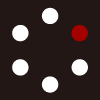
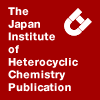
HETEROCYCLES
An International Journal for Reviews and Communications in Heterocyclic ChemistryWeb Edition ISSN: 1881-0942
Published online by The Japan Institute of Heterocyclic Chemistry
e-Journal
Full Text HTML
Received, 3rd September, 2008, Accepted, 4th November, 2008, Published online, 5th November, 2008.
DOI: 10.3987/REV-08-642
■ Development of Fluorescent 2-Pyrone Derivatives Using Ketene Dithioacetals for Organic EL Devices
Masayori Hagimori, Naoko Mizuyama, Yasuhiro Shigemitsu, Bo-Cheng Wang, and Yoshinori Tominaga*
School of Pharmaceutical Sciences, Nagasaki University, 1-14, Bunkyo-machi, Nagasaki 852-8521, Japan
Abstract
In recent years, organic electro luminescent (EL) materials have received considerable attention due to their potential application in next-generation display devices with high brightness levels, wide color ranges, and wide viewing angles. Fluorescent 2-pyrone derivatives have been synthesized using a convenient method involving ketene dithioacetals, which emit fluorescence (red, green, and blue) in both solution (dichloromethane and ethanol) and solid state. The structure-activity relationships of various 2-pyrone derivatives have been clarified.1. INTRODUCTION
Pigment chemistry such as that of mauveine pigments from the United Kingdom and indigo pigments from Germany has contributed to modern chemistry.1–4 Many heterocycles are examples of pigment compounds. The design of pigment compounds has become easier with the development of computational chemistry; however, not all compounds can be designed theoretically since practical and experimental pigment compounds possess large molecules.5,6 Since pigment chemistry obviously involves the π electron system, it is important that the chemical structure should include a functional group such as a chromophore or auxochrome. In particular, utilizing the properties of hetero atoms such as nitrogen, oxygen, and sulfur is critical in the design of pigments with various functionalities.2
Recently, the properties of pigment compounds have been used to create materials with new functions, including information storage, display of information, and energy conversion, for devices that act in response to light, heat, pressure, and electric fields.7–11 4-(Dicyanomethylene)-2-methyl-6-(p-dimethylaminostyryl)-4H-pyran (DCM), a red laser dye, has the capability of enhancing luminous efficiency in electro luminescence (EL) and controlling the light spectrum by expressing the property of a small amount of dopant molecule that exhibits a high fluorescence quantum yield.12 From the synthesis of heterocycles using ketene dithioacetals, we found that fluorescent 2-pyrone derivatives had remarkably similar properties to those of DCM. These compounds developed our interest in the organic EL materials. Herein, we describe our studies on the synthesis of organic EL materials expected to be used as next-generation display devices that will replace the widely used liquid crystal displays.
2. SYNTHESIS OF 4-METHYLSULFANYL-2-PYRONE DERIVATIVES
Ketene dithioacetals are readily prepared by the condensation of active methylene compounds with carbon disulfide in the presence of a base, followed by alkylation with suitable alkylating reagents. They are very important electrophilic reagents used in the synthesis of heterocycles having various substituents.
