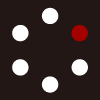
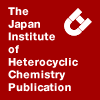
HETEROCYCLES
An International Journal for Reviews and Communications in Heterocyclic ChemistryWeb Edition ISSN: 1881-0942
Published online by The Japan Institute of Heterocyclic Chemistry
e-Journal
Full Text HTML
Received, 2nd September, 2008, Accepted, 15th October, 2008, Published online, 16th October, 2008.
DOI: 10.3987/COM-08-S(D)16
■ Synthesis, Determination of the Absolute Stereochemistry, and Evaluations at the Nicotinic Acetylcholine Receptors of a Hydroxyindolizidine Alkaloid from the Ant Myrmicaria melanogaster
Dejun Zhou, Naoki Toyooka,* Hideo Nemoto, Kaoru Yamaguchi, Hiroshi Tsuneki, Tsutomu Wada, Toshiyasu Sasaoka, Hideki Sakai, Yasuhiro Tezuka, Shigetoshi Kadota, Tappey H. Jones, H. Martin Garraffo, Thomas F. Spande, and John W. Daly
Faculty of Pharmaceutical Sciences, Toyama University, 2630 Sugitani, Toyama, Toyama 930-0194, Japan
Abstract
The first chiral synthesis of new hydroxyindolizidine alkaloid (1) detected in the ant Myrmicaria melanogaster has been achieved, and its absolute stereochemistry was determined to be 3S, 5R, 8S, 9S by the present chiral synthesis.INTRODUCTION
The indolizidine skeleton has been found in several natural sources such as skin extracts of poison-frogs,1 venoms of ants,2 and several plants,3 and many of these indolizidines showed interesting biological activities.4 Consequently, considerable effort has been invested in the development of innovative methods for preparing this ring system in a highly stereocontrolled manner.5 Recently, Jones’ group reported the detection of five new alkaloids from an extract of the ant Myrmicaria melanogaster from Brunei in the Indonesian archipelago.6 Among them, the structure of the hydroxyindolizidine (1) which was proved using a non-stereoselective synthesis.6 However, the absolute stereochemistry of 1 remained unknown. Here we report a full account of the first chiral synthesis of 1 and the determination of its absolute stereochemistry.7 Biological evaluations of 1 and its C-8-epimer (8) at neuronal nicotinic acetylcholine receptors is also presented.
RESULTS AND DISCUSSION
The synthesis began with known lactam 2,8 which was converted to imide 3 in good yield. Treatment of 3 with n-BuMgBr in the presence of TMEDA at -78 °C provided the acyclic ketone 4 in 65% yield, which was subjected to Martin’s protocol9 to afford the 2,5-cis-disubstituted pyrrolidine 5 as the sole product. Half-reduction of the ester moiety in 5 with DIBAL followed by treatment of the resulting aldehyde with excess vinylMgBr (3 eq) gave rise to a mixture of corresponding alcohols 6. Expansion of the side chain at 2-position by the cross-metathesis reaction using the Grubbs’ second-generation catalyst10 afforded a mixture of unsaturated ketones 7. Finally, hydrogenation of 7 using Pearlman’s catalyst provided 1 and its 8-epimer 8 in 62% and 19% isolated yield, respectively. The stereochemistry of the major isomer 1 was determined by the NOE experiments as shown in Scheme 1.
The stereoselectivity of the addition of the vinylMgBr to the aldehyde derived from 5 was explained by the chelation-control model as shown in Figure 1. Both synthtic (-)-1 and the natural product coeluted on GC and had identical mass spectra. Synthetic (-)-1 had also same retention time and mass spectrum as the first eluting isomer of (±)-1. The absolute stereochemistry of the natural product was determined by the comparison of synthetic (-)-1, (±)-1 synthesized by Jones,6 and natural product on the GC analysis using a chiral permethylated β-cyclodextrin GC column. The natural product and synthetic (-)-1 each coeluted with the slightly more slowly eluting enantiomer of (±)-1 (156.5 °C), whereas the (+)-1 eluted at 156 °C. Thus, the absolute stereochemistry of natural product was determined to be 3S, 5R, 8S, 9S by the present chiral synthesis.
