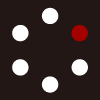
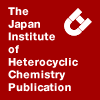
HETEROCYCLES
An International Journal for Reviews and Communications in Heterocyclic ChemistryWeb Edition ISSN: 1881-0942
Published online by The Japan Institute of Heterocyclic Chemistry
e-Journal
Full Text HTML
Received, 6th September, 2008, Accepted, 22nd October, 2008, Published online, 30th October, 2008.
DOI: 10.3987/COM-08-S(D)18
■ Asymmetric Synthesis of 4-Substituted 2,6-Dioxopiperidine-3-carbonitrile by Using Thiourea-Catalyzed Asymmetric Michael Addition
Tsubasa Inokuma, Yuuki Nagamoto, Shota Sakamoto, Hideto Miyabe, Kiyosei Takasu, and Yoshiji Takemoto*
Graduate School of Pharmaceutical Sciences, Kyoto University, 46-29 Yoshida-shimoadachi-machi, Sakyo-ku, Kyoto 606-8501, Japan
Abstract
An enantioselective Michael addition of several α,β-unsaturated carbonyl compounds with malononitrile catalyzed by a bifunctional thiourea is described. We also demonstrate the transformation of Michael adduct into an enantiomerically enriched functionalized piperidine.INTRODUCTION
Enantioselective formation of carbon-carbon bonds in a catalytic manner has been the subject of significant interest in the field of synthetic chemistry. Among a lot of excellent efforts on the catalytic enantioselective reactions, an asymmetric Michael reaction of α,β-unsaturated carbonyl compounds with activated methylene compounds, such as nitroalkane, 1,3-diketones and malononitrile, has been extensively studied.1 We have reported that bifunctinal thiourea catalyst (1)2 promoted the enantioselective Michael reaction of α,β-unsaturated imides with several activated methylene compounds (Scheme 1).3
The use of 2-methoxybenzimide 2, in which an intramolecular hydrogen bond between the methoxy group and the imide proton would be formed, accelerated the reaction rate and achieved excellent
asymmetric induction in the thiourea-catalyzed reaction.3b We envisioned that the product 3 might be a precursor of chiral piperidine derivatives such as 4 by the intramolecular cyclization (Scheme 2).4 A piperidine ring is a ubiquitous molecular skeleton, which often appears in naturally occurring substances such as antimalarial nakadomarin A 55 as well as synthetic pharmaceuticals such as anti-depressive paroxetine 6.6 With an aim of the synthesis of 5, we further explored several substrates 7 bearing a 3-furyl group as the β-substituent to reveal the effect of the substituent (X) of Michael acceptors 7 on the reactivity with malononitrile. In addition, synthetic application of the Michael adducts 8 for the preparation of chiral piperidine-2,6-dione derivative 10 was examined.
RESULTS AND DISCUSSION
In an initial study, we selected 2-methoxybenzimide 7a as a substrate for the asymmetric Michael reaction with malononitrile. In contrast to 2 (R = Ph),3b the reaction of 7a proceeded very slowly due to the electron-rich furyl group (Scheme 3). In addition, simple benzimide 7b was much poorer substrate for this reaction as expected. Then, in order to improve the reactivity of this reaction, we screened several α,β-unsaturated carbonyl compounds 7c and 7d together with the imides 7e and 7f. The reaction of 7c-f with malononitrile (2.0 equiv.) was carried out in toluene (0.1 M) at ambient temperature in the presence of 10 mol% of 1 until the substrate was completely consumed or, otherwise, for 1 week (168 h). The results are summarized in Scheme 3. The reaction of phenylketone 7c took place to give the desired Michael adduct 8c in 63% yield.7 However, the reaction did not complete within 168 h and the ee was modelate (70% ee). In a case of 7d bearing N-methyl imidazole as the acyl moiety, the reaction was complete within 64 h to give 8d in 91% yield with 64% ee.8 As a result, all these substrates were inferior to 7a in terms of both the reactivity and the enantioselectivity. Therefore, we reexamined other imides 7e and 7f. We speculated that if the methoxy group of 7a was replaced by the fluoro group, its electron-withdrawing property as well as its potential ability as a proton acceptor would make the substrates 7e and 7f more reactive than 7a. When o-fluorobenzimide 7e was utilized as a substrate for this reaction, the reaction was complete within 24 h and desired product 8e was obtained in good yield with 95% ee. On the other hand, the reaction of 2,6-difluorobenzimide 7f led to significant decrease in both reactivity and enantioselectivity.
