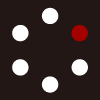
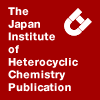
HETEROCYCLES
An International Journal for Reviews and Communications in Heterocyclic ChemistryWeb Edition ISSN: 1881-0942
Published online by The Japan Institute of Heterocyclic Chemistry
e-Journal
Full Text HTML
Received, 30th August, 2008, Accepted, 10th October, 2008, Published online, 14th October, 2008.
DOI: 10.3987/COM-08-S(F)108
■ Direct and Stereoselective Synthesis of 2-Azido-2-deoxy-β-D-mannosides Using the Phosphate Method
Seiichi Nakamura, Toshifumi Tsuda, Noritoshi Suzuki, and Shunichi Hashimoto*
Laboratory of Synthetic and Industrial Chemistry, Graduate School of Life Science, Division of Life Science, Faculty of Pharmaceutical Sciences, Hokkaido University, Kita 12, Nishi 6, Kita-ku, Sapporo, Hokkaido 060-0812, Japan
Abstract
TMSOTf-promoted glycosidation of 2-azido-3-O-benzyl-4,6-O-benzylidene-2-deoxy-α-D-mannosyl diphenyl phosphate with a variety of acceptor alcohols in CH2Cl2 at –30 °C in the presence of pulverized 4-Å molecular sieves (MS) afforded 2-azido-2-deoxy-β-mannosides in high yields and with good to high β-selectivities.The β-linked 2-acetamido-2-deoxy-D-mannosides are present in a number of bacterial capsular polysaccharides and lipopolysaccharides (LPS). Due to the potential utilization of capsular polysaccharides as immunogens in providing protection against bacterial infection1 and due to the overwhelming spectrum of biological activities of LPS, efforts have been devoted to the stereocontrolled construction of 2-acetamido-2-deoxy-β-D-mannosidic linkages.2 Although the first example reported by Paulsen and co-workers involved the use of 2-azido-2-deoxy-α-D-mannosyl bromide as a donor,3 most approaches to this type of glycoside rely on indirect methods. The 2-oximinoglycosyl donor approach4,5 and the SN2 inversion of readily available β-glucosides at C-2 with an azide nucleophile6 have been developed for this purpose, and these methods provide reliable access to pure 2-acetamido-2-deoxy-β-mannosides. However, it is clear that a direct β-glycosidation would constitute an ideal procedure in terms of efficiency and practicality. In this context, extension of the Crich’s β-selective mannosylation methodology7 to the synthesis of 2-azido-2-deoxy-β-mannosides was recently reported by van der Marel and co-workers.8 They demonstrated that the activator systems 1-(phenylsulfinyl)- piperidine/Tf2O and diphenyl sulfoxide/Tf2O are effective for the glycosidation of 4,6-O-benzylidene- protected 2-azido-2-deoxy-1-thiomannoside, producing 2-azido-2-deoxymannosides in good yields with good β-selectivities: however, the operational inconvenience8c—acceptor alcohols must be added after activation of the donor—as well as the formation of the leaving group-derived by-products seems to be less favorable from a practical standpoint.
As part of a program to extend the glycosidation method that capitalizes on phosphorus-containing leaving groups,9,10 we have reported that TMSOTf-promoted reaction of 2,3-di-O-benzyl-4,6-O- benzylidene- D-mannosyl diethyl phosphite with a broad variety of acceptor alcohols in CH2Cl2 at –45 °C offers a facile and high-yielding route to β-mannosides.11 As an extension of the mannosylation reaction, we addressed the direct construction of 2-azido-2-deoxy-β-mannosidic linkages. In our previous mannosylation studies, the use of diethyl phosphite as a leaving group proved to be optimal in terms of reactivity and product yield.11 However, the difficulty in preparation of 2-azido-2-deoxyglycosyl donors carrying a phosphite as a leaving group9i,12 prompted us to investigate the reaction of 2-azido-3-O-benzyl-4,6-O-benzylidene-2-deoxy-D-mannosyl diphenyl phosphates.
The diphenyl phosphate was prepared starting with the known compound 113 (Scheme 1). Deacetylation of triacetate 1 with NaOMe in MeOH was followed by reprotection of the C4 and C6 hydroxyl groups as their benzylidene acetal and that of the C3 hydroxyl group as its benzyl ether to give 1-O-TBS glycoside 2 in 72% yield in three steps. After desilylation of 2 with Bu4NF in the presence of AcOH, the resultant hemiacetal was phosphorylated under Sabesan conditions14 to give diphenyl phosphates 3 and 4 in 71% and 27% yields, respectively.