When the oocytes expressing α4β2 nicotinic receptors were treated with synthetic (-)-1 (10 μM), the peak amplitude of currents elicited by ACh (1 μM) was greatly decreased (Figure 2A). In fact, the ACh-elicited currents were blocked by this alkaloid in a concentration-dependent manner (Figure 2B) with 50% inhibitory concentration (IC50) values of 9.5 μM [95% confidence intervals (CI) = 6.3-14.4 μM, number of determinations (N) = 3]. Similarly, the 8-epimer 8 (1-30 μM) inhibited α4β2 currents in a concentration-dependent manner with IC50 values of 7.9 μM (95% CI = 3.3-19.3 μM, N=3) (data not shown). The blocking effects of (-)-1 and 8 at 10 μM on α4β2 nicotinic receptors were reversible within 3 min (data not shown). These results demonstrate that the antagonist activity of (-)-1 on α4β2 nicotinic receptors was comparable with that of 8.
CONCLUSION
In summary, we have achieved the first chiral synthesis of new hydroxyindolizidine 1, isolated from ant Myrmicaria melanogaster, and the absolute stereochemistry of natural alkaloid was determined by the present chiral synthesis. Both (-)-1 and its 8-epimer 8 showed inhibitory effect on α4β2 nicotinic receptors with IC50 values of 9.5 μM and 7.9 μM, respectively.
EXPERIMENTAL
General Melting points are uncorrected. NMR spectra were recorded at 500 or 300 MHz (1H), 75 MHz (13C) using CDCl3 as the solvent. Chemical shifts are given in parts per million (ppm, δ) downfield from TMS and referenced to CHCl3 (7.26 ppm) as an internal standard. Mass spectra were obtained by EI mode. Column chromatography was performed on Kanto Kagaku silica gel 60N.
(2S)-5-Oxopyrrolidine-1,2-dicarboxylic acid 1-benzyl ester 2-methyl ester (3)
To a stirred solution of 28 (9.7 g, 67.83 mmol) in THF (80 mL) was added a solution of LiHMDS, prepared from 1,1,1,3,3,3-hexamethyldisilazane (15.56 mL, 74.6 mmol) and n-BuLi (1.6 M in hexane, 46.7 mL, 74.6 mmol) in THF (20 mL) at 0 oC for 30 min., at -78 oC, and the reaction mixture was stirred at the same temperature for 30 min. To the reaction mixture was added ClCO2Bn (10.98 mL, 74.6 mmol) -78 oC, and the reaction mixture was stirred at -78 oC ~ 0 oC for 1 h, then the reaction was quenched with satd. aqueous NaHCO3 (30 mL), and the aqueous mixture was extracted with CH2Cl2 (50 mL × 3). The organic extracts were combined, dried over MgSO4, and evaporated to give a pale yellow oil, which was chromatographed on silica gel (hexane : acetone = 10:1 ~ 3:1) to afford 3 (17.29 g, 92%) as a colorless oil. The spectral data were identical with those for reported values.11
(2S)-2-Benzyloxycarbonylamino-5-oxononanoic acid methyl ester (4)
To a stirred solution of 3 (5.63 g, 20.32 mmol) in THF (80 mL) at -78 oC was added a solution of n-BuMgBr, prepared from n-butyl bromide (6.54 mL, 60.96 mmol) and magnesium turnings (1.46 g, 60.96 mmol) in THF (80 mL) at reflux, and TMEDA (9.2 mL, 60.96 mmol), and then the reaction mixture was stirred for 1.5 h at -78 oC. The reaction was quenched with i-PrOH (7 mL) and 10% HCl (60 mL), and the aqueous mixture was extracted with Et2O (60 mL x 4). The organic extracts were combined, dried over MgSO4, and evaporated to give a pale yellow oil, which was chromatographed on silica gel (hexane : acetone = 10:1 ~ 5:1) to afford 4 (4.43 g, 65%) as a colorless solid (mp 38-40 oC).
IR (KBr): 3345, 3033, 2956, 2363, 1805, 1715, 1521, 1456, 1214, 1092, 1049, 740, 698cm-1; 1H NMR (300 MHz) δ 0.88 (3H, t, J = 7.2 Hz), 1.28 (2H, sext-like, J = 7.2 Hz), 1.52 (2H, quint-like, J = 7.2Hz), 1.91 (1H, sext-like, J = 7.2 Hz), 2.11-2.17 (1H, m), 2.37 (2H, t-like, 7.2 Hz), 2.40-2.56 (2H, m), 3.73 (3H, s), 4.34 (1H, m), 5.09 (2H, ABq, J=12.4 Hz), 5.43 (1H, br d, J = 7.3Hz), 7.37-7.30 (5H, m); 13C NMR (75 MHz) δ 13.51 (q), 21.94 (t), 25.48 (t), 25.79 (t), 37.96 (t), 42.17 (t), 52.06 (q), 53.10 (d), 66.56 (t), 127.72 (d), 127.79 (d), 128.15 (d), 136.00 (s), 155.81 (s), 172.34 (s), 209.60 (s); MS 335 (M+), 232(100). HRMS Calcd for C18H25NO5 335.1731, Found 335.1739; [α]D26 +4.37 (c 1.26, CHCl3).