We next elucidated the intramolecular hydrogen bond of the imide substrates by 1H NMR analysis (Figure 1). As reported previously, the chemical shifts (CDCl3 at 25 oC) of the imide proton of 7a was observed at significant downfield area (10.24 ppm) compared with 7b, which undoubtedly indicate that an intramolecular hydrogen bond between the alkoxy oxygen and the imide proton is formed in the compound 7a. On the other hand, the imide proton of 2-fluorobenzimide 7e was observed at 8.89 ppm as a doublet peak (J = 13.4 Hz). The observed spin-spin coupling indicated the formation of H-F hydrogen bond.9 In sharp contrast, no H-F interaction, that is spin-spin coupling of the imide proton, was observed in 1H NMR spectrum of difluorobenzimide 7f. 9 On the basis of these results, the conformation of 7a and 7e should be restricted by the formation of the intramolecular hydrogen bond, and therefore the bifunctional thiourea 1 could appropriately activate them by the formation of intermolecular hydrogen bond network.10 Consequently, the reaction of 7e proceeded much faster in a highly enantioselective manner.
Finally, to transform the obtained Michael adduct 8e into advanced derivative, we undertook the synthesis of piperidine-2,6-dione 10, which might be a potential synthetic intermediate for nakadomarin A (5). Treatment of benzimide 8e with benzylamine readily afforded benzylamide 9. Subsequently the reaction of 9 with t-BuOK, followed by HCl hydrolysis, provided the desired piperidine-2,6-dione 10 in 60% yield.
In summary, we have screened several α,β-unsaturated carbonyl compounds for the organocatalytic asymmetric Michael reaction with malononitrile and found that new Michael acceptor 7e possessed potential property in terms of reactivity and stereoselectivity. Moreover, we have demonstrated the transformation of 8e into piperidine-2,6-dione 10 bearing 3-furyl group at C(4) position, which corresponds to a partial structure (A or C ring) of nakadomarin A. Further study is in progress towards establishing synthetic routes for the natural product.
EXPERIMENTAL
Melting Points were taken on a YANAGIMOTO micromelting point apparatus and are uncorrected. 1H and 13C NMR spectra were recorded in CDCl3 at 500 and 126 MHz, respectively. Tetramethylsilane (TMS) was used as an internal standard. IR spectra were recorded on a JASCO FT/IR-410 spectrometer. Low and high resolution mass spectra were obtained by EI or FAB method. Optical rotations were recorded on a JASCO DIP-360 polarimeter with a path length of 1 cm; concentrations are quoted in mg (1 mL). [α]D values are measured in 10−1 deg cm2 g−1. Enantiomeric excess was determined by high performance liquid chromatography (HPLC) analysis.
Typical procedure for preparation of benzimide 7a.
A 1.58 M solution of nBuLi in hexane (8.6 mL, 13.6 mmol) was added to a solution of diethyl 2-(2-methoxybenzamido)-2-oxoethylphosphonate3b,11 (2.23 g, 6.78 mmol) in THF (15 mL) at -78 oC. The resulting solution was stirred at the same temperature for 10 min. After addition of 3-furaldehyde (0.59 mL, 6.78 mmol), the mixture was stirred at ambient temperature for 2 h. After dilution with H2O and acidification with by 1 N HCl, the resulting mixture was extracted with EtOAc, dried over Na2SO4, filtered, and concentrated in vacuo. The resulting residue was recrystallized from hexane-EtOAc to afford 7a (1.18 g, 64%) as colorless solids.
