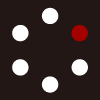
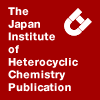
HETEROCYCLES
An International Journal for Reviews and Communications in Heterocyclic ChemistryWeb Edition ISSN: 1881-0942
Published online by The Japan Institute of Heterocyclic Chemistry
e-Journal
Full Text HTML
Received, 9th September, 2008, Accepted, 30th October, 2008, Published online, 31st October, 2008.
DOI: 10.3987/COM-08-11544
■ Efficient Syntheses of 1-Azatricyclic Ring Systems from Anthranylamide
Daniele Muroni, Mauro Mucedda, and Antonio Saba*
Department of Chemistry, University of Sassari, Via Vienna 2, I-07100 Sassari, Italy
Abstract
Concise syntheses of novel tricyclic quinazolinone and pyrrolo benzoazacyclononenone alkaloids in two and three steps (two pots), starting from 3-styryl quinazolinone, by utilizing RCM and cascade spiro-to fused protocols, respectively, were reported.INTRODUCTION
As apart of the ongoing efforts devoted to optimize synthetic protocols to prepare 1-azacyclic alkaloids by using simple reagents and concise procedures, as cascade cyclization processes,1 or ring closing metathesis (RCM),2 herein we describe a synthetic approach to the family of tricyclic quinazolinone and medium sized azacyclic systems by utilizing both these procedures, even starting from N-benzyl- antranylamide, easily available and convenient material.
Tricyclic systems which possesses the 4(3H)quinazoline moiety3 are encountered in medicinal chemistry for their interesting pharmacological activities as respiratory stimulant, bronchodilator4 or antihistaminic and sedative-hypnotic activity.5
For such purpose, construction of five- six- and seven-membered rings by RCM of quinazolinone derivatives, bearing two unsaturated pendants properly situated, has been planned (Scheme 1).
RESULT AND DISCUSSION
Acylation of racemic 3-styryl-2-benzyl-quinazolinone 1, prepared from N-benzyl antranylamide,6 with acryloyl, ω-butenoyl and ω-pentenoyl chlorides, in the presence of pyridine or triethylamine, afforded the quinazolinone dienes 2a, 2b and 2c.
The N-acyl in the place of N-alkyl derivatives choice was due to knowledge that the Grubb’s RCM catalytic protocol may present difficulties in metathesizing compounds containing amino groups, whose nitrogen lone pair generally tends to coordinate the metal centre, poisoning and deactivating of ruthenium catalyst.
In order to prevent this inconvenience, conversion of the amine into carbamate, sulfonamide or amide functions have been carried out.7 However, increasing examples of successful metathesized amine compounds are reported.8
With the desired diene compounds in hand, we studied with accuracy the RCM reaction in order to field optimum conditions. After surveying several catalysts, the Grubb’s second generation one (Grubb’s II), was chosen, being better its efficiency. Then the catalytic intramolecular cyclizations were performed in different reaction conditions and the results are summarized in Table 1.
By refluxing of compound 2a in CH2Cl2, CH2ClCH2Cl and PhMe solutions for 2 days in the presence of 5 mol% of Grubb’s II catalyst, no traces of the desired tricyclic alkaloid were observed. This failure could be ascribed to a strain opposed to the construction of a five membered-ring on the pre-existing six-membered one. This is probably due to the particular rigidity of the latter, concomitant with that of the amidic alkene pendant. By refluxing a CH2ClCH2Cl solution of 2b for 1.5 h, in the presence of Grubb’s II catalyst (5 mol%), the conversion was complete and the target alkaloid 3b was obtained as the sole product in excellent yield. Changing the reaction conditions, both conversion and yield decrease (Entries 4 and 6).
In the case of diene compound 2c (Entry 8) a 71/29 mixture of the target azepino quinazolinone 3c and the undesired 3b was obtained. The formation of the latter compound is probably due to the terminal double bond isomerization of substrate 2c preceding the intramolecular cyclization step. Anyhow, the homologous compounds 3c and 3b were easily separated by flash chromatography (light petroleum ether/ethyl acetate 7:3). Aiming to minimize this isomerization, the reaction of 2c has been performed in the same solvent at milder conditions (40 °C, Entry 9). In this case, as in boiling CH2Cl2 solution (Entry 7), the exclusive formation of 3c is compromised by a very low conversion.