(2S, 5S)-5-Butylpyrrolidine-1,2-dicarboxylic acid 1-benzyl ester 2-methyl ester (5)
BF3•Et2O (2.74 mL, 21.48 mmol) was added to a solution of Ph3SiH (2.8 g, 10.74 mmol) in CH2Cl2 (15 mL) at rt. The solution was stirred for 10 min at the same temperature, and then transferred via a cannula to a stirred solution of 4 (1.8 g, 5.37 mmol) in CH2Cl2 (20 mL) at -78 °C. The reaction mixture was stirred at -78 °C for 30 min, and then at rt for 2 h. The reaction mixture was poured into a mixture of satd. aqueous NaHCO3 (20 mL) at 0 °C. The organic layer was separated, and the aqueous layer was extracted with Et2O (3 × 30 mL). The organic layer and extracts were combined, dried over MgSO4, and evaporated to give a pale yellow oil, which was chromatographed on silica gel (hexane : acetone = 40:1 ~ 20:1) to afford 5 (1.7 g, 99%) as a colorless oil.
IR (neat): 3049, 2954, 2872, 1753, 1705, 1458, 1411, 1353, 1174, 1106, 1016, 874, 752, 699 cm-1; 1H NMR (300 MHz) δ 0.86 & 0.91 (3H, each t, J = 7.2 Hz), 1.28-1.44 (6H, m), 1.69-1.77 (1H, m), 1.94-2.05 (2H, m), 2.17-2.24 (1H, m), 3.61 & 3.75 (3H, each S), 3.89 & 3.95 (1H, each br s), 4.34 & 4.39 (1H, each t, J = 7.2 Hz), 5.15 (2H, ABq, J = 11.9 Hz), 7.34-7.47 (5H, m); 13C NMR (75 MHz) δ 13.79 & 13.90 (each q), 22.32 & 22.48 (each t), 27.94 & 28.38 (each t), 28.84 & 29.09 (each t), 29.84 (t), 33.36 & 33.95 (each t), 51.75 & 51.91 (each q), 58.46 & 59.14 (each d), 59.44 & 59.77 (each d), 66.56 & 66. 84 (each t), 127.41 & 127.51 (each d), 127.60 & 127.66 (each d), 128.13 & 129.54 (each d), 134.80 (d), 135.71 & 136.43 (each s), 153.98 & 154.83 (each s) 173.16 & 173.33 (each s); MS 319 (M+), 260 (100); HRMS Calcd for C18H25NO4 319.1782, Found 319.1776; [α]D26 -20.69 (c 1.26, CHCl3).
2-Butyl-5-(1-hydroxyallyl)pyrrolidine-1-carboxylic acid benzyl ester (6)
To a stirred solution of 5 (478.5 mg, 1.5 mmol) in CH2Cl2 (20 mL) was added a solution of DIBAL (1 M in n-hexane, 1.65 mL, 1.65 mmol) at -78 °C, and the reaction mixture was stirred at the same temperature for 30 min. The reaction was quenched with MeOH and satd. aqueous Rochelle salt, and the aqueous mixture was extracted with CH2Cl2 (15 mL x 4). The organic extracts were combined, dried over MgSO4, and evaporated to give a pale yellow oil which was used directly in the next step. To a stirred solution of the aldehyde obtained above in THF (5 mL) was added a solution of vinylmagnesium bromide (1.0 M in THF, 4.5 mL, 4.5 mmol) at -78 °C, then the reaction mixture was warmed to rt gradually for 16 h with stirring. The reaction was quenched with satd. aqueous NH4Cl (15 mL), and the aqueous mixture was extracted with CH2Cl2 (20 mL × 3). The organic extracts were combined, dried over MgSO4, and evaporated to give a pale yellow oil, that was chromatographed on silica gel (hexane : acetone = 25:1 ~ 15:1) to afford the carbinol mixture 6 (342 mg, 72%) as a colorless oil.