N-(E)-(3-(Furan-3-yl)acryloyl)-2-methoxybenzamide (7a): Colorless solids, mp 119-120 oC (hexane- EtOAc); 1H NMR δ 10.24 (s, 1H), 8.19 (dd, J = 7.3, 1.8 Hz, 1H), 7.81 (d, J = 15.5 Hz, 1H), 7.71 (s, 1H), 7.58 (d, J = 15.5 Hz, 1H), 7.55 (td, J = 7.3, 1.8 Hz, 1H), 7.45 (s, 1H), 7.13 (t, J = 7.3 Hz, 1H), 7.04 (d, J = 7.3 Hz, 1H), 6.74 (d, J = 1.2 Hz, 1H), 4.04 (s, 3H); 13C NMR δ 167.6, 164.0, 157.8, 145.2, 144.4, 135.9, 134.7, 132.8, 123.3, 121.7, 120.5, 120.2, 111.7, 107.8, 56.2; IR (KBr) ν 3343, 1697 cm−1; FABMS m/z 272 (M-H+, 100); Anal. Calcd for C15H13NO4: C, 66.41; H, 4.83; N, 5.16; Found: C, 66.13; H, 4.77; N, 5.16.
N-(E)-(3-(Furan-3-yl)acryloyl)benzamide (7b): Colorless solids, mp 142-143 oC (hexane-EtOAc); 1H NMR δ 9.10 (s, 1H), 7.94 (d, J = 7.3 Hz, 2H), 7.82 (d, J = 15.2 Hz, 1H), 7.72 (s, 1H), 7.61 (t, J = 7.3 Hz, 1H), 7.57 (d, J = 15.2 Hz, 1H), 7.51 (t, J = 7.3 Hz, 2H), 7.45 (s, 1H), 6.73 (s, 1H); 13C NMR δ 167.9, 166.1, 145.5, 144.5, 136.7, 133.2, 133.0, 128.9, 127.9, 123.2, 119.1, 107.8; IR (KBr) ν 3255, 1699 cm−1; EIMS m/z 241 (M+, 5), 105 (100); Anal. Calcd for C14H11NO3: C, 69.70; H, 4.60; N, 5.81; Found: C, 69.78; H, 4.84; N, 5.88.
Typical procedure for preparation of α,β-unsaturated ketone 7c.
A mixture of 3-furaldehyde (45 μL, 0.667 mmol) and BzCH=PPh3 (300 mg, 0.789 mmol) in toluene (4.0
mL) was stirred at 70 oC for 17 h. Then, the resulting mixture was concentrated in vacuo, purified with silica gel column chromatography (hexane-EtOAc = 6 : 1) to afford 7c (78.7 mg, 76%).
(E)-3-(Furan-3-yl)-1-phenylprop-2-en-1-one (7c): Yellow solids, mp 77-78 oC (hexane-EtOAc); 1H NMR δ 7.99 (td, J = 6.9, 1.1 Hz, 2H), 7.74 (s, 1H), 7.72 (d, J = 15.0 Hz, 1H), 7.58 (tt, J = 6.9, 1.1 Hz, 1H), 7.51 (dd, J = 6.3, 1.1 Hz, 1H), 7.48 (dd, J = 3.5, 1.7 Hz, 2H), 7.25 (d, J = 15.0 Hz, 1H), 6.71 (d, J = 1.7 Hz, 1H); 13C NMR δ 190.4, 145.4, 144.5, 138.1, 134.8, 132.7, 128.6, 123.2, 122.0, 107.4; IR (KBr) ν 3144, 1661 cm−1; FABMS m/z 199 (M-H+, 100); Anal. Calcd for C13H10O2: C, 78.77; H, 5.09; Found: C, 78.96; H, 5.18.