It is noteworthy that better results were obtained in CH2ClCH2Cl than in CH2Cl2 solution, generally employed in the RCM reactions; this suggests that suitable temperatures are required in the process. Moreover, 1,2-dichloroethane has been shown to be more effective than toluene, at a given reaction temperature.
Due to combination of two or more distinct reactions into a single transformation, cascade or tandem processes are powerful methods for constructing complex structures starting from relatively simple materials. In this field, intramolecular cyclizations based on metallo carbenoids generated by transition metal catalyzed decomposition of diazo compounds9 and leading to ammonium ylides, which spontaneously undergo sigmatropic [1,2]- or [2,3]-rearrangements, has gained significant importance in organic synthesis and has become a versatile strategy for the synthesis of nitrogen heterocycles.10
For this purpose, utilization of the same styryl quinazolinone 1 as the starting material, was planned (Scheme 2). In this case, the initiation of cascade process is based upon catalytic decomposition of 4. Generation of metallo carbenoid species with intramolecular attack to the amine nitrogen is expected to produce the spirocyclic ylide 5. This intermediate could rearrange with [2,3]-shift,11 across the pendant unsaturated group, leading to the nine-membered pyrrolo azacycle 6 with three carbons expansion of the heterocycle ring moiety. Nevertheless, the presence of a conjugative styryl function, situated on the putative migrating carbon, could activate the ylide Stevens [1,2]-rearrangement,12 consequently, formation of the pyrrolo benzodiazepine 7 would not be avoided, in principle (Figure 1).
Effectively, decompositions of the corresponding diazo quinazolinones, bearing the carbon migrating atom activated by an ester group, afforded the Stevens [1,2]-shift products in acceptable total yields and complete stereoselectivity, as recently reported by our group.1e
The cyclization precursor 4 was conveniently prepared by two steps one pot conjugate addition of 1 to ethyl 3-keto-pent-4-enoate,13 followed by diazo-transfer reaction with tosyl azide.
Two transition metal catalysts were studied. The Cu(acac)2 catalyzed diazo-decomposition of 4, carried out in refluxing toluene, gave mixtures of the expected pyrrolo benzoazacyclononenone 6 and the pyrrolobenzodiazepinone 7, in 81/19 ratio (NMR spectrometry). The same reaction, performed by using Rh2(OAc)4 in refluxing toluene, afforded mixtures of 6 and 7 in 54/46 ratio, respectively. In both cases the decompositions gave quantitative overall yields, in spite of preceding data demonstrating the copper-based catalysis to be more effective for generation of ammonium ylides.14
Olefin geometry of 6 has been assigned on the base of spectroscopic data.15 No traces of the intermediate ylide 5 was observed: at the employed reaction conditions it was impossible its isolation or spectroscopic detection.
CONCLUSIONS
This work reports very concise and convenient syntheses of novel tricyclic quinazolinone in two steps and pyrrolo benzoazacyclononenone alkaloids in three steps two pots, starting from a simple material as 3-styryl quinazolinone, by utilizing the RCM and cascade spiro-to fused protocols, respectively.
Of particular significance, both these procedures utilizes no additional reagents beyond a catalyst and the other product from the reaction is a volatile olefin (RCM) or nitrogen (cascade).
The efficacy of the tandem ylide formation/rearrangement reaction suggests a valid approach to the medium sized azacycloalkene system containing natural products.16
It is noteworthy that amino esters, conformationally constrained analogues to natural amino acids, can be recognized into both the diazepinone and azacyclononenone frames, consequently it could be of interesting synthetic tool.17
Moreover, larger cycle amino ester as 6 could prove useful in peptide mimic design.18
Finally, the presence of a carbon-carbon double bond make the alkaloids 3 and 6 further functionalizable.
Biological evaluations and structure-activity relationship (SAR) will be reported in due course.
EXPERIMENTAL
General
1H NMR (300 MHz) and 13C NMR (75 MHz) spectra were recorded on a Varian VXR-300 spectrometer with TMS as internal standard. Infrared (IR) spectra were performed on a FT/IR-480plus JASCO spectrophotometer. All reagents and solvents employed were reagent grade materials purified by standard methods and redistilled before use.
(E)-1-Acryloyl-3-benzyl-2-styryl-2,3-dihydroquinazolin-4(1H)-one 2a.