1H NMR (300 MHz) δ 0.85-0.90 (3H, br m) 1.25-1.36 (5H, br m), 1.63-1.96 (5H, br m), 3.89-4.37 (2H, br m), 4.91 (1H, br s), 5.10-5.35 (4H, m), 5.76-5.84 (1H, m), 7.30-7.39 (5H, m).
2-Butyl-5-(1-hydroxy-4-oxohept-2-enylpyrrolidine-1-carboxylic acid benzyl ester (7)
To a solution of 6 (180 mg, 0.568 mmol) in CH2Cl2 (10 mL) were added 1-hexen-3-one (0.37 mL, 2.84 mmol) and Grubbs’ second catalyst (48 mg, 0.057 mmol), and the resulting mixture was refluxed for 15 h. After cooling, the solvent was evaporated, and the residue was chromatographed on silica gel (hexane : acetone = 30:1 ~ 5:1) to afford a mixture of the carbinols 7 (212 mg, 97%) as a pale brown oil.
1H NMR (300 MHz) δ 0.83-0.96 (6H, m), 1.24 (5H, br s), 1.56-2.17 (7H, m), 2.42-2.59 (2H, m), 3.88-4.18 (3H, br m), 4.43 (1H, br s), 5.04-5.20 (2H, m), 5.56 (1H, br s), 6.33-6.47 (1H, br m), 6.68-6.77 (1H, m), 7.32-7.36 (5H, m).
(3S, 5R,8S, 9S)-3-Butyl-5-propyloctahydroindolizin-8-ol (1) and (3S, 5R, 8R, 9S)-3-Butyl-5-propyl- octahydroindolizin-8-ol (8)
To a stirred solution of 7 (58.1 mg, 0.162 mmol) in EtOH (7 mL) was added 20% Pd(OH)2/C (10 mg), and the resulting suspension was hydrogenated at 1 atm for 48 h. The catalyst was removed by filtration and the filtrate was evaporated to give a pale yellow oil, that was chromatographed on silica gel (hexane : acetone = 10:1 ~ 3:1) to yield 1 (21.2 mg, 62 %) as a colorless oil and 8 (6.5 mg, 19 %) as a colorless solid, respectively.
(-)-1: IR (neat) 3482, 2956, 2872, 1513, 1457, 1378, 1234, 1130, 1054, 970, 826 cm-1; 1H NMR (500 MHz, CDCl3) δ 0.90 (3H, t, J = 7.2 Hz), 0.91 (3H, t, J = 7.2 Hz), 1.17-1.49 (11H, br m), 1.53-1.62 (4H, m), 1.68-1.86 (3H, m), 2.25 (1H, t-like, J = 9.8 Hz), 2.40 (1H, m), 2.75 (1H, t-like, J = 8.5 Hz), 3.03 (1H, d, J = 10.3 Hz), 3.74 (1H, d, J = 9.8 Hz); 13C NMR (75 MHz, CDCl3) δ 14.27 (q), 14.50 (q), 19.16 (t), 22.98 (t), 25.92 (t), 26.67 (t), 28.75 (t), 28.98 (t), 32.18 (t), 37.83 (t), 39.43 (t), 60.43 (d), 64.07 (d), 65.45 (d), 70.06 (d); MS 239 (M+), 196 (100); HRMS Calcd for C15H29ON 239.2103, Found 239.2121; [α]D26 -48.92 (c 0.62, CHCl3).
8: mp 39~40 °C; IR (KBr) 3343, 2955, 2933, 2859, 2784, 1466, 1378, 1259, 1207, 1194, 1158, 1123, 1062, 1019 cm-1; 1H NMR (500 MHz) δ 0.89 (3H, t, J = 7.3 Hz), 0.91 (3H, t, J = 7.3 Hz), 1.18-1.36 (8H, m), 1.42 (1H, m), 1.45-1.64 (4H, m), 1.80-1.84 (2H, m), 1.92-2.08 (3H, m), 2.15 (1H, br), 2.66 (1H, t-like, J = 8.5 Hz), 3.43 (1H, br); 13C NMR (75 MHz) δ 14.24 (q), 14.53 (q), 19.55 (t), 22.88 (t), 27.91 (t), 29.22 (t), 29.68 (t), 30.87 (t), 34.08 (t), 37.51 (t), 39.37 (t), 62.10 (d), 63.75 (d), 72.87 (d), 73.05 (d); MS 239 (M+), 182 (100); HRMS Calcd for C15H29ON 239.2103, Found 239.2127 ; [α]D26 -53.55 (c 1.05, CHCl3).