(E)-3-(Furan-3-yl)-1-(1-methyl-1H-imidazol-2-yl)prop-2-en-1-one (7d): White solids, mp 103-104 oC (hexane-EtOAc); 1H NMR δ 7.77 (d, J = 16.0 Hz, 1H), 7.75 (d, J = 2.3 Hz, 1H), 7.73 (d, J = 16.0 Hz, 1H), 7.45 (s, 1H), 7.21 (s, 1H), 7.07 (s, 1H), 6.77 (d, J = 1.6 Hz, 1H), 4.09 (s, 3H); 13C NMR δ 180.4, 145.5, 144.4, 143.9, 133.4, 129.2, 127.1, 123.4, 122.7, 107.8, 36.3; IR (KBr) ν 3128, 1660 cm−1; FABMS m/z 203 (M-H+, 100); Anal. Calcd for C11H10N2O2: C, 65.34; H, 4.98; N, 13.85; Found: C, 65.10; H, 4.92; N, 13.80.
N-(E)-(3-(Furan-3-yl)acryloyl)-2-fluorobenzamide (7e): Colorless solids, mp 113-114 oC (hexane- EtOAc); 1H NMR δ 8.89 (d, JC-F = 13.4 Hz, 1H), 8.09 (td, J = 8.0, 1.7 Hz, 1H), 7.83 (d, J = 15.4 Hz, 1H), 7.73 (s, 1H), 7.63-7.56 (m, 1H), 7.46 (d, J = 15.4 Hz, 1H), 7.46 (s, 1H), 7.34 (t, J = 8.0 Hz, 1H), 7.21 (dd, J = 12.2, 8.9 Hz, 1H), 6.73 (d, J = 1.2 Hz, 1H); 13C NMR δ 166.8, 162.1, 161.5, 159.5, 144.2, 136.8, 135.0, 132.2, 125.2, 123.1, 120.4, 119.3, 116.5, 107.7; IR (KBr) ν 3123, 1678 cm−1; FABMS m/z 262 (M-H+, 100); Anal. Calcd for C14H10FNO3: C, 64.86; H, 3.89; N, 5.40; Found: C, 64.91; H, 3.90; N, 5.35.
N-(E)-(3-(Furan-3-yl)acryloyl)-2,6-difluorobenzamide (7f): Colorless solids, mp 152-153 oC (hexane- EtOAc); 1H NMR δ 8.35 (s, 1H), 7.80 (d, J = 15.5 Hz, 1H), 7.72 (s, 1H), 7.50-7.44 (m, 2H), 7.19 (d, J = 15.5 Hz, 1H), 7.01 (dd, J = 8.6, 8.1 Hz, 2H), 6.70 (d, J = 1.7 Hz, 1H); 13C NMR δ 165.6, 160.9, 159.9, 145.7, 144.6, 137.3, 133.1, 133.0, 122.9, 118.6, 112.2, 107.7; IR (KBr) ν 1682 cm−1; FABMS m/z 278 (M-H+, 100); Anal. Calcd for C14H9F2NO3: C, 60.66; H, 3.27; N, 5.05; Found: C, 60.50; H, 3.25; N, 4.95.
General procedure for the catalytic enantioselective Michael reaction.
A mixture of 7, malononitrile (2 equiv.) and thiourea 1 (10 mol%) in toluene (0.1 M) was stirred at ambient temperature. After concentration in vacuo, the reaction mixture was purified with silica gel column chromatography (hexane-EtOAc = 4 : 1) to afford 8.