Since 1962, research on the use of this reagent in the synthesis of heterocyclic compounds has been greatly advanced by R. Gompper et al. in Germany and our group. As shown in Figure 1, these reactions proceed via an addition-elimination mechanism, which is initiated by a nucleophilic addition at the sp2 sulfur-substituted carbon atom of ketene dithioacetal, followed by an elimination of the methylsulfanyl group to complete the substitution reaction. Further, the same substitution reaction occurs at the other methylsulfanyl group and produces various derivatives. Therefore, ketene dithioacetals enable the introduction of various functional groups. In particular, chromophores and auxochromes can be introduced into the pigment molecules.13–19
On the basis of the initial research on ketene dithioacetals, it has been suggested that their skeletons are significant in the construction of dye molecules such as merocyanine dyes and the styryl series. We began our studies on the synthesis of materials for information storage with absorption in the near infrared and luminol type chemical luminescence reagents as high-sensitive analytical reagents of enzymes and reactive oxygen. This research finding triggered the development of fluorescence 2H-pyrone derivatives.20–26
The arrangement of suitable chromophores and auxochromes within the molecules both in the solution and solid states is necessary to confer fluorescent properties to a compound. In this respect, heterocycles synthesized using ketene dithioacetals have good substituents show fluorescence, due to ketene dithioacetals having strong electro-donating groups such as a methylsulfanyl group and strong electro-withdrawing groups such as cyano and ester groups. Therefore, many fluorescent materials are expected to be included under the category of heterocycles that we have previously synthesized. While in search of a solid fluorescent material, we found that 2H-pyrone derivatives exhibit strong fluorescence; this was first reported in 1977.27 A variety of pyrone derivatives were obtained via the reactions of various active methylene compounds with ketene dithioacetals; however, not all these derivatives were found to be fluorescent compounds. Our research suggests that the substitution of an aryl group without electro-withdrawing group such as a phenyl group at position 6 and not position 5 of the pyrone ring was important for fluorescence to occur. For example, 6-methyl- (9a), 6-(4-cyanophenyl)- (9o), 5-cyano- (16), and 6-pyrid-3-yl-2H-pyrone (9r) do not show fluorescence (Schemes 1 and 2).
In a similar manner to 9 and 14, methyl ester compounds (17a–m) were synthesized via the reactions between arylacetyl compounds and ketene dithioacetal (2b), which was prepared from methyl malonate (12) (Scheme 3). In addition, reactions of methyl ketones with sulfonyl ketene dithioacetal (2c, d) afforded 3-phenylsulfonyl derivatives (18a−d) (Scheme 4).28
The introduction of electro-withdrawing groups such as cyano, ester, and sulfonyl groups at position 3 of the pyrone ring was necessary for fluorescence to occur in 2-pyrone derivatives. The introduction of electron-rich aromatic compounds at position 6 of the pyrone ring increased the intensity of fluorescence. In the case of derivatives in which electron-poor compounds were introduced, such as pyridine or 4-cyanophenyl derivative (9o), no fluorescence emission was observed.29 However, 6-pyridyl-3-phenyl-4-sulfanyl-2-pyrone derivatives (21a–c), especially 3′,4′-dimethoxyphenyl derivative (21c), exhibit strong fluorescence (Scheme 5).30 These results suggest that derivatives with an electron-rich group and an electron-poor group at either position 3 or 6 of the pyrone ring, respectively, exhibit fluorescence. Compounds 21a–c were obtained via the reactions of phenylacetonitrile (19a–c) with the ketene dithioacetal (2e) in the presence of sodium hydroxide as a base in DMSO followed by neutralization with hydrochloric acid.
3. SYNTHESIS OF 2-PYRONE DERIVATIVES VIA THE NUCLEOPHILIC-SUBSTITUTION REACTION OF 4-METHYLSULFANYL-2-PYRONES
When 2-pyrone derivatives are synthesized using ketene dithioacetals, they have a methylsulfanyl group at position 4 of the pyrone ring, which can be easily substituted by other functional groups. The reactions of 9a–e with methyl alkoxide afford 4-methoxy-2-pyrone derivatives (22a–e) (Scheme 6), and the reactions of ester compounds (17a–d) with methyl alkoxide produce 2-pyrone derivatives such as methoxyphenylcoumarin (23a)31 and methoxyparakotoin (23d)32 that are found in natural products, albeit
very weak intensities (Scheme 7). In order to avoid altering the ester group, the above reaction was performed and was then followed by conversion of the methylsulfanyl group to a sulfonyl group; this reaction was found to be effective (Scheme 8).33
4-Amino-2-pyrone derivatives (26a–y, 27a–f) were synthesized by reacting methylsulfanyl and methylsulfenyl derivatives (9, 24) with amines (Schemes 9 and 10). Although only aliphatic amine derivatives are examined in this section, aromatic amine derivatives are of more interest from the viewpoint of fluorescence. These derivatives will be examined in the future. Amine derivatives are easily synthesized using an excess of amines in methanol; however, the reactions are slow. Without the presence of a solvent, 9 directly reacts with amines; however, this reaction is accompanied by the ring-opening reaction of the pyrone ring.34 The resulting amine derivatives, in which the sulfur atom at position 4 of the pyrone ring is replaced by an oxygen or nitrogen atom, show relatively strong fluorescence, and the emitting regions showed an approximately 30–50 nm blue shift.