With donors 3 and 4 in hand, we then explored TMSOTf-promoted glycosidation with 6-O-unprotected glucoside 5. Addition of 1.5 equiv of TMSOTf to a mixture of the donor (3 or 4) and 1.1 equiv of acceptor 5 in CH2Cl2 afforded disaccharide 6, the α:β ratio of which was assayed by HPLC (Zorbax® Sil column).15,16 An initial experiment revealed that the reaction of α-phosphate 3 proceeded to completion within 2.5 h at –30 °C, providing disaccharide 6 in 65% yield with good β-selectivity (α:β = 18:82) (Table 1, entry 1). Since the modest product yield was attributed to the partial hydrolysis of the benzylidene acetal under acidic conditions, we expected that the side reaction could be suppressed by an additive that buffers the Lewis acidity of TMSOTf. Gratifyingly, pulverized 4-Å MS17 turned out to be suitable for this purpose, improving the chemical yield to 80% without affecting the β-selectivity (entry 2). It was anticipated that the same β-selectivity could be obtained by the use of β-phosphate 4 as a donor if the reaction proceeded through the intermediacy of the corresponding glycosyl triflate as demonstrated by Crich and co-workers for their β-selective mannosylation method.7c However, a slight erosion in β-selectivity (α:β = 18:82 → 24:76) was observed in the reaction of β-phosphate 4 with alcohol 5 (entry 3). This result suggests that the use of α-phosphate 3 is essential for obtaining higher β-selectivity, although the reason is not clear at present.
With the optimal conditions identified, the scope of the reaction was explored (Table 2). The glycosida¬tion of α-phosphate 3 with less reactive 4-O-unprotected glucoside 7 proceeded to completion within 4 h under these conditions, leading to the preferential formation of β-disaccharide 12β, which corresponds to a constituent of the capsular polysaccharide of Streptococcus pneumoniae type 19F (entry 1). The best β-selectivity (α:β = 8:92) was achieved by the glycosidation of the 2-O-unprotected glucoside 8 (entry 2). The galactose, mannose and L-rhamnose derivatives 9–11 could be uneventfully glycosylated under these conditions with good β-selectivities (entries 3–5).
In conclusion, we have demonstrated that TMSOTf-promoted glycosidation of 2-azido-3-O-benzyl-4,6-O-benzylidene-2-deoxy-α-D-mannosyl diphenyl phosphate (3) with a variety of acceptor alcohols in CH2Cl2 at –30 °C in the presence of pulverized 4-Å MS exhibits good to high β-selectivities. High product yields can be achieved with approximately equimolar proportions of the donor 3 and acceptor alcohols, and of particular note is a simple reaction protocol that does not need the pre-activation of the donor. Further investigation into the mechanism of this glycosidation is currently underway, and results will be reported in due course.
ACKNOWLEDGEMENTS
This research was supported in part by a Grant-in-Aid for Scientific Research from the Japan Society for the Promotion of Science (JSPS). We also acknowledge JSPS for graduate fellowships to T.T. We thank Ms. Hiroko Matsumoto, Ms. Akiko Maeda, Ms. Seiko Oka, and Ms. Miwa Kiuchi, and Mr. Tomohiro Hirose of the Center for Instrumental Analysis, Hokkaido University for technical assistance in mass and elemental analysis.
† Dedicated to Professor Emeritus Keiichiro Fukumoto on the occasion of his 75th birthday.