(R)-N-(4,4-Dicyano-3-(furan-3-yl)butanoyl)-2-methoxybenzamide (8a): colorless solids, mp 141-145 oC (hexane-EtOAc); [α]23D −8.5 (c 1.2, CHCl3, 92% ee); 1H NMR δ 10.42 (s, 1H), 8.15 (dd, J = 7.8, 1.8 Hz, 1H), 7.60 (s, 1H), 7.58 (td, J = 7.8, 1.8 Hz, 1H), 7.47 (t, J = 1.5 Hz, 1H), 7.13 (t, J = 7.8 Hz, 1H), 7.04 (d, J = 7.8 Hz, 1H), 6.60 (s, 1H), 4.63 (d, J = 4.9 Hz, 1H), 4.03 (s, 3H), 3.87 (ddd, J = 8.5, 5.5, 4.9 Hz, 1H), 3.62 (dd, J = 18.6, 5.5 Hz, 1H), 3.57 (dd, J = 18.6, 8.5 Hz, 1H); 13C NMR δ 172.9, 164.0, 157.8, 144.0, 140.8, 135.3, 132.9, 121.9, 121.1, 119.4, 112.2, 111.8, 111.6, 109.4, 56.2, 40.1, 33.4, 28.5; IR (KBr) ν 3331, 1748, 1679 cm−1; FABMS m/z 338 (M-H+, 97), 135 (100); Anal. Calcd for C18H15N3O4: C, 64.09; H, 4.48; N, 12.46; Found: C, 64.10; H, 4.43; N, 12.48; HPLC analysis (DAICELL CIRALPAK AS-H, Hexane:2-Propanol = 70:30, flow rate = 0.5 mL/min, 254 nm) retension time; major: 59.7 min and minor: 73.9 min.
(R)-N-(4,4-Dicyano-3-(furan-3-yl)butanoyl)benzamide (8b): colorless solids, mp 138-139 oC (hexane- EtOAc); [α]23D -8.9 (c 2.4, CHCl3, 90% ee); 1H NMR δ 8.62 (s, 1H), 7.84 (dd, J = 8.3, 1.0 Hz, 2H), 7.66 (t, J = 8.3 Hz, 1H), 7.61 (s, 1H), 7.54 (t, J = 8.3 Hz, 2H), 7.49 (t, J = 1.5 Hz, 1H), 6.59 (s, 1H), 4.50 (d, J = 4.9 Hz, 1H), 3.88 (ddd, J = 8.9, 5.2, 4.9 Hz, 1H), 3.67 (dd, J = 18.9, 5.2 Hz, 1H), 3.61 (dd, J = 18.9, 4.9 Hz, 1H); 13C NMR δ 173.2, 165.7, 144.2, 140.9, 133.9, 131.9, 129.2, 127.8, 121.0, 112.0, 111.5, 109.3, 39.5, 33.4, 28.6; IR (KBr) ν 3244, 1724, 1673 cm−1; FABMS m/z 308 (M-H+, 100); Anal. Calcd for C17H13N3O3: C, 66.44; H, 4.26; N, 13.67; Found: C, 66.36; H, 4.49; N, 13.54; HPLC analysis (DAICELL CHIRALPAK AD-H, Hexane:2-Propanol = 50:50, flow rate = 0.5 mL/min, 254 nm) retension time; major: 23.8 min and minor: 17.2 min.
(R)-2-(1-(Furan-3-yl)-3-oxo-3-phenylpropyl)malononitrile (8c): colorless oil; [α]32D -4.4 (c 1.0, CHCl3, 70% ee); 1H NMR δ 7.90 (d, J = 7.5 Hz, 2H), 7.57 (t, J = 7.5 Hz, 1H), 7.52 (s, 1H), 7.43 (dd, J = 14.3, 7.5 Hz, 3H), 6.51 (s, 1H), 4.55 (d, J = 4.3 Hz, 1H), 3.88 (dt, J = 8.0, 5.2 Hz, 1H), 3.49 (dd, J = 7.7, 5.2 Hz, 2H); 13C NMR δ 196.6, 144.2, 140.7, 135.7, 134.3, 129.0, 128.1, 121.3, 112.0, 111.7, 109.2, 40.1, 33.3, 28.5; IR (KBr) ν 3749, 2360, 1683 cm−1; FABMS m/z 265 (M-H+, 22), 73 (100); HRMS (FAB+): Calcd for C16H14N2O2 (M-H+) 265.0977, Found: 265.0930; HPLC analysis (DAICELL CHIRALCEL OD-H, Hexane:2-Propanol = 90:10, flow rate = 1.0 mL/min, 254 nm) retension time; major: 42.3 min and minor: 55.5 min.