Malonate derivatives (29a–h, 30a–e) are obtained in good yields via the reactions of malonates (28a, b) with 4-methylsulfany-2-pyrone derivatives (9, 14, 17) (Tables 1 and 2).35 Using various active methylene compounds (31), pyranopyridine derivatives (32a–h) (Table 3) and pyranopyrone derivative (33a–e) (Table 4) have also been synthesized.36,37 Among these compounds, 29h is found to emit more red light than DCM (630 nm), which is known as a red fluorescent compound. In addition, pyranopyrone derivatives (33a–e) are also obtained via the reaction between the active methylene compound and 31
with no emission of fluorescence. In contrast, 32a–h exhibit fluorescence due to an intramolecular
H-bonded structure resulting between the proton of the hydroxyl group and the carbonyl group, which leads to a molecular flatness arising from electron delocalization.35
Coumarin derivatives, which are fused pyrone derivatives, are essential intramolecular excimer-forming fluorescence derivatization reagents required for high-sensitivity analysis. The fused pyrone derivatives (33a–e) obtained from ketene dithioacetal show weak or no fluorescence; however, the pyrano[4,3-b]quinolizine derivatives (36a–d) shown in Scheme 11 exhibit strong fluorescence. These derivatives are easily synthesized via the reactions of pyridine-2-acetonitriles (35a, b) (an active methylene compound) with 2-pyrone derivatives (9b, c, j) in the presence of potassium carbonate as the base in DMSO. The reaction of these compounds results in the formation of an intramolecular hydrogen bond as shown in 32a–h. However, although the pyrano[4,3-b]quinolizine derivative (37) does not possess a hydrogen bond, it exhibits fluorescence when synthesized via the reaction of 35b with 17c. Moreover, its decarboxylated derivative (38) is easily obtained by treating 37 with polyphosphoric acid (PPA). This derivative shows fluorescence although it does not contain an electro-withdrawing group. These results suggest that fluorescence is determined by the presence of a quinolizine ring. For similar reasons, 40a and b synthesized via the reaction of 2-aminopyridine (39) with 17a and c exhibited yellow fluorescence. Thus, stretching the conjugated structures of 2-pyrone derivatives affords near-infrared fluorescent dyes.38
3. FLUORESCENCE PROPERTY
In 2-pyrone derivatives, the arrangement of the substitution groups in the pyrone ring is very important for fluorescence. In particular, aryl groups such as the phenyl group at position 6 have a significant effect on the fluorescent properties. Compounds possessing alkyl groups such as the methyl group at position 6 exhibit no fluorescence. Figure 2 shows representative fluorescent 2H-pyrone derivatives. An aryl group such as the phenyl group is absolutely necessary at position 6 in order for it to emit fluorescence. Further, electro-donating groups such as an amino or alkoxy group on the aryl group influence the fluorescence emission and bathochromic shifts. The dimethylamino derivative (9j) emits at 608 nm and the 6-(4-dimethylamino)styryl derivative (14d) emits at 610 nm in dichloromethane; both these are red fluorescent compounds (Figure 3). Electro-withdrawing groups such as the cyano group are necessary at position 3 of the pyrone ring. Moreover, ester derivatives (17a, 18d) exhibit stronger fluorescence than cyano derivatives. Detailed structural analysis using X-ray crystallography has revealed that the intramolecular nonbonded S…O (SMe…CO2Me) distance is 2.735 Å, which is shorter than the sum of
