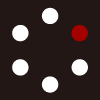
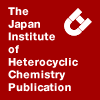
HETEROCYCLES
An International Journal for Reviews and Communications in Heterocyclic ChemistryWeb Edition ISSN: 1881-0942
Published online by The Japan Institute of Heterocyclic Chemistry
e-Journal
Full Text HTML
Received, 13th September, 2008, Accepted, 14th October, 2008, Published online, 16th October, 2008.
DOI: 10.3987/COM-08-11550
■ Effect of a Metal Salt on Thiiranation of 2’-Adamantylidene-9-benzonorbornenylidene with 4,4’-Dithiodimorpholine and Acetic Anhydride
Yoshiaki Sugihara,* Hiroki Koyama, and Juzo Nakayama*
Department of Chemistry, Graduate School of Science and Engineering, Saitama University, Shimo-okubo, Sakura-ku, Saitama 338-8570, Japan
Abstract
Using a solution of a metal salt, it was possible to decrease the quantity of Ac2O used in the thiiranation of 2’-adamantylidene-9-benzonorbornenylidene 1 with 4,4’-dithiodimorpholine 4 and Ac2O. Thiiranation in a 0.1 M Et2O solution of LiClO4 at -15 °C afforded thiiranes 2 and 3 in moderate yields without the decomposition of the thermally less stable 3.Thiiranes, as well as oxiranes and aziridines, can act as key intermediates in the syntheses of functional materials and biologically active compounds. Thus far, numerous methods, such as two-step synthesis from alkenes through oxiranes (a conventional laboratory method), for synthesizing thiiranes have been reported.1 When using two-step synthesis, undesired polymerization of the resulting thiiranes occured frequently.2 On the other hand, thiiranation of alkenes (episulfidation), i.e., direct synthesis of thiiranes from alkenes, is less common than oxiranation (epoxidation) and aziridination of alkenes.3 There have been cases in which problems, such as the polymerization and decomposition of the resulting thiiranes, limitations of the alkenes used, and difficulty in synthesizing a sulfurating reagent, were faced during the thiiranation. Development of a new method is required to overcome these problems. Recently, we indicated the possibility of using 2’-adamantylidene-9-benzonorbornenylidene 1, which acts as a model alkene for investigating thiiranation conditions.4 Until now, we investigated two novel thiiranation methods with 4,4’-oligothiodimorpholines from the results of both thiiranation of 1 to the corresponding thiirane 2 and 3, and the decomposition of 3 to 1 and 2.5,6 Thiirane 3 is thermally less stable than 2; thus, the reaction of 3 at its C−S bond tends to produce 3 together with 1. As a result, the method with 4,4’-dithiodimorpholine 4, a commercially available, inexpensive vulcanizing agent, in Ac2O at −15 °C was found to provide the best results.6 Although the thiiranation proceeded with retention of the configuration of the starting alkene, removal of excess Ac2O from the reaction mixture by either vacuum distillation at −15 °C or hydrolysis with LiOH restricted the use of alkenes. A method of decreasing the quantity of Ac2O used must be found. Here, we report the effect of a metal salt on the thiiranation of 1 with 4 and Ac2O.
LiClO4 is highly soluble in many organic solvents, such as EtOH, Et2O, and acetone, and is used as a weak Lewis acid in many organic reactions.7 A highly concentrated Et2O solution of LiClO4 is known to be a powerful reaction medium in organic synthesis.8 When this solution is used in the reaction, LiClO4 not only acts as a Lewis acid, but also increases the polarity of the solvent. Therefore, some reactions that usually proceed only under drastic reaction conditions can proceed smoothly in this solution even at rt. Considering these effects, thiiranation of 1 with 4 (1.0 molar equivalent) and Ac2O (1.0 molar equivalent) in solutions of metal salts were examined (Scheme 1), and the results are summarized in Table 1. Thiiranation of 1 with 4 and Ac2O in an Et2O solution of LiClO4 (4.1 molar equivalent, 0.1 M) at −15 °C proceeded to give 2 and 3 in 20 and 29% yields, respectively, together with 1 in 42% yield (Entry 1). When thiiranation was performed in CH2Cl2 at rt in the absence of LiClO4, a small quantity of 3 was formed together with the recovery of 1 (Entry 2).6 In the presence of 12-crown-4, which is known to capture Li+ ions efficiently,9 1 was recovered (Entry 3). Increasing the concentration of LiClO4 in the solution tended to increase the yields of the thiiranes slightly, but formed oxirane 5 (Entries 4 and 5). In the absence of Ac2O, a quantitative recovery of 1 was observed (Entry 6). These results suggest that Ac2O is activated by the action with LiClO4 as a Lewis acid, and then reacts with 4 to form a true thiiranation reagent. When the solvent was changed from Et2O into AcOEt and acetone, consumption of 1 increased, but oxirane 6 was obtained in addition to 5 (Entries 7 and 8). In these cases, AcOEt and acetone, both of which have a basic carbonyl-oxygen atom, may react with LiClO4 instead of Ac2O, and then with 4 to form the thiiranation reagent. The oxiranes 5 and 6 must be produced by the oxidation of 1 with the perchlorate ion ClO4−.10 The reaction of 1 with Ac2O and LiBF4 in CH3CN at −15 °C proceeded to give small quantities of 2 and 3 (Entries 9 and 10). Increasing the reaction temperature to rt resulted in the formation of 2 and 3 in moderate to good yields (Entries 11−14). Using THF and AcOEt as solvent showed the progress of thiiranation, and production of 2 was greater than that of 3 (Entries 15 and 16). When LiOTf was used, the ratio of 2 to 3 decreased significantly, compared to using LiClO4 and LiBF4 (Entries 17 and 18). Mg(OTf)2 seems to be more efficient for activating Ac2O than LiOTf (Entries 19 and 20). From the results as shown in entries 1, 10, 18, and 20, the efficiency of the reaction medium in the thiiranation of 1 seemed to increase in the order of LiBF4 in CH3CN < LiOTf in CH2Cl2 < LiClO4 in Et2O < Mg(OTf)2 in CH2Cl2.