(R)-2-(1-(Furan-3-yl)-3-(1-methyl-1H-imidazol-2-yl)-3-oxopropyl)malononitrile (8d): colorless oil; [α]30D -7.5 (c 1.0, CHCl3, 64% ee); 1H NMR δ 7.57 (s, 1H), 7.45 (s, 1H), 7.17 (s, 1H), 7.08 (s, 1H), 6.56 (s, 1H), 4.47 (d, J = 4.6 Hz, 1H), 3.99 (s, 3H), 3.91 (dt, J = 8.0, 6.3 Hz, 1H), 3.85 (dd, J = 17.8, 6.3 Hz, 1H), 3.64 (dd, J = 17.8, 8.0 Hz, 1H); 13C NMR δ 188.5, 144.0, 142.1, 140.8, 129.9, 127.9, 121.2, 112.0, 111.5, 109.3, 40.4, 36.1, 33.5, 28.9; IR (KBr) ν 3749, 2361, 1676 cm−1; FABMS m/z 269 (M-H+, 100); HRMS (FAB+): Calcd for C14H13N4O2 (M-H+) 269.1039, Found: 269.1039; HPLC analysis (DAICELL CHIRALCEL OD-H, Hexane:2-Propanol = 70:30, flow rate = 0.5 mL/min, 254 nm) retension time; major: 19.6 min and minor: 25.9 min.
(R)-N-(4,4-Dicyano-3-(furan-3-yl)butanoyl)-2-fluorobenzamide (8e): colorless solids, mp 105-106 oC (hexane-EtOAc); [α]23D −7.5 (c 1.0, CHCl3, 95% ee); 1H NMR δ 9,09 (d, J = 13.8 Hz, 1H), 8.06 (t, J = 7.5 Hz, 1H), 7.62 (t, J = 6.9 Hz, 1H), 7.60 (s, 1H), 7.48 (s, 1H), 7.35 (t, J = 7.5 Hz, 1H), 7.21 (dd, J = 8.6, 8.0 Hz, 1H), 6.58 (s, 1H), 4.58 (d, J = 5.2 Hz, 1H), 3.87 (ddd, J = 8.6, 5.2, 5.2 Hz, 1H), 3.63 (dd, J = 15.5, 5.2 Hz, 1H), 3.58 (dd, J = 15.5, 8.6 Hz, 1H); 13C NMR δ 172.3, 162.0, 161.6, 159.6, 144.1, 140.8, 135.8, 132.4, 125.5, 120.9, 119.2, 116.6, 112.0, 111.5, 109.3, 40.1, 33.4, 28.6; IR (KBr) ν 2361, 1697 cm−1; FABMS m/z 326 (M-H+, 100); HRMS (FAB+): Calcd for C17H13FN3O3 (M-H+) 326.0941, Found: 326.1003; HPLC analysis (DAICELL CHIRALCEL OD-H, Hexane:2-Propanol = 80:20, flow rate = 0.5 mL/min, 254 nm) retension time; major: 71.8 min and minor: 88.7 min.
(R)-N-(4,4-Dicyano-3-(furan-3-yl)butanoyl)-2,6-difluorobenzamide (8f): colorless amorphous; [α]31D −4.4 (c 1.4, CHCl3,63% ee); 1H NMR δ 8.61 (s, 1H), 7.59 (s, 1H), 7.53 (td, J = 8.5, 2.3 Hz, 2H), 7.49 (t, J = 1.8 Hz, 1H), 7.05 (t, J = 8.5 Hz, 2H), 4,54 (d, J = 4.6 z 1H), 3.84 (ddd, J = 8.6, 5.2, 5.2 Hz, 1H), 3.58 (dd, J = 18.9, 5.2 Hz, 1H), 3.53 (dd, J = 18.9, 8.6 Hz, 1H); 13C NMR δ 171.7, 161.1, 159.3, 159.1, 144.2, 140.9, 134.0, 120.7, 112.7, 112.5, 111.9, 111.4, 109.2, 39.8, 33.5, 30.9, 28.6; IR (KBr) ν 3289, 2258, 1624 cm−1; FABMS m/z 344 (M-H+, 2), 45 (100); HRMS (FAB+): Calcd for C17H12F2N3O3 (M-H+) 344.0847, Found: 344.0876; HPLC analysis (DAICELL CHIRALPAK AS-H, Hexane:2-Propanol = 90:10, flow rate = 0.5 mL/min, 254 nm) retension time; major: 62.6 min and minor: 85.2 min.