ACKNOWLEDGEMENTS
This work was supported in part by a Grant-in-Aid for Scientific Research from the Japan Society for the Promotion of Science and Tamura Foundation for Promotion of Science and Technology.
References
1. J. W. Daly, T. F. Spande, and H. M. Garraffo, J. Nat. Prod., 2005, 68, 1556; CrossRef J. W. Daly, H. M. Garraffo, and T. F. Spande, In Alkaloids: Chemical and Biological Perspectives, ed. by S. W. Pelletier, Pergamon Press: New York, 1999, Vol. 13, pp. 1-161.
2. W. Francke, F. Schroder, F. Walter, V. Sinnwell, H. Baumann, and M. Kaib, Liebigs Ann., 1995, 965; CrossRef F. Schroder, V. Sinnwell, H. Baumann, and M. Kaib, Chem. Commun., 1996, 2139; CrossRef F. Schroder, S. Francke, W. Francke, H. Baumann, M. Kaib, J. M. Pasteels, and D. Daloze, Tetrahedron, 1996, 52, 13539; CrossRef F. Schroder, V. Sinnwell, H. Baumann, M. Kaib, and W. Francke, Angew. Chem., Int. Ed. Engl., 1997, 36, 77. CrossRef
3. R. C. Barbosa, F. Riet-Correa, R. M. T. Medeiros, E. F. Lima, S. S. Barros, E. J. Gimeno, R. J. Molyneux, and D. R. Gardner, Toxicon, 2006, 47, 371. CrossRef
4. N. Toyooka, D. Zhou, S. Kobayashi, H. Tsuneki, T. Wada, H. Sakai, H. Nemoto, T. Sasaoka, Y. Tezuka, Subehan, S. Kadota, H. M. Garraffo, T. F. Spande, and J. W. Daly, Synlett, 2008, 61; CrossRef N. Toyooka, S. Kobayashi, D. Zhou, H. Tsuneki, T. Wada, H. Sakai, H. Nemoto, T. Sasaoka, H. M. Garraffo, T. F. Spande, and J. W. Daly, Bioorg. Med. Chem. Lett., 2007, 17, 5872; CrossRef S. Kobayashi, N. Toyooka, D. Zhou, H. Tsuneki, T. Wada, T. Sasaoka, H. Sakai, H. Nemoto, H. M. Garraffo, T. F. Spande, and J. W. Daly, Beilstein J. Org. Chem., 2007, 3, 30; CrossRef H. Tsuneki, Y. You, N. Toyooka, S. Kagawa, S. Kobayashi, T. Sasaoka, H. Nemoto, I. Kimura, and J. A. Dani, Mol. Pharmacol., 2004, 66, 1061. CrossRef
5. J. P. Michael, Beilstein J. Org. Chem., 2007, 3, 27; CrossRef J. P. Michael, Nat. Prod. Rep., 2007, 24, 191. CrossRef
6. T. H. Jones, H. L. Voegtle, H. M. Miras, R. G. Weatherford, T. F. Spande, H. M. Garraffo, J. W. Daly, D. W. Davidson, and R. R. Snelling, J. Nat. Prod., 2007, 70, 160. CrossRef
7. N. Toyooka, D. Zhou, H. Nemoto, Y. Tezuka, S. Kadota, T. H. Jones, H. M. Garraffo, T. F. Spande, and J. W. Daly, Synlett, 2008, 1894. CrossRef
8. K. Drauz, A. Kleemann, J. Martens, P. Scherberich, and F. Effenberger, J. Org. Chem., 1986, 51, 3494. CrossRef
9. J. B. Brenneman, R. Machauer, and S. F. Martin, Tetrahedron, 2004, 60, 7301. CrossRef
10. P. Schwab, M. B. France, J. W. Ziller, and R. H. Grubbs, Angew. Chem. Int. Ed., Engl., 1995, 34, 2039. CrossRef
11. Y. S. Lee, D. J. Cho, S. N. Kim, J. H. Chio, and P. Hokoon, J. Org. Chem., 1999, 64, 9727. CrossRef