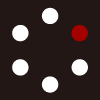
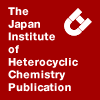
HETEROCYCLES
An International Journal for Reviews and Communications in Heterocyclic ChemistryWeb Edition ISSN: 1881-0942
Published online by The Japan Institute of Heterocyclic Chemistry
e-Journal
Full Text HTML
Received, 14th September, 2008, Accepted, 14th October, 2008, Published online, 16th October, 2008.
DOI: 10.3987/COM-08-11551
■ Synthesis and Thermal Decomposition of Thiirane 1-Imides of 2’-Adamantylidene-9-benzonorbornenylidene
Yoshiaki Sugihara,* Ayumi Kobiki, and Juzo Nakayama*
Department of Chemistry, Graduate School of Science and Engineering, Saitama University, Shimo-okubo, Sakura-ku, Saitama 338-8570, Japan
Abstract
Two isolable thiirane 1-imides 21 and 22 were synthesized successfully by imination of anti- and syn-2’-adamantylidene-9-benzonorbornenylidene sulfides 19 and 20 with Chloramine T in CH2Cl2 and MeCN at -18 °C. Thermal decompositions of 21 and 22 in solution at room temperature or in the solid state on heating gave mainly 19, alkene 23, and aziridine 24.The chemistry of thiiranes and their 1-oxides has been extensively investigated from the viewpoint of synthesis, structure, reactions, and synthetic application to useful compounds.1 Nevertheless, only a few studies have dealt with the chemistry of thiirane 1-imides.2–4 Kellogg and coworkers reported the attempted synthesis of N-tosylthiirane 1-imide 1.2 Although cis-thiirane 2 reacted with tosyl azide in the presence of Cu powder in MeOH or with Chloramine T in water, no thiirane 1-imide was observed. Hata and Watanabe reported that thiirane reacted with oxaziridine and 1,3-butadiene at room temperature to give cycloadduct 3.3 For the reaction pathway, it was speculated that N-methylthiirane 1-imide 4 forms in the initial stage, and then decomposes into the corresponding alkene and MeN=S, which is trapped by butadiene to finally give 3. Jenks and coworkers used computational methods to estimate the bond dissociation enthalpies of the S–N bond for the parent thiirane 1-imide, which are about 40 kcal mol–1 lower than those of the S–O bond for the parent thiirane 1-oxide.4 Recently, we succeeded in the first isolation of thiirane 1-imides 5–7,5 which were synthesized successfully by imination of the corresponding thiiranes 8–10 with Chloramine T (TsNNaCl) in a mixed solvent of CH2Cl2 and MeCN. Stereospecific ring-enlargement of 5 and 6 proceeded to form 11 and 12, respectively, in solution at room temperature or in the solid state on heating, whereas 7 decomposed on heating to form a mixture of 10 and 13. Decomposition of S-aminothiiranium salt 15, which was synthesized by reaction of 9 with MSSO3NH2, gave 18, whereas those of 14 and 16 afforded a mixture of the corresponding alkanes and thiiranes.6 The decomposition products seemed to strongly depend on the substituents connecting with the thiirane ring. Here, we report the synthesis and thermal decomposition of thiirane 1-imides of 2'-adamantylidene-9-benzonorbornenylidene.
Thiirane 1-imides 21 and 22 were synthesized from the corresponding thiiranes 19 and 20,7 respectively, by methods similar to the synthesis of 5−7.5 Thus, 19 and 20 reacted with 1.5 molar equivalent of Chloramine T in CH2Cl2-MeCN (3:1) at −18 °C. After a cold aqueous NaHCO3 solution was added to the reaction mixture, the organic layer was separated, dried over K2CO3, and evaporated under reduced pressure at 0 °C. The thiirane 1-imide 21 was obtained quantitatively in the imination of 19. Recrystallization of 21 from CH2Cl2 and hexane at rt resulted in the decomposition to 19, alkene 23, and aziridine 24 in 23%, 73%, and 4% yields, respectively, together with TsNH2 in 81% yield, whereas that at –18 °C afforded 21 as colorless crystals in the pure form, mp 107.0−108.0 °C (dec.). The residue for the reaction of 20 consisted of a mixture of 22, 23, and 19 in the ratio of 96:3:1. Purification of the mixture by recrystallization from CH2Cl2 and hexane at –18 °C gave 22, mp 94.0−95.0 °C (dec.), in 52% yield. The concentration of the mother liquor of this recrystallization at –18 °C yielded a mixture of 22, 23, and 19 in the ratio of 93:6:1.