A CH2Cl2 (0.5 mL) solution of acryloyl chloride (0.68 mL, 8 mmol) was added dropwise to a solution of (E)-3-benzyl-2-styryl-2,3-dihydroquinazolin-4(1H)-one 1 (1.3 g, 3.8 mmol), and TEA (1.6 mL, 11.4 mmol) in CH2Cl2 (25 mL) and stirred at 0 °C under nitrogen atmosphere. After the addition was complete, the solution was stirred at rt for 21 h. The solvent was evaporated and the residue gave, after flash chromatography (light petroleum ether/EtOAc 8:2), the quinazolinone diene 2a (0.8 g, 53%), yellow crystals, mp 56-58 °C. 1H NMR (CDCl3): δ 4.24 (AB system, 1H), 5.38 (AB system, 1H), 5.77 (t, 1H, J=5.7 Hz), 5.98 (dd, 1H, J=15.9, 6.0 Hz), 6.47-6.62 (m, 3H), 7.10-7.47 (m, 13H), 7.44 (t, 1H, J=7.8, Hz ), 8.14 (d, 1H, J=6.6 Hz). 13C NMR (CDCl3): δ 48.2, 67.2, 122.0, 122.7, 123.3, 125.8, 126.5, 127.6, 127.7, 127.9, 128.2, 128.3, 128.6, 130.3, 132.2, 134.0, 134.9, 136.0, 136.6, 161.5, 163.6. IR (nujol): 3028, 2924, 1668, 1649, 1467, 1376, 1318, 1222, 1159, 1105, 1077, 1028, 965, 924 cm-1. Anal. Calcd for C26H22N2O2: C, 79.16; H, 5.62; N, 7.10%. Found: C, 78.93; H, 5.65; N, 7.07%.
(E)-3-Benzyl-1-but-3-enoyl-2-styryl-2,3-dihydroquinazolin-4(1H)-one 2b.
A CH2Cl2 (1 mL) solution of 3-butenoyl chloride (0.35 g, 3.4 mmol) was added dropwise to a solution of (E)-3-benzyl-2-styryl-2,3-dihydroquinazolin-4(1H)-one 1 (1.06 g, 3.1 mmol), DMAP (0.04 g, 0.31 mmol) and pyridine (0.53 mL, 6.6 mmol) in CH2Cl2 (15 mL) and stirred at 0 °C under nitrogen atmosphere. After the addition was complete, the solution was stirred at rt for 6 h. The solvent was evaporated and the residue gave, after flash chromatography (light petroleum ether/EtOAc 7:3), the quinazolinone diene 2b (0.83 g, 65%), amorphous yellow solid. 1H NMR (CDCl3): δ 2.96-3.41 (m, 2H), 4.20 (AB system, 1H), 4.99-5.0 (m, 1H), 5.13 (d, 1H, J=7.2 Hz), 5.39 (AB system, 1H), 5.78-5.96 (m, 2H), 6.45 (d, 1H, J=15.6 Hz), 7.19-7.35 (m, 13H), 7.49 (dt, 1H, J=7.8, 2.1 Hz ), 8.14 (d, 1H, J=6.9 Hz). 13C NMR (CDCl3): δ 38.9, 48.2, 67.2, 118.6, 122.1, 123.4, 123.7, 126.4, 126.6, 126.8, 128.2, 128.3, 128.4, 128.5, 128.7, 130.4, 132.1, 132.3, 133.9, 134.9, 136.2, 137.2, 161.4, 169.2. IR (Nujol): 3028, 2923, 1679, 1651, 1604, 1470, 1448, 1375, 1256, 1202, 1160, 1077, 1028, 966, 922, 757 cm-1. Anal. Calcd for C27H24N2O2: C, 79.39; H, 5.92; N, 6.86%. Found: C, 79.28; H, 5.94; N, 6.90%.
(E)-3-Benzyl-1-pent-4-enoyl-2-styryl-2,3-dihydroquinazolin-4(1H)-one 2c.