(R)-N-Benzyl-4,4-dicyano-3-(furan-3-yl)butanamide (9): A mixture of 8e (252 mg, 0.775 mmol) and benzylamine (110 μL, 1.01 mmol) in toluene (4.0 mL) was stirred at rt for 21 h. After concentrated in vacuo, the reaction mixture was purified by silica gel column chromatography (hexane-EtOAc = 3 : 1) to afford 9 (107 mg, 63%) as a brownish oil: [α]32D +14.6 (c 1.1, CHCl3); 1H NMR δ 7.51 (s, 1H), 7.46 (t, J = 1.3 Hz, 1H), 7.38-7.29 (m, 3H), 7.21 (d, J = 7.0 Hz, 2H), 6.49 (s, 1H), 5.90 (brs, 1H), 4,75 (d, J = 4.9 Hz, 1H), 4.46 (dd, J = 14.7, 5.8 Hz, 1H), 4.39 (dd, J = 14.7, 5.5 Hz, 1H), 3.77 (ddd, J = 8.9, 5.2, 4.9 Hz, 1H), 2.78 (dd, J = 16.2, 8.9 Hz, 1H), 2.71 (dd, J = 16.2, 5.2 Hz, 1H); 13C NMR δ 168.8, 144.2, 140.7, 137.3, 128.9, 127.8, 121.0, 112.1, 111.7, 109.1, 43.8, 37.7, 34.4, 28.4; IR (neat) ν 3301, 1640 cm−1; FABMS m/z 294 (M-H+, 100); HRMS (FAB+): Calcd for C17H16N3O2 (M-H+) 294.1243, Found: 294.1243.
(R)-1-Benzyl-4-(furan-3-yl)-2,6-dioxopiperidine-3-carbonitrile (10): To a stirred solution of 9 (105 mg, 0.359 mmol) in THF (3.6 mL) at rt, t-BuOK (40.2 mg, 0.359 mmol) was added and stirred at 50 ℃ for 3 h. After the reaction mixture was diluted with H2O and acidified with 1N HCl, the resulting mixture was extracted with EtOAc, dried over Na2SO4, filtered, and concentrated in vacuo. Resulting crude enamine product was directly used for the next reaction. A suspension of the crude enamine in 1N HCl (1.71 mL, 1.71 mmol) was stirred at reflux for 3 h. After the reaction mixture was diluted with H2O, the resulting mixture was extracted with EtOAc, dried over Na2SO4, filtered, and concentrated in vacuo. The residue was purified by silica gel column chromatography (hexane-EtOAc = 7 : 2) to afford 10 (46.1 mg, 60% from 9) as brownish oil: [α]32D +2.6 (c 2.5, CHCl3); 1H NMR (3 : 1 mixture of diastereomers, with signals corresponding to the major indicated by) δ 7.44-7.26 (m, 7H), 6.33-6.30 (m, 1H), 5.02 (dd, J = 13.9, 2.7 Hz, 1H), 4.96 (dd, J = 13.9, 5.1 Hz, 1H), 4.71 (dd, J = 17.6, 8.8 Hz, 1H), 4.05 (dd, J = 4.4, 1.0 Hz, 1H), 3.17 (dd, J = 17.6, 4.4 Hz, 1H), 3.05 (dd, J = 5.1, 1.0 Hz, 1H), 2.84 (dd, J = 10.5, 7.6 Hz, 1H); 13C NMR δ 169.1, 168.8, 163.9, 163.8, 144.5, 144.3, 143.9, 139.8, 139.7, 135.8, 135.7, 135.6, 129.1, 129.0, 128.8, 128.7, 128.58, 128.57, 128.02, 128.00, 122.0, 120.8, 114.4, 114.0, 109.1, 108.8, 44.0, 43.9, 43.0, 42.7, 42.1, 37.6, 36.9, 36.8, 35.8, 30.3, 29.3; IR (neat) ν 3136, 2259, 1705, 1677 cm−1; FABMS m/z 295 (M-H+, 51), 73 (100); HRMS (FAB+): Calcd for C17H15N2O3 (M-H+) 295.1083, Found: 295.1039.