Reactions of 3 with 4 (1.0 molar equivalent) and Ac2O (1.0 molar equivalent) in solutions of metal salts were examined (Scheme 2), and the results are summarized in Table 2. Decomposition of 3 did not occur in the Et2O solution of LiClO4 (4.1 molar equivalent, 0.1 M) at −15 °C and in CH2Cl2 at rt (Entries 1 and 2). When the concentration of LiClO4 in Et2O was increased to 1.0 M, isomerization to 2 and slight decomposition to 1 were observed (Entry 3). Oxirane 5, in addition to 1 and 2, was obtained by the reaction in the 2.0 M solution, but the recovery of 3 increased in comparison with that in the 1.0 M solution (Entry 4). Changing Et2O into AcOEt or acetone decreased both the consumption of 3 and formation of 2 (Entries 5 and 6). The reaction in the Et2O solution of LiBF4 (4.1 molar equivalent, 0.1 M) at −15 °C resulted in the quantitative recovery of 3, whereas that at rt gave 1 and 2 (Entries 7 and 8). The decompositions of 3 in both the LiClO4 and LiBF4 solutions tended to produce 2 rather than 1. This tendency is similar to that of the reaction of 3 with 4 (1.0 molar equivalent) and acid anhydride (1.0 molar equivalent) in CH2Cl2.6 On the other hand, an approximately 1:1 to 1:2 mixture of 1 and 2 was obtained in the decompositions in metal-triflate solutions (Entries 9−11). From the results shown in entries 1, 7, 9, and 10, the order of ability as the reaction medium in the decomposition of 3 seemed to be LiOTf in CH2Cl2 ≈ Mg(OTf)2 in CH2Cl2 < LiBF4 in CH3CN ≈ LiClO4 in Et2O. Therefore, thiiranation in the 0.1 M Et2O solution of LiClO4 at −15 °C produced the best results, because the thiiranation gave 2 and 3 in moderate yields without the decomposition of the thermally less stable 3.
A possible mechanism of the thiiranation of 1 and the decomposition of 3 is as follows. A metal salt 7 is solvated by coordination to the basic atom in the solvent to form 8. The M+ ion from 7 or 8 coordinates the carbonyl-oxygen atom in Ac2O to give ammonium salt 10, which then reacts with 4 to form sulfonium salt 11. The salts 10 and 11 must act as a thiiranation reagent. Reaction of 13 with 10 or 11 gives thiiranium salts 14 and 15, which then extrude 16 to give 17 and 18, respectively. The decomposition of 3 to 2 proceeds through the thiiranium salts 14 and 15 and carbenium salts 19 and 20, and that to 1 is a reverse process of thiiranation. The processes among 13−15 and 17−20 are reversible, and therefore, the ratio of 1, 2, and 3 may be determined by both the Lewis acidity of the metal salt and the polarity of the concentrated solution of the metal salt.
In summary, we studied the effect of a metal salt on the thiiranation of 1 with 4 and Ac2O. As a result, the 0.1 M Et2O solution of LiClO4 at −15 °C was used as a reaction medium for successful thiiranation of 1. Further work is in progress in applying this thiiranation to other alkenes.
ACKNOWLEDGEMENTS
This work was supported by Grants-in-Aid for Scientific Research from Japan Society for the Promotion of Science.