To a solution of (E)-3-benzyl-2-styryl-2,3-dihydroquinazolin-4(1H)-one 1 (1.43 g, 4.2 mmol), DMAP (0.05 g, 0.42 mmol) and pyridine (0.72 mL, 9.0 mmol) in CH2Cl2 (30 mL) a CH2Cl2 (1 mL) solution of 4-pentenoyl chloride (0.51 g, 4.6 mmol) was added dropwise and stirred at 0 °C under nitrogen atmosphere. After the addition was complete, the solution was stirred at rt for 21 h. The solvent was evaporated and the residue gave, after flash chromatography (light petroleum ether/EtOAc 7:3), the quinazolinone diene 2c (1.05 g, 60%), amorphous yellow solid. 1H NMR (CDCl3): δ 2.25-2.54 (m, 4H), 4.14 (AB system, 1H), 4.89-4.96 (m, 2H), 5.43 (AB system, 1H), 5.60-5.72 (m, 1H), 5.93 (dd, 1H, J=15.9, 2.1 Hz), 6.47 (d, 1H J=15.9 Hz), 7.09-7.34 (m, 13H), 7.49 (t, 1H, J=7.5 Hz). 8.14 (d, 1H, J=7.5 Hz). 13C NMR (CDCl3): δ 28.9, 32.9, 66.8, 115.5, 122.1, 123.3, 123.7, 125.8, 126.0, 126.5, 127.7, 128.1, 128.2, 128.3, 128.4, 128.6, 132.2, 133.7, 134.9, 136.1, 136.2, 137.1, 161.6, 170.5. IR (CHBr3): 3019, 2923, 1668, 1648, 1604, 1471, 1447, 1378, 1312, 1206, 1143, 1077, 1028, 965, 918, 756, 693 cm-1. Anal. Calcd for C28H26N2O2: C, 79.59; H, 6.20; N, 6.82%. Found: C, 79.78; H, 6.17; N, 6.85%.
General procedure for the RCM reaction
A solution of substrate (2.5 mmol) in 5 mL of dry degassed toluene was added dropwise to a solution of 2.2 mol% of catalyst in 5 mL of the same solvent; afterward the mixture was refluxed under argon. The solvent was evaporated and the residue was submitted to flash chromatography (light petroleum ether/EtOAc 7:3).
5-Benzyl-4a,5-dihydro-2H-pyrido[1,2a]quinazoline-1,6-dione 3b: white crystals, mp 122-124 °C. Yield 96%. 1H NMR (CDCl3): δ 2.87 (AB system, 1H), 3.20 (AB system, 1H), 4.74 (AB system, 1H), 5.09 (AB system, 1H), 5.72-5.84 (m, 1 H), 5.82 (AB system, 1H), 6.07 (AB system, 1H), 7.13-7.34 (m, 5H), 7.38 (dt, 1H, J=7.5, 1.5 Hz), 7.57 (dt, 1H, J=7.5, 0.9 Hz), 7.83 (d, 1H, J=8.1 Hz), 8.18 (dd, 1H, J=6.3, 1.5 Hz). 13C NMR (CDCl3): 32.4, 45.8, 69.2, 117.9, 123.7, 123.8, 126.1, 126.5, 127.2, 128.5, 128.7, 128.8, 132.2, 137.9, 139.7, 164.0, 165.5. IR (CHBr3): 2924, 1674, 1652, 1603, 1479, 1465, 1446, 1374, 1331, 1296, 1027, 975, 921, 888, 850, 795, 729, 693, 654. Anal. Calcd for C19H16N2O2: C, 74.98; H, 5.30; N, 9.20%. Found: C, 74.79; H, 5.27; N, 9.24%.
(Z)-6-Benzyl-6,6a,9,10-tetrahydroazepino[1,2a]quinazoline-5,11-dione 3c: white crystals, 48-50 °C. Yield 71%. 1H NMR (CDCl3): δ 2.50-2.62 (m, 3H), 3.05-3.18 (m, 1H), 4.05 (AB system, 1H), 5.61 (AB system, 1H), 5.70-5.79 (m, 1H), 5.88 (ddd, 1H, J=11.1, 4.5, 2.1 Hz), 6.24 (dd, 1H, J=4.2, 2.1 Hz), 7.19 (dt, 1H, J=7.2, 1.2 Hz), 7.24-7.42 (m, 5H), 7.48 (dt, 1H, J=7.5, 1.5 Hz), 8.13 (dd, 1H, J=7.8, 1.5 Hz), 8.31 (d, 1H, J=8.4 Hz). 13C NMR (CDCl3): 25.7, 36.2, 47.6, 66.0, 118.3, 119.2, 123.9, 127.9, 128.1, 128.6, 129.0, 129.5, 133.1, 133.5, 135.6, 138.1, 161.0, 172.8. IR (CHBr3): 1679, 1655, 1601, 1479, 1460, 1428, 1391, 1376, 1296, 1264, 1197, 873, 754, 654. Anal. Calcd for C20H18N2O2: C, 81.15; H, 5.92; N, 8.23%. Found: C, 79.88; H, 5,89; N, 8.19%.