ACKNOWLEDGEMENTS
This work was supported in part by a Grant-in-Aid for Scientific Research (B) (Y.T.) and Scientific Research on Priority Areas: Advanced Molecular Transformations of Carbon Resources (Y.T. and K.T.), “Targeted Proteins Research Program” from Ministry of Education, Culture, Sports, Science and Technology of Japan, and JSPS KAKENHI (3316). T. I thanks the JSPS for a Fellowship.
References
1. For a review, see: M. Shibasaki and N. Yoshikawa, Chem. Rev., 2002, 102, 2187. CrossRef
2. For recent reviews, see: (a) Y. Takemoto and H. Miyabe, Chimia, 2007, 61, 269; CrossRef (b) H. Miyabe and Y. Takemoto, Bull. Chem. Soc. Jpn., 2008, 81, 785. CrossRef
3. (a) Y. Hoashi, T. Okino, and Y. Takemoto, Angew. Chem. Int. Ed., 2005, 44, 4032; CrossRef (b) T. Inokuma, Y. Hoashi, and Y. Takemoto, J. Am. Chem. Soc., 2006, 128, 9413. CrossRef
4. M. S. Taylor and E. N. Jacobsen, J. Am. Chem. Soc., 2003, 125, 11204. CrossRef
5. J. Kobayashi, D. Watanabe, N. Kawasaki, and M. Tsuda, J. Org. Chem., 1997, 62, 9236. CrossRef
6. K. L. Dechant and S. P. Clissold, Drugs, 1991, 41, 225. CrossRef
7. For the examples of organocatalytic asymmetric Michael addition of other α,β-unsaturated ketones, see: (a) C. Gu, L. Liu, Y. Sui, J. Zhao, D. Wang, and Y. Cheng, Tetrahedron: Asymmetry, 2007, 18, 455; CrossRef (b) J. Wang, H. Li, L. Zu, W. Jiang, H. Xie, W. Duan, and W. Wang, J. Am. Chem. Soc., 2006, 128, 12652; CrossRef (c) B. Vakulya, S. Varga, A. Csampai, and T. Soos, Org. Lett., 2005, 7, 1967; CrossRef (d) X. Li, L. Cun, C. Lian, L. Zhong, Y. Chen, J. Liao, J. Zhu, and J. Deng, Org. Biomol. Chem., 2008, 6, 349. CrossRef
8. For the examples of organocatalytic asymmetric Michael addition of other α,β-unsaturated ester derivatives, see: (a) B. Vakulya, S. Varga, and T. Soos, J. Org. Chem., 2008, 73, 3475; CrossRef (b) S. B. Tsogoeva, Eur. J. Org. Chem., 2007, 1701. CrossRef
9. Coupling constants (JH-F) of a hydrogen-fluorine non-bonding interaction is generally observed at a value within 9.5-15.0 Hz in 1H NMR. However, the chemical shift of the imide proton is generally observed at the similar area as that of compounds having no H-F hydrogen bond. For an example, see: X Zhao, X. Wang, X. Jiang, Y. Chen, Z. Liand, and G. Chen, J. Am. Chem. Soc., 2003, 125, 15128. CrossRef
10. Recently, a detailed simulative study of the hydrogen bond network in the transition state of α,β-unsaturated imides with malononitrile activated by thiourea is reported, see: D. Zhang, G. Wang, and R. Zhu, Tetrahedron: Asymmetry, 2008, 19, 568. CrossRef
11. N. S. Goodman and E. N. Jacobsen, Adv. Synth. Catal., 2002, 344, 9.