the van der Waals distance for O and S (3.25 Å). This result suggests the presence of strong molecular stacking because of the planar nature of molecules, and this strong molecular stacking strongly influences the fluorescence properties. The same phenomenon is observed when compounds 32 and 33 are compared. Although compound 33 does not exhibit fluorescence in the solid state, compound 32, which has an intramolecular H-bonded structure due to the C=O----H-O-C system, exhibits strong fluorescence. The reaction of 4-methylsulfanyl-2-pyrone derivatives with various nucleophilic reagents, as shown in Scheme 6–11 and Table 1–4, produces various derivatives by displacing the methylsulfanyl group at position 4 of the pyrone ring. Amino derivatives such as compounds 26a and 26l show stronger fluorescence; however, they also exhibit hypsochromic shifts. The malonate derivative (29e) exhibits a slight bathochromic shift and stronger fluorescence. In particular, the 6-(4-dimethylamino)styryl derivative (29h) emits at 620 nm, which is close to the ideal wavelength for red-fluorescent compounds used in EL devices. The derivatives not substituted at positions 5 have much greater fluorescence than compounds with an electro-withdrawing or electro-donating group at that position. This fact suggests that derivatives bridged at positions 5 and 6 exhibit no fluorescence. Our findings show that an electron-rich aryl group is necessary at position 6 of the pyrone ring for the emission of fluorescence, and derivatives containing electron-poor heterocycles such as the pyridyl group exhibit no fluorescence. However, the introduction of a phenyl group at position 3 of the 6-pyridyl-2-pyrone ring in 21a affects the fluorescence expression. Consequently, in 2-pyrone derivatives, the introduction of various substituents at each position enables the control of the light-emission region and intensity, and also allows us to design pigments with various functionalities.39
4. CONCLUSION
Display devices using organic EL materials are making rapid progress in terms of practical use. Studies on novel organic EL compounds are underway, with particular focus on aromatic compounds. Heterocycles, in which a carbon atom in the aromatic ring is replaced by an oxygen or nitrogen atom, have also received considered attention due to their diversity. In fact, many fluorescent heterocycles have been reported as diagnostic reagents or intramolecular excimer-forming fluorescence derivatization reagents for use in biological samples and pharmaceuticals. These findings will contribute to the development of organic EL materials. In addition, fluorescent heterocycles will be applicable not only to the synthesis of optimal organic EL materials but also to the synthesis of exotic materials that support the progress in technology, such as laser dyes and chemical luminous materials. We expect that ketene dithioacetals will be the best starting materials for the synthesis of various useful dyes. For almost 5 decades, there has been research on these reagents; however, their application to the synthesis of merocyanine dyes and chemical luminescence reagents is only a recent development. Therefore, these reagents will be applied in the development of useful fluorescent dyes.
References
1. O. Meth-Cohn and M. Smith, J. Chem. Soc., Perkin Trans. 1, 1994, 5. CrossRef
2. W. N. Watson and C. H. Penning, Ind. Eng. Chem., 1926, 18, 1309. CrossRef
3. A. T. Peters and H. S. Freeman, ‘Color Chemistry’, Elsevier Applied Science, London and New York, 1991.
4. D. R. Waring and G. Hallas, ‘The Chemistry and Application of Dyes’, Plenum Press, New York and London, 1990.
5. F. Jensen, ‘Ann. Rep. Comput. Chem.,’ Vol. 1, Elsevier Science Ltd, Amsterdam, 2005.
6. A. Dreuw and M. H. Gordon, Chem. Rev., 2005, 105, 4009. CrossRef
7. H. Hibst, J. Magn. and Magn. Mater., 1988, 74, 193. CrossRef
8. C. Lal, J. Power Sources, 2007, 164, 926. CrossRef
9. T. Hasobe, S. Hattori, P. V. Kamat, Y. Urano, N. Umezawa, T. Nagano and S. Fukuzumi, Chem. Phys., 2005, 319, 243. CrossRef