References
1. H. J. Jennings, Adv. Carbohydr. Chem. Biochem., 1983, 41, 155. CrossRef
2. For a review, see: J. J. Gridley and H. M. I. Osborn, J. Chem. Soc., Perkin Trans. 1, 2000, 1471. CrossRef
3. (a) H. Paulsen and J. P. Lorentzen, Carbohydr. Res., 1984, 133, C1; CrossRef (b) H. Paulsen, J. P. Lorentzen, and W. Kutschker, Carbohydr. Res., 1985, 136, 153. CrossRef
4. (a) F. W. Lichtenthaler, E. Kaji, and S. Weprek, J. Org. Chem., 1985, 50, 3505; CrossRef (b) F. W. Lichtenthaler and E. Kaji, Liebigs Ann. Chem., 1985, 1659; CrossRef (c) E. Kaji, F. W. Lichtenthaler, T. Nishino, A. Yamane, and S. Zen, Bull. Chem. Soc. Jpn., 1988, 61, 1291; CrossRef (d) E. Kaji, F. W. Lichtenthaler, Y. Osa, K. Takahashi, and S. Zen, Bull. Chem. Soc. Jpn., 1995, 68, 2401; CrossRef (e) S. C. Ennis, J. J. Gridley, H. M. I. Osborn, and D. G. Spackman, Synlett, 2000, 1593; CrossRef (f) M. G. B. Drew, S. C. Ennis, J. J. Gridley, H. M. I. Osborn, and D. G. Spackman, Tetrahedron, 2001, 57, 7919. CrossRef
5. For a review, see: E. Kaji and F. W. Lichtenthaler, Trends Glycosci. Glycotechnol., 1993, 5, 121.
6. (a) K. Sato and A. Yoshitomo, Chem. Lett., 1995, 39; CrossRef (b) K. Sato, A. Yoshitomo, and Y. Takai, Bull. Chem. Soc. Jpn., 1997, 70, 885; CrossRef (c) M. Nilsson and T. Norberg, J. Chem. Soc., Perkin Trans. 1, 1998, 1699; CrossRef (d) E. Bousquet, M. Khitri, L. Lay, F. Nicotra, L. Panza, and G. Russo, Carbohydr. Res., 1998, 311, 171; CrossRef (e) S. Koto, Y. Shinoda, M. Hirooka, A. Sekino, S. Ishizumi, M. Koma, C. Matuura, and N. Sakata, Bull. Chem. Soc. Jpn., 2003, 76, 1603. CrossRef
7. (a) D. Crich and S. Sun, J. Org. Chem., 1996, 61, 4506; CrossRef (b) D. Crich and S. Sun, J. Org. Chem., 1997, 62, 1198; CrossRef (c) D. Crich and S. Sun, J. Am. Chem. Soc., 1997, 119, 11217; CrossRef (d) D. Crich and S. Sun, J. Am. Chem. Soc., 1998, 120, 435; CrossRef (e) D. Crich and S. Sun, Tetrahedron, 1998, 54, 8321; CrossRef (f) D. Crich and N. S. Chandrasekera, Angew. Chem. Int. Ed., 2004, 43, 5386. CrossRef
8. (a) R. E. J. N. Litjens, M. A. Leeuwenburgh, G. A. van der Marel, and J. H. van Boom, Tetrahedron Lett., 2001, 42, 8693; CrossRef (b) J. D. C. Codée, R. E. J. N. Litjens, R. den Heeten, H. S. Overkleeft, J. H. van Boom, and G. A. van der Marel, Org. Lett., 2003, 5, 1519; CrossRef (c) J. D. C. Codée, L. J. van den Bos, R. E. J. N. Litjens, H. S. Overkleeft, C. A. A. van Boeckel, J. H. van Boom, and G. A. van der Marel, Tetrahedron, 2004, 60, 1057; CrossRef (d) R. E. J. N. Litjens, R. den Heeten, M. S. M. Timmer, H. S. Overkleeft, and G. A. van der Marel, Chem. Eur. J., 2005, 11, 1010; CrossRef (e) R. E. J. N. Litjens, L. J. van den Bos, J. D. C. Codée, R. J. B. H. N. van den Berg, H. S. Overkleeft, and G. A. van der Marel, Eur. J. Org. Chem., 2005, 918; CrossRef (f) L. J. van den Bos, B. A. Duivenvoorden, M. C. de Koning, D. V. Filippov, H. S. Overkleeft, and G. A. van der Marel, Eur. J. Org. Chem., 2007, 116. CrossRef
