3H, t, J=7.1 or 7.2 Hz, 2xOCH2CH3), 3.43 (2H, d, J=7.3 Hz, 2-H), 4.22 and 4.40 (each 2H, q, J=7.1 or 7.2 Hz, 2xOCH2CH3), 7.04 (1H, dt, J=6.8, 1.2, and 1.2 Hz, 8-H), 7.19 (1H, t, J=7.3 Hz, 3-H), 7.46 (1H, ddd, J=9.1, 7.0, and 1.2 Hz, 9-H), 8.23 (1H, dt, J=9.0, 1.2, and 1.2 Hz, 10-H), 8.95 (1H, dt, J=6.7, 1.2, and 1.2 Hz, 7-H). 13C NMR (CDCl3) δ: 14.19, 14.55, 35.86, 60.42, 61.45, 100.90, 114.92, 119.24, 121.41, 127.24, 127.59, 128.96, 141.50, 143.79, 145.50, 162.99, 169.17, 171.78. HRMS m/z calcd for C18H18NO5S (M++H): 360.0900, found: 360.0898.
11b: mp 121-124 °C, ν (KBr) cm-1 1664, 1701, 1748. 1H NMR (CDCl3) δ: 1.30 and 1.43 (each 3H, t, J=7.1 or 7.2 Hz, 2xOCH2CH3), 2.48 (3H, s, 9-Me), 3.41 (2H, d, J=7.3 Hz, 2-H), 4.22 and 4.39 (each 2H, q, J=7.1 or 7.2 Hz, 2xOCH2CH3), 8.00 (1H, br s, 10-H), 8.79 (1H, d, J=6.8 Hz, 7-H). 13C NMR (CDCl3) δ: 14.19, 14.57, 21.93, 35.87, 60.32, 61.43, 99.94, 117.23, 118.21, 121.02, 126.63, 127.18, 141.19, 141.69, 144.26, 145.90, 163.15, 169.24, 171.32. HRMS m/z calcd for C19H20NO5S (M++H): 374.1057, found: 374.1025.
12c, mp 117-120 °C, ν (KBr) cm-1 1668, 1692, and 1736. 1H NMR (CDCl3) δ: 1.30 and 1.45 (each 3H, t, J=7.1 or 7.2 Hz, 2xOCH2CH3), 2.35 (3H, s, 8-Me), 2.89 (3H, s, 6-Me), 3.39 (2H, d, J=7.3 Hz, 2-H), 4.21 and 4.41 (each 2H, q, J=7.0 or 7.1 Hz, 2xOCH2CH3), 7.03 (1H, br s, 9-H), 7.07 (1H, t, J=7.3 Hz, 3-H), 9.19 (1H, br s, 9-H). 13C NMR (CDCl3) δ: 14.20, 14.55, 18.32, 20.00, 36.17, 60.64, 61.34, 107.99, 113.60, 124.69, 125.57, 125.82, 129.55, 131.54, 135.47, 142.45, 144.73, 160.39, 169.47, 178.12. HRMS m/z calcd for C20H22NO5S (M++H): 388.1232, found: 388.1191.
9. Because thiepinoindolizines 11a,b and 12c were considerably unstable, their compositions were confirmed by high resolution mass spectra.
10. Other proton signals were overlapped with those of major products 4a-c.