10. I. Tanaka, Y. Inoue, N. Ishii, K. Tanaka, Y. Izumi and S. Okamoto, Displays, 2002, 23, 249. CrossRef
11. K. Asai, Y. Amao, Y. Iijima, I. Okura and H. Nishide, J. Thermophys. Heat Transfer, 2002, 109.
12. C. W. Tang, S. A. Vanslyke and C. H. Chen, J. Appl. Phys., 1989, 65, 3610. CrossRef
13. R. Gompper and W. Topfl, Chem Ber., 1962, 95, 2861. CrossRef
14. Y. Tominaga and Y. Matsuda, J. Heterocycl. Chem., 1985, 37, 937. CrossRef
15. Y. Tominaga and Y. Matsuda, Yuki Gousei Kagaku Kyokai-shi (J. Synth. Org. Chem. Jpn), 1985, 43, 669. CrossRef
16. Y. Tominaga, S. Kohra, H. Honkawa and A. Hosomi, Heterocycles, 1989, 29, 1409. CrossRef
17. Y. Tominaga, ‘Trends in Heterocyclic Chemistry,’ Vol. 2, ed. by J. Menon, Research Trends, India, 1991, pp. 43-83.
18. R. K. Dieter, Tetrahedron, 1986, 42, 3029. CrossRef
19. H. Ila, H. Junjappa and P. K. Mohanta, Progress in Heterocyclic Chemistry, 2001, 13, 1. CrossRef
20. Y. Tominaga, S. Hidaki, Y. Matsuda, G. Kobayashi and K. Sakemi, Yakugaku Zasshi, 1984, 104, 134.
21. Y. Matsuda, H. Gotou, K. Katou, H. Matsumoto, M. Yamashita and S. Maeda, Dyes and Pigments, 1990, 14, 225. CrossRef
22. Y. Tominaga, J. K. Luo, L. W. Castle and R. N. Castle, J. Heterocycl. Chem., 1993, 30, 267. CrossRef
23. Y. Tominaga, N. Yoshioka, S. Kataoka, N. Aoyama, T. Masunami and A. Miike, Tetrahedron Lett., 1995, 36, 8641. CrossRef
24. Y. Tominaga, N. Yoshioka, S. Kataoka, Y. Shigemitsu, T. Hirota and K. Sasaki, Heterocycles, 1999, 50, 43. CrossRef
25. Y. Tominaga, K. Komiya, S. Kataoka, Y. Shigemitsu, T. Hirota and K. Sasaki, Heterocycles, 1998, 48, 1985. CrossRef
26. Unpublished.
27. Y. Tominaga, A. Ushirogouchi, Y. Matsuda and G. Kobayashi, Heterocycles, 1977, 8, 193. CrossRef
28. N. Mizuyama, Y. Murakami, S. Kohra, K. Ueda, K. Hiraoka, J. Nagaoka, K. Takahashi, Y. Shigemitsu and Y. Tominaga, J. Hetercycl. Chem., 2007, 44, 115. CrossRef
29. Y. Tominaga, N. Mizuyama and Y. Murakami, Jpn. Kokai Tokkyo Koho, JP2006206523, .
30. N. Mizuyama, S. Kohra, K. Ueda, K. Hiraoka, K. Takahashi and Y. Tominaga, Heterocycles, 2007, 71, 399. CrossRef
31. O. R. Gottlieb and W. B. Mors, J. Am. Chem. Soc., 1958, 80, 2263. CrossRef
32. J. Kalff, Rec. Trav. Chim., 1927, 46, 596.
33. Y. Tominaga, A. Ushirogouchi and Y. Matsuda, J. Heterocycl. Chem., 1987, 24, 1557. CrossRef
34. N. Mizuyama, Y. Murakami, J. Nagaoka, S. Kohra, K. Ueda, K. Hiraoka, Y. Shigemitsu and Y. Tominaga, Heterocycles, 2006, 68, 1105. CrossRef
35. N. Mizuyama, Y. Murakami, S. Kuronita, S. Kohra, K. Ueda, R. Hiraoka and Y. Tominaga, J. Heterocycl. Chem., 2008, 45, 1. CrossRef
36. Y. Tominaga, Y. Matsuda and G. Kobayashi, Chem. Pharm. Bull., 1984, 32, 1665. CrossRef
37. Y. Tominaga, A. Ushirogouchi, Y. Matsuda and G. Kobayashi, Chem. Pharm. Bull., 1984, 32, 3384. CrossRef
38. M. Hagimori, N. Mizuyama and Y. Tominaga, ARKIVOC, 2008, xiii, 16.
39. Y. Tominaga, N. Mizuyama, Y. Murakami, S. Kohra, K. Hiraoka and K. Ueda, Journal of Environmental Studies Special Issue Celebrating Ten Years of Establishment, 2007, 7.