The stereochemistry of 21 and 22 could be determined easily by 1H NMR.7,8 Thus, the two hydrogen atoms of the methylenes of 21, which face the benzene ring and hence are under the influence of the ring current effect,7 appeared as a multiplet in the region of δ 0.83−0.99. This multiplet is a higher field than any multiplet in the region of δ 1.58−2.35 assigned to the corresponding methylene hydrogens of 22. On the other hand, the 13C NMR spectra at –20 °C showed fifteen sp3 and ten sp2 carbon peaks for 219 and sixteen sp3 and ten sp2 carbon peaks for 22. The carbon signals of the thiirane ring of 22 (δ 79.7, 87.9) appeared at lower fields than those of 21 (δ 75.7, 83.9), 5 (δ 82.8, 83.7), 6 (δ 79.9), and 7 (δ 77.3), suggesting that steric repulsion among the substituents of 22, which causes the thiirane ring to be strained, is more severe than for those of 21 and 5−7.10 The IR spectra showed strong S–N stretching absorption at 970 cm–1 for 21 and 967 cm–1 for 22. These values were similar to the corresponding absorption for 5−7 (5: 970, 6: 957, 7: 961 cm–1).5
Both 21 and 22 decomposed in solution at room temperature, or in the solid state on heating (Table 1). Thus, allowing a CDCl3 solution of 21 to stand at room temperature for 10 days gave 19, 23, and 24 in 36%, 59%, and 5% yields, respectively, and that of 22 afforded 19, 23, and 24 in 19%, 75%, and 4% yields, respectively, together with 20 in 2% yield. When CD2Cl2 was used, each 21 and 22 decomposed to
an approximately 7:5:1 mixture of 19, 23, and 24. In the case of 22, 20 was also produced. Changing CDCl3 for CD2Cl2 increased the ratio of 19 to 23. On the other hand, heating each 21 and 22 without solvent to near their decomposition point gave 19 as a major product, and the proportion of 24 was larger compared with the decomposition in solution. The aziridine 24 seemed to be formed through 1,2-thiazetidine intermediate 25. Interestingly, the decomposition of 21 proceeded with retention of the configuration of the original stereochemistry, whereas that of 22 primarily showed an inversion of its configuration.
A possible mechanism of the thermal decompositions is as follows. In case of 21, the C−S bond that faces the benzene ring is cleft to form carbenium salt 26,11 where the neighboring group participation of the benzene ring not only assists the bond cleavage, but also stabilizes the carbocation by homoconjugation.12 The salt 26 undergoes extrusion of tosyl nitrene 28 to form carbenium salt 29, which then transforms to 19 by the C−S bond formation. Similarly, the pathway of 22, in which the C−S bond is easily broken by steric repulsion among the substituents, proceeds through 27 and then 30 to form 20. The alkene 23 is produced by several reactions such as releasing reactive species 28 and TsN=S 3113 from 21 and 22 and from 26 and 27,3 respectively, and desulfurizing 29 and 30. These reactions must be accelerated by the action with the resulting reactive species and H2O as an impurity. The extrusion of 31 from 21 and 22 follows a pathway similar to that of intermediary SO14 from thiirane 1-oxides.15 The equilibrium between 26 and 27 and that between 29 and 30 proceed through rotation about their C−C bond. The C−N bond formation in 26 gives 1,2-thiazetidine 25, which is unstable by steric repulsion among the substituents. The C−S bond cleavage with the assistance of the neighboring group participation results in the formation of carbenium salt 32, which is then desulfurized to form carbenium salt 33. The C−N bond formation of 33 affords 24. The reason why the original stereochemistry is retained in the decompositions of 21 but is inverted for 22 must be the steric repulsion among the substituents, which shifts the equilibria to 26 and 29, and the homoconjugation of the carbocation with the benzene ring in 26 and 29, causing the sp2 face opposite to the benzene ring to be more reactive than the other.