References
1. D. C. Dittmer, ‘Comprehensive Heterocyclic Chemistry,’ Vol. 7, ed. by E. D. Lwowski, Pergamon, Oxford, 1984, pp. 131-184; H. Hart, ‘Comprehensive Heterocyclic Chemistry,’ Vol. 7, ed. by E. D. Lwowski, Pergamon, Oxford, 1984, pp. 185-193; W. Ando, N. Choi, and N. Tokitoh, ‘Comprehensive Heterocyclic Chemistry II,’ Vol. 1A, ed. by A. R. Katritzky, C. W. Rees, and E. F. V. Scriven, Pergamon, Oxford, 1996, pp. 173-240; S. R. Harring and T. Livinghouse, ‘Comprehensive Heterocyclic Chemistry II,’ Vol. 1A, ed. by A. R. Katritzky, C. W. Rees, and E. F. V. Scriven, Pergamon, Oxford, 1996, pp. 241-25.
2. L. Goodman and B. R. Baker, J. Am. Chem. Soc., 1959, 81, 4924; CrossRef E. E. van Tamelen, J. Am. Chem. Soc., 1951, 73, 3444. CrossRef
3. W. Adam and R. M. Bargon, Chem. Rev., 2004, 104, 251 and references cited therein. CrossRef
4. Y. Sugihara, A. Kobiki, and J. Nakayama, Heterocycles, 2009, 78, 103. CrossRef
5. Y. Sugihara, H. Nozumi, and J. Nakayama, Heteroatom Chem., in press.
6. Y. Sugihara, H. Koyama, Y. Yasukawa, and J. Nakayama, Chem. Lett., in press.
7. A. B. Charette, ‘Handbook of Reagents for Organic Synthesis, Acidic and Basic Reagents,’ ed. by H. J. Reich and J. H. Rigby, John Wiley & Sons, Chichester, 1999, pp. 229-231; T. Imahori, “Acid Catalysis in Modern Organic Synthesis,’ ed. by H. Yamamoto and K. Ishihara, Wiley-VCH, Weinheim, 2008, pp. 109-133.
8. A. Heydari, Tetrahedron, 2000, 58, 6777. CrossRef
9. Typical procedures for thiiranaton of 1 with 4 and Ac2O in a metal-salt solution: To a Et2O solution of a metal salt (3.0 mL) was added 1 (20.1 mg, 73 μmol) and 4 (17.2 mg, 73 μmol). After the mixture was cooled to -15 °C, Ac2O (6.8 μL, 73 μmol) was added dropwise to the mixture. After stirring for 45 h at the same temperature, the reaction was quenched by the addition of ice water. After the mixture was diluted with Et2O, the organic layer was separated, washed with H2O three times, dried over MgSO4, and evaporated under reduced pressure. The residue was chromatographed on a column of silica gel and the column was eluted with hexane to give a mixture of 1, 2, and 3, and with CH2Cl2/hexane (1:5) to give a mixture of oxiranes 5 and 6. The product ratios were estimated by 1H NMR.
10. F. A. Cotton, G. Wilkinson, C. A. Murillo, and M. Bochmann, Advanced Inorganic Chemistry, 6th ed., Wiley-Interscience, New York, 1999, p. 103.
11. 5: colorless crystals (from CH2Cl2/MeOH), mp 202.5-203.0 °C; 1H NMR (300.1 MHz) δ 1.01-1.11 (m, 2H), 1.28-1.37 (m, 2H), 1.52-1.73 (m, 9H), 1.85-1.92 (m, 1H), 1.93-2.04 (m, 2H), 2.95-3.03 (m, 2H), 7.10-7.22 (m, 4H); 13C NMR (50.3 MHz) δ 25.2, 26.8, 27.1, 34.48, 34.54, 36.5, 36.8, 42.9, 72.1, 86.8, 120.8, 126.9, 144.9; IR (KBr) 3055, 2995, 2976, 2932, 2909, 2846, 1504, 1460, 1446, 1351, 1280, 1238, 1143, 1102, 1066, 1003, 958, 938, 927, 881, 851, 836, 769, 752, 686, 641, 613 cm-1; Anal. Calcd for C21H24N2O: C, 86.25; H, 8.27. Found: C, 86.22; H, 8.35. 6: colorless crystals (from CH2Cl2/MeOH), mp 198.0-198.5 °C; 1H NMR (300.1 MHz) δ 1.29-1.37 (m, 2H), 1.67-2.06 (m, 14H), 2.11-2.21 (m, 2H), 3.05-3.12 (m, 2H), 7.09-7.17 (m, 2H), 7.18-7.26 (m, 2H); 13C NMR (50.3 MHz) δ 25.2, 27.0, 27.3, 33.6, 34.8, 36.7, 37.5, 43.7, 79.2, 79.4, 121.6, 126.1, 145.2; IR (KBr) 3035, 2994, 2946, 2913, 2846, 1446, 1140, 1106, 1011, 957, 924, 837, 757, 728, 642 cm-1; Anal. Calcd for C21H24N2O: C, 86.25; H, 8.27. Found: C, 86.01; H, 8.31.
12. F. A. Cotton, G. Wilkinson, C. A. Murillo, and M. Bochmann, Advanced Inorganic Chemistry, 6th ed., Wiley-Interscience, New York, 1999, pp. 568-569.