(E)-Ethyl 5-(3-benzyl-4-oxo-2-styryl-3,4-dihydroquinazolin-1(2H)-yl)-2-diazo-3-oxopentanoate 4.
A solution of (E)-3-benzyl-2-styryl-2,3-dihydroquinazolin-4(1H)-one 1 (0.60 g, 1.76 mmol), and 3-oxo-pent-4-enoic acid ethyl ester (0.50 g, 3.52 mmol), in MeCN (15 mL) was stirred at rt for 7 days. The solvent was evaporated and a solution of tosyl azide (0.52 g, 2.6 mmol) and TEA (0.37. mL, 2.6 mmol) in CH2Cl2 (15 mL) were added dropwise at 0 °C. After the addition was complete, the solution was stirred at rt overnight. The solvent was evaporated and the residue gave, after flash chromatography (light petroleum ether/EtOAc 7:3), the diazo compound 4a (0.53 g, 61%) as a yellow oil. 1H NMR: δ 1.27 (t, 3H, J=8.0 Hz), 3.02 (AB system, 1H,), 3.20 (AB system, 1H), 3.46-3.56 (m, 2H), 3.90 (AB system, 1H), 4.21 (q, 2H, J=8.0 Hz), 5.05 (d, 1H, J=8.4 Hz), 5.55 (AB system, 1H), 6.21 (dd, 1H, J=15.6, 8.4 Hz), 6.51 (d, 1H, J=15.6 Hz),), 6.70 (d, 1H, J=8.4 Hz), 6.87 (t, 1H, J=7.5 Hz), 7.22-7.35 (m, 11H), 8.04 (dd, 1H, J=7.8, 1.5 Hz). 13C NMR (CDCl3): δ 14.2, 38.5, 42.6, 46.7, 61.5, 75.6, 111.9, 116.6, 118.3, 122.7, 126.8, 127.4, 128.4, 128.6, 129.4, 133.8, 135.4, 136.8, 145.4, 160.9, 162.5, 190.3. IR (neat): 3028, 2980, 2136, 1714, 1650, 1604, 1492, 1454, 1372, 1306, 1251, 1218, 1163, 1132, 1098, 1048, 1028, 992, 912, 746, 695 cm-1. Anal. Calcd for C30H28N4O4: C, 70.85; H, 5.55; N, 11.02%. Found: C,71.01; H, 5.51; N, 10.99%.
General procedure for the diazo-decomposition
To a refluxing solution of catalyst (Rh2OAc4, 2.6 mg, 3 mol% or Cu (acac)2, 2.6 mg, 5 mol%) in 6 mL of dry toluene, a solution of diazo compound (0.2 mmol) in 5 mL of dry toluene was added dropwise over 30 min. After stirring for 30 min at reflux, the mixture was cooled, and after evaporation of the solvent, purified by flash chromatography (light petroleum ether/ Et OAc 7:3).
(E)-Ethyl 4-benzyl-5,15-dioxo-1-phenyl-4,5,13,14,15,15a-hexahydro-1H-benzo[b]-1H-pyrrolo [1,2i] [1,5]diazonine-15a carboxylate 6: (yield 74.9%), white crystals, mp 45-47 °C. 1H NMR (CDCl3): δ 1.15 (t, 3H, J=7.2 Hz ), 2.50-2.62 (m, 1H), 2.72-2.82 (m, 1H), 3.81 (q, 1H, J=7.2 Hz), 3.94-4.17 (m, 3H), 4.28 (AB system, 1H), 4.97 (d, 1H, J=7.4 Hz), 5.51 (dd, 1H, J=15.9, 7.4), 5.60 (AB system, 1H), 5.92 (d, 1H, J=15.9 Hz), 6.94-6.99 (m, 3H), 7.15-7.39 (m, 9H), 7.47 (dt, 1H, J=7.4, 1.8 Hz). 8.47 (dd, 1H, J=7.4, 1.8 Hz). 13C NMR (CDCl3): δ 14.1, 34.1, 43.6, 55.5, 64.4, 78.9, 113.3, 118.6, 119.3, 122.7, 127.1,126.6, 127.4, 128.0, 128.3, 128.4, 129.2, 132.8, 134.7, 135.8, 137.2, 142.9, 165.7, 167.1, 204.0. IR (CHBr3): 3413, 2256, 1769, 1733, 1614, 1492, 1478, 1451, 1372, 1291, 1227, 749, 692 cm-1. Anal. Calcd for C30H28N2O4: C, 74.98; H, 5.87; N, 5.83%. Found: C, 74.73; H, 5.83; N, 5.86%.