ACKNOWLEDGEMENTS
This work was supported by Grants-in-Aid for Scientific Research from Japan Society for the Promotion of Science.
References
1. I. A. Abu-Yousef and D. N. Harpp, J. Sulfur Chem., 1997, 20, 1; CrossRef D. C. Dittmer, ‘Comprehensive Heterocyclic Chemistry,’ Vol. 7, ed. by E. D. Lwowski, Pergamon, Oxford, 1984, p. 131; H. Hart, ‘Comprehensive Heterocyclic Chemistry,’ Vol. 7, ed. by E. D. Lwowski, Pergamon, Oxford, 1984, p. 18; W. Ando, N. Choi, and N. Tokitoh, ‘Comprehensive Heterocyclic Chemistry II,’ Vol. 1A, ed. by A. R. Katritzky, C. W. Rees, and E. F. V. Scriven, Pergamon, Oxford, 1996, p. 173; S. R. Harring, T. Livinghouse, ‘Comprehensive Heterocyclic Chemistry II,’ Vol. 1A, ed. by A. R. Katritzky, C. W. Rees, and E. F. V. Scriven, Pergamon, Oxford, 1996, p. 241.
2. P. Raynolds, S. Zonnebelt, S. Bakker, and R. M. Kellogg, J. Am. Chem. Soc., 1974, 96, 3146. CrossRef
3. Y. Hata and M. Watanabe, J. Org. Chem., 1980, 45, 1691. CrossRef
4. S. A. Stoffregen, R. D. McCulla, R. Wilson, S. Cercone, J. Miller, and W. S. Jenks, J. Org. Chem., 2007, 72, 823. CrossRef
5. Y. Sugihara, Y. Aoyama, H. Okada, and J. Nakayama, Chem. Lett., 2008, 37, 658. CrossRef
6. Y. Sugihara, R. Ohtsu, and J. Nakayama, Heterocycles, 2008, 75, 2415. CrossRef
7. Y. Sugihara, A. Kobiki, and J. Nakayama, Heterocycles, 2009,78, 103. CrossRef
8. 21: colorless crystals (CH2Cl2/hexane); mp > 107 °C (dec.); 1H NMR (400 MHz, -20 °C): δ 0.83-0.99 (m, 2H), 1.35-1.43 (m, 1H), 1.47-1.54 (m, 2H), 1.55-1.74 (m, 6H), 1.75-1.86 (m, 2H), 1.90 (br s, 1H), 1.96-2.04 (m, 1H), 2.22-2.36 (m, 2H), 2.41 (s, 3H), 2.47 (br s, 1H), 3.25-3.32 (m, 1H), 3.88-3.97 (m, 1H), 7.15-7.33 (m, 6H), 7.80-7.88 (m, 2H). 13C NMR (101 MHz, -20 °C): δ 21.4, 25.3, 25.8, 26.0, 26.2, 28.8, 33.6, 33.7, 35.0, 35.5, 35.6 (2C), 43.1, 44.0, 75.7, 83.9, 120.7, 120.9, 125.5, 127.0, 127.3, 129.1, 141.1, 141.3, 141.8, 144.2; IR (KBr): 3040, 2982, 2931, 2853, 1469, 1445, 1295, 1140, 1088, 970, 810, 767, 740 cm-1. Anal. Calcd for C28H31NO2S2: C, 70.40; H, 6.54; N, 2.93. Found: C, 70.29; H, 6.52; N, 2.90. 22: colorless crystals (CH2Cl2/hexane); mp > 94 °C (dec.); 1H NMR (400 MHz, -20 °C): δ 1.58-1.66 (m, 1H), 1.67-1.76 (m, 2H), 1.78-1.97 (m, 6H), 2.05-2.17 (m, 4H), 2.23-2.35 (m, 4H), 2.32 (s, 3H), 2.69 (br s, 1H), 3.32-3.36 (m, 1H), 4.08-4.14 (m, 1H), 6.94-6.97 (m, 2H), 7.14-7.24 (m, 3H), 7.24-7.26 (m, 2H), 7.28-7.34 (m, 2H). 13C NMR (101 MHz, -20 °C): δ 21.4, 25.0, 26.4, 26.6, 27.8, 28.5, 33.1, 34.1, 36.2, 37.0, 37.5, 38.1, 44.1, 45.6, 79.7, 87.9, 120.9, 121.4, 125.6, 126.3, 126.7, 128.8, 140.8, 141.2, 143.6, 145.4; IR (KBr): 3018, 2984, 2936, 2855, 1470, 1452, 1278, 1134, 1084, 967, 942, 821, 780, 769, 736 cm-1.