9. (a) S. Hashimoto, T. Honda, and S. Ikegami, J. Chem. Soc., Chem. Commun., 1989, 685; CrossRef (b) S. Hashimoto, T. Honda, and S. Ikegami, Heterocycles, 1990, 30, 775; CrossRef (c) S. Hashimoto, T. Honda, and S. Ikegami, Tetrahedron Lett., 1990, 31, 4769; CrossRef (d) S. Hashimoto, Y. Yanagiya, T. Honda, H. Harada, and S. Ikegami, Tetrahedron Lett., 1992, 33, 3523; CrossRef (e) S. Hashimoto, K. Umeo, A. Sano, N. Watanabe, M. Nakajima, and S. Ikegami, Tetrahedron Lett., 1995, 36, 2251; CrossRef (f) S. Hashimoto, A. Sano, H. Sakamoto, M. Nakajima, Y. Yanagiya, and S. Ikegami, Synlett, 1995, 1271; CrossRef (g) H. Tanaka, H. Sakamoto, A. Sano, S. Nakamura, M. Nakajima, and S. Hashimoto, Chem. Commun., 1999, 1259; CrossRef (h) T. Tsuda, S. Nakamura, and S. Hashimoto, Tetrahedron Lett., 2003, 44, 6453; CrossRef (i) T. Tsuda, S. Nakamura, and S. Hashimoto, Tetrahedron, 2004, 60, 10711; CrossRef (j) R. Arihara, S. Nakamura, and S. Hashimoto, Angew. Chem. Int. Ed., 2005, 44, 2245; CrossRef (k) M. Koshiba, N. Suzuki, R. Arihara, T. Tsuda, H. Nambu, S. Nakamura, and S. Hashimoto, Chem. Asian J., 2008, 3, 1664. CrossRef
10. For a review on glycosidations of glycosyl phosphates, phosphites and other O-P derivatives, see: S. Nakamura, H. Nambu, and S. Hashimoto, ‘Handbook of Chemical Glycosylation: Advances in Stereoselectivity and Therapeutic Relevance,’ ed. by A. V. Demchenko, Wiley-VCH, Weinheim, 2008, pp. 223-259.
11. (a) T. Tsuda, S. Sato, S. Nakamura, and S. Hashimoto, Heterocycles, 2003, 59, 509; CrossRef (b) T. Tsuda, R. Arihara, S. Sato, M. Koshiba, S. Nakamura, and S. Hashimoto, Tetrahedron, 2005, 61, 10719. CrossRef
12. C.-C. Lin, M. Shimazaki, M.-P. Heck, S. Aoki, R. Wang, T. Kimura, H. Ritzèn, S. Takayama, S.-H. Wu, G. Weitz-Schmidt, and C.-H. Wong, J. Am. Chem. Soc., 1996, 118, 6826. CrossRef
13. W. Kinzy and R. R. Schmidt, Liebigs Ann. Chem., 1985, 1537. Schmidt and Kinzy illustrated in their article that 1-O-TBS glycoside with an α-configuration at C-1 was obtained by silylation of the corresponding hemiacetal. However, the 1H NOE interactions of H-1 with H-2 (9.0%), H-3 (6.0%) and H-5 (13.1%) unambiguously established the β-configuration of 1-O-TBS glycoside 2. CrossRef
14. S. Sabesan and S. Neira, Carbohydr. Res., 1992, 223, 169. CrossRef
15. A typical experimental procedure is illustrated as follows (Table 1, entry 2): TMSOTf in CH2Cl2 (1.0 M, 0.15 mL, 0.15 mmol) was added to a stirred mixture of diphenyl phosphate 3 (61.6 mg, 0.10 mmol), alcohol 5 (51.1 mg, 0.11 mmol) and pulverized 4-Å MS (80 mg) in CH2Cl2 (1 mL) at -30 °C. After stirring for 2.5 h, the reaction was quenched with Et3N (0.1 mL). The mixture was poured into a two-layer mixture of AcOEt (2 mL) and saturated aqueous NaHCO3 (3 mL), and the whole mixture was extracted with AcOEt (20 mL). The organic extract was successively washed with saturated aqueous NaHCO3 (5 mL) and brine (2 x 5 mL), and dried over anhydrous Na2SO4. Filtration and evaporation in vacuo furnished the crude product (104.9 mg), which was purified by flash column chromatography (silica gel 5 g, 5:1 n-hexane/AcOEt) to give disaccharide 6 (66.0 mg, 80%, α:β = 18:82) as a white solid. The α- and β-glycosides were separated by flash column chromatography with 5:1 n-hexane/AcOEt for characterization purposes.
16. The stereochemical assignments were verified by 1H NOE correlations between H-1’ and H-5’ of the β-anomers.
17. 4-Å MS pellets, purchased from Junsei Chemical Co., Ltd., were finely ground in mortar and activated by heating in vacuo at 220 °C for 12 h