(Z)-Ethyl 5-benzyl-3,6-dioxo-4-styryl-2,3,5,6-tetrahydro-1H-4H-benzo[f]pyrrolo [1,2-a][1,4] diazepine-3a-carboxylate 7: (yield 21.1%), white crystals, mp 108-110 °C. 1H NMR (CDCl3): δ 0.98 (t, 3H, J=7.2 Hz), 2.61-2.72 (m, 2H), 3.55 (dt, 1H, J=8.4, 2.4 Hz), 3.80-3.98 (m, 3H), 4.25 (AB system, 1H), 4.85 (d, 1H, J=9.3 Hz), 5.35 (AB system, 1H), 5.58 (dd, 1H, J=15.9, 9.3 Hz), 5.69 (d, 1H, J=15.9 Hz), 6.78-6.81 (m, 2H), 7.13-7.36 (m, 9H), 7.44 (dd, 1H, J=7.8, 1.5 Hz), 7.53 (dt, 1H, J=7.8, 1.5 Hz), 7.76 (dd, 1H, J=7.8, 1.5 Hz). 13C NMR (CDCl3): δ 14.01, 14.04, 35.51, 43.27, 52.61, 61.16, 61.96, 77.20, 83.66, 121.47, 123.15, 124.27, 126.39, 127.63, 128.05, 128.39, 128.41, 129.41, 130.64, 131.50, 131.96, 135.70, 135.83, 137.25, 143.11, 166.53, 169.92, 203.28. IR (CHBr3): 2969, 1764, 1733, 1624, 1466, 1364, 1298, 1227, 1201, 1143, 1052, 965, 763, 693, 654 cm-1. Anal. Calcd for C30H28N2O4: C, 74.98; H, 5.87; N, 5.83. Found: C, 74.82; H, 5.84; N, 5.87.
ACKNOWLEDGEMENTS
The Authors are thankful to Fondazione Banco di Sardegna for financial support.
References
1. (a) A. Saba, Tetrahedron Lett., 2003, 44, 2895; CrossRef (b) D. Muroni, A. Saba, and N. Culeddu, Tetrahedron: Asymmetry, 2004, 15, 2609; CrossRef (c) D. Muroni, A. Saba, and N. Culeddu, Tetrahedron, 2006, 62, 1459; CrossRef (d) D. Muroni, A. Saba, and N. Culeddu, Heterocycles, 2006, 68, 47; CrossRef (e) M. Mucedda, D. Muroni, A. Saba, and C. Manassero, Tetrahedron, 2007, 63, 12232. CrossRef
2. D. Muroni, M. Mucedda, and A. Saba, Tetrahedron Lett., 2008, 49, 2373. CrossRef
3. For a review on quinazoline alkaloids, see: (a) J. P. Michael, Nat. Prod. Rep., 2000, 17, 603; CrossRef (b) S. Johne, ‘Supplements to the Second Edition of Rodd’s Chemistry of Carbon Compounds’, Vol. IV I/J, ed. by M. F. Ansell, Elsevier Inc., Amsterdam, 1995, pp. 223-240.
4. S. Johne, ‘The Alkaloids, Chemistry and Pharmacology’, Vol. 29, ed. by A. Brossi, Academic Press, Inc., New York, 1986, pp. 99-140.