9. The signal at δ 35.6 is due to a degeneracy of two carbon signals.
10. E. Breimaier and W. Voelter, Carbon-13 NMR Spectroscopy, 3rd ed., VCH, Weinheim, 1987, p. 186; N. J. Jacobsen, NMR Spectroscopy Explained, Wiley-Interscience, Hoboken, 2007, p. 29. CrossRef
11. S. Oae, T. Aida, and N. Furukawa, J. Chem. Soc., Perkin Trans. 2, 1974, 1231; CrossRef T. Aida, N. Furukawa, and S. Oae, J. Chem. Soc., Perkin Trans. 2, 1976, 1432. CrossRef
12. K. Okada and T. Mukai, J. Am. Chem. Soc., 1978, 100, 6509; CrossRef L. A. Paquette, L. W. Hertel, R. Gleiter, and M. Böhm, J. Am. Chem. Soc., 1978, 100, 6510; CrossRef L. A. Paquette, L. W. Hertel, R. Gleiter, M. C. Böhm, M. A. Beno, G. G. Christoph, J. Am. Chem. Soc., 1981, 103, 7106; CrossRef Y. Sugihara, K. Noda, and J. Nakayama, Tetrahedron Lett., 2000, 40, 8907; CrossRef Y. Sugihara, K. Noda, and J. Nakayama, Tetrahedron Lett., 2000, 41, 8907; CrossRef K. Noda, Y. Sugihara, and J. Nakayama, Heteroatom Chem., 2001, 12, 625. CrossRef
13. O. Meth-Cohn and G. Vuuren, J. Chem. Soc., Perkin Trans. 1, 1986, 245. CrossRef
14. J. Nakayama, Y. Tajima, P. Xue-hua, and Y. Sugihara, J. Am. Chem. Soc., 2007, 129, 7250. CrossRef
15. G. E. Hartzell and J. N. Paige, J. Am. Chem. Soc., 1966, 88, 2616; CrossRef G. E. Hartzell and J. N. Paige, J. Org. Chem., 1967, 32, 459; CrossRef K. Kondo, M. Matsumoto, and A. Negishi, Tetrahedron Lett., 1972, 2131; CrossRef P. Chao and D. M. Lemal, J. Am. Chem. Soc., 1973, 95, 920; CrossRef D. M. Lemal and P. Chao, J. Am. Chem. Soc., 1973, 95, 922; CrossRef W. G. L. Aalbersberg and K. P. C. Vollhardt, J. Am. Chem. Soc., 1977, 99, 2792; CrossRef R. S. Glass and W. Jung, Sulfur Lett., 1994, 17, 183; I. A. Abu-Yousef and D. N. Harpp, Tetrahedron Lett., 1995, 36, 201; CrossRef I. A. Abu-Yousef and D. N. Harpp, J. Org. Chem., 1997, 62, 8366. CrossRef