5. (a) V. Alagarsamy, V. R. Solomon, and M. Murugan, Bioorg. Med. Chem., 2007, 15, 4009; CrossRef (b) A. S. Shawali, H. M. Hassaneen, and N. K. Shurrab, Heterocycles, 2008, 75, 1479; CrossRef (c) J. J. Wade, U. S. Patent , No. 4,528,288 (Chem. Abstr., 1986, 104, 5889); (d) W. R. West July, Eur. Patent , No. 34,529 (Chem. Abstr., 1982, 96, 20114).
6. T. A. Kilroe Smith and H. Stephen, Tetrahedron, 1957, 1, 38. CrossRef
7. (a) R. H. Grubbs, S. J. Miller, and G. C. Fu, Acc. Chem. Res., 1995, 28, 446; CrossRef (b) R. H. Grubbs, Tetrahedron, 2004, 60, 7117. CrossRef
8. P. Compain, Adv. Synth. Catal., 2007, 349, 1829. In this review examples are reported in which successful RCM reactions are performed utilizing substrates containing secondary and tertiary amines. CrossRef
9. A. Padwa and M. D. Weingarten, Chem. Rev., 1996, 96, 223. CrossRef
10. For general reviews of the Stevens rearrangement, see: (a) I. E. Markò, ‘The Stevens and related rearrangements’, ed. by B. M. Trost and I. Fleming, Wiley, Inc., Oxford, 1991, p. 913; (b) F. G. West and G. S. Clark, ‘Nitrogen, Oxygen and Sulfur Ylide Chemistry’, ed. by J. S. Clark, Oxford University Press, Inc., Oxford, 2002; (c) For a recent review of the Stevens rearrangement of ammonium ylides, see: J. A. Vanecko, H. Wan, and F. G. West, Tetrahedron, 2006, 62, 1043; CrossRef (d) For a review of ammonium ylides generated by diazo compounds, see: M. P. Doyle, M. A. McKervey, and T. Ye, ‘Modern Catalytic Methods for Organic Sinthesis with Diazo Compounds’, John Wiley and Sons, Inc., New York, NY, 1997.
11. For some examples of [2,3]-rearrangement of allylic ammonium ylides, see: (a) J. S. Clark and M. D. Middleton, Org. Lett., 2002, 4, 765; CrossRef (b) E. Roberts, J. P. Sancon, J. B. Sweeney, and J. A. Workman, Org. Lett., 2003, 5, 4775; CrossRef (c) C.-Y. Zhou, W.-Y. Yu, P. W. Chan, and C.-M. Che, J. Org. Chem., 2004, 69, 7072. CrossRef
12. (a) J. A. Vanecko and F. G. West, Org. Lett., 2002, 4, 2813; CrossRef (b) A. Padwa, L. S. Beall, C. K. Eidell, and K. J. Worsencroft, J. Org. Chem., 2001, 66, 2414; CrossRef (c) F. G. West, K. W. Glaeske, and B. N. Naidu, Synthesis, 1993, 977. CrossRef
13. R. Zibuck and J. M. Streiber, J. Org. Chem., 1989, 54, 4717. CrossRef
14. The copper-based catalysis has been demonstrated to be more effective: a) F. G. West, B. N. Naidu, and R. W. Tester, J. Org. Chem., 1994, 59, 6892; CrossRef (b) see ref. 1 (e).
15. Coupling constants were found by 1H 300 MHz NMR to be 15.9 Hz.
16. For the use of spirocyclic ammonium ylide rearrangements to prepare medium-sized azacycles, see: (a) D. L. Wright, R. M. Weekly, R. Groff, and M. McMills, Tetrahedron Lett., 1996, 37, 2165; CrossRef (b) J. S. Clark, P. B. Hodgson, M. D. Goldsmith, A. J. Blake, P. A. Cooke, and L. J. Street, J. Chem. Soc., Perkin Trans. 1, 2001, 3325. CrossRef
17. (a) F. G. West and B. N. Naidu, J. Org. Chem., 1994, 59, 6051; CrossRef (b) C. Cativiela and M. D. Diaz-de-Villegas, Tetrahedron: Asymmetry, 1998, 9, 3517; CrossRef (c) S. Hanessian, G. McNaughton-Smith, H.-G. Lombart, and W. D. Lubell, Tetrahedron, 1997, 53, 12789; CrossRef (d) see ref. 11 (a).
18. A. Giannis and T. Kolter, Angew. Chem., Int. Ed. Engl., 1993, 32, 1244